
- •CONTENTS
- •Preface
- •Contributors
- •1 Introduction to Toxicology
- •1.1 Definition and Scope, Relationship to Other Sciences, and History
- •1.1.2 Relationship to Other Sciences
- •1.1.3 A Brief History of Toxicology
- •1.3 Sources of Toxic Compounds
- •1.3.1 Exposure Classes
- •1.3.2 Use Classes
- •1.4 Movement of Toxicants in the Environment
- •Suggested Reading
- •2.1 Introduction
- •2.2 Cell Culture Techniques
- •2.2.1 Suspension Cell Culture
- •2.2.2 Monolayer Cell Culture
- •2.2.3 Indicators of Toxicity in Cultured Cells
- •2.3 Molecular Techniques
- •2.3.1 Molecular Cloning
- •2.3.2 cDNA and Genomic Libraries
- •2.3.3 Northern and Southern Blot Analyses
- •2.3.4 Polymerase Chain Reaction (PCR)
- •2.3.5 Evaluation of Gene Expression, Regulation, and Function
- •2.4 Immunochemical Techniques
- •Suggested Reading
- •3.1 Introduction
- •3.2 General Policies Related to Analytical Laboratories
- •3.2.1 Standard Operating Procedures (SOPs)
- •3.2.2 QA/QC Manuals
- •3.2.3 Procedural Manuals
- •3.2.4 Analytical Methods Files
- •3.2.5 Laboratory Information Management System (LIMS)
- •3.3 Analytical Measurement System
- •3.3.1 Analytical Instrument Calibration
- •3.3.2 Quantitation Approaches and Techniques
- •3.4 Quality Assurance (QA) Procedures
- •3.5 Quality Control (QC) Procedures
- •3.6 Summary
- •Suggested Reading
- •4 Exposure Classes, Toxicants in Air, Water, Soil, Domestic and Occupational Settings
- •4.1 Air Pollutants
- •4.1.1 History
- •4.1.2 Types of Air Pollutants
- •4.1.3 Sources of Air Pollutants
- •4.1.4 Examples of Air Pollutants
- •4.1.5 Environmental Effects
- •4.2 Water and Soil Pollutants
- •4.2.1 Sources of Water and Soil Pollutants
- •4.2.2 Examples of Pollutants
- •4.3 Occupational Toxicants
- •4.3.1 Regulation of Exposure Levels
- •4.3.2 Routes of Exposure
- •4.3.3 Examples of Industrial Toxicants
- •Suggested Reading
- •5 Classes of Toxicants: Use Classes
- •5.1 Introduction
- •5.2 Metals
- •5.2.1 History
- •5.2.2 Common Toxic Mechanisms and Sites of Action
- •5.2.3 Lead
- •5.2.4 Mercury
- •5.2.5 Cadmium
- •5.2.6 Chromium
- •5.2.7 Arsenic
- •5.2.8 Treatment of Metal Poisoning
- •5.3 Agricultural Chemicals (Pesticides)
- •5.3.1 Introduction
- •5.3.3 Organochlorine Insecticides
- •5.3.4 Organophosphorus Insecticides
- •5.3.5 Carbamate Insecticides
- •5.3.6 Botanical Insecticides
- •5.3.7 Pyrethroid Insecticides
- •5.3.8 New Insecticide Classes
- •5.3.9 Herbicides
- •5.3.10 Fungicides
- •5.3.11 Rodenticides
- •5.3.12 Fumigants
- •5.3.13 Conclusions
- •5.4 Food Additives and Contaminants
- •5.5 Toxins
- •5.5.1 History
- •5.5.2 Microbial Toxins
- •5.5.3 Mycotoxins
- •5.5.4 Algal Toxins
- •5.5.5 Plant Toxins
- •5.5.6 Animal Toxins
- •5.6 Solvents
- •5.7 Therapeutic Drugs
- •5.8 Drugs of Abuse
- •5.9 Combustion Products
- •5.10 Cosmetics
- •Suggested Reading
- •6 Absorption and Distribution of Toxicants
- •6.1 Introduction
- •6.2 Cell Membranes
- •6.3 Mechanisms of Transport
- •6.3.1 Passive Diffusion
- •6.4 Physicochemical Properties Relevant to Diffusion
- •6.4.1 Ionization
- •6.5 Routes of Absorption
- •6.5.1 Extent of Absorption
- •6.5.2 Gastrointestinal Absorption
- •6.5.3 Dermal Absorption
- •6.5.4 Respiratory Penetration
- •6.6 Toxicant Distribution
- •6.6.1 Physicochemical Properties and Protein Binding
- •6.7 Toxicokinetics
- •Suggested Reading
- •7 Metabolism of Toxicants
- •7.1 Introduction
- •7.2 Phase I Reactions
- •7.2.4 Nonmicrosomal Oxidations
- •7.2.5 Cooxidation by Cyclooxygenases
- •7.2.6 Reduction Reactions
- •7.2.7 Hydrolysis
- •7.2.8 Epoxide Hydration
- •7.2.9 DDT Dehydrochlorinase
- •7.3 Phase II Reactions
- •7.3.1 Glucuronide Conjugation
- •7.3.2 Glucoside Conjugation
- •7.3.3 Sulfate Conjugation
- •7.3.4 Methyltransferases
- •7.3.7 Acylation
- •7.3.8 Phosphate Conjugation
- •Suggested Reading
- •8 Reactive Metabolites
- •8.1 Introduction
- •8.2 Activation Enzymes
- •8.3 Nature and Stability of Reactive Metabolites
- •8.4 Fate of Reactive Metabolites
- •8.4.1 Binding to Cellular Macromolecules
- •8.4.2 Lipid Peroxidation
- •8.4.3 Trapping and Removal: Role of Glutathione
- •8.5 Factors Affecting Toxicity of Reactive Metabolites
- •8.5.1 Levels of Activating Enzymes
- •8.5.2 Levels of Conjugating Enzymes
- •8.5.3 Levels of Cofactors or Conjugating Chemicals
- •8.6 Examples of Activating Reactions
- •8.6.1 Parathion
- •8.6.2 Vinyl Chloride
- •8.6.3 Methanol
- •8.6.5 Carbon Tetrachloride
- •8.6.8 Acetaminophen
- •8.6.9 Cycasin
- •8.7 Future Developments
- •Suggested Reading
- •9.1 Introduction
- •9.2 Nutritional Effects
- •9.2.1 Protein
- •9.2.2 Carbohydrates
- •9.2.3 Lipids
- •9.2.4 Micronutrients
- •9.2.5 Starvation and Dehydration
- •9.2.6 Nutritional Requirements in Xenobiotic Metabolism
- •9.3 Physiological Effects
- •9.3.1 Development
- •9.3.2 Gender Differences
- •9.3.3 Hormones
- •9.3.4 Pregnancy
- •9.3.5 Disease
- •9.3.6 Diurnal Rhythms
- •9.4 Comparative and Genetic Effects
- •9.4.1 Variations Among Taxonomic Groups
- •9.4.2 Selectivity
- •9.4.3 Genetic Differences
- •9.5 Chemical Effects
- •9.5.1 Inhibition
- •9.5.2 Induction
- •9.5.3 Biphasic Effects: Inhibition and Induction
- •9.6 Environmental Effects
- •9.7 General Summary and Conclusions
- •Suggested Reading
- •10 Elimination of Toxicants
- •10.1 Introduction
- •10.2 Transport
- •10.3 Renal Elimination
- •10.4 Hepatic Elimination
- •10.4.2 Active Transporters of the Bile Canaliculus
- •10.5 Respiratory Elimination
- •10.6 Conclusion
- •Suggested Reading
- •11 Acute Toxicity
- •11.1 Introduction
- •11.2 Acute Exposure and Effect
- •11.3 Dose-response Relationships
- •11.4 Nonconventional Dose-response Relationships
- •11.5 Mechanisms of Acute Toxicity
- •11.5.1 Narcosis
- •11.5.2 Acetylcholinesterase Inhibition
- •11.5.3 Ion Channel Modulators
- •11.5.4 Inhibitors of Cellular Respiration
- •Suggested Reading
- •12 Chemical Carcinogenesis
- •12.1 General Aspects of Cancer
- •12.2 Human Cancer
- •12.2.1 Causes, Incidence, and Mortality Rates of Human Cancer
- •12.2.2 Known Human Carcinogens
- •12.3 Classes of Agents Associated with Carcinogenesis
- •12.3.2 Epigenetic Agents
- •12.4 General Aspects of Chemical Carcinogenesis
- •12.5 Initiation-Promotion Model for Chemical Carcinogenesis
- •12.6 Metabolic Activation of Chemical Carcinogens and DNA Adduct Formation
- •12.7 Oncogenes
- •12.8 Tumor Suppressor Genes
- •12.8.1 Inactivation of Tumor Suppressor Genes
- •12.8.2 p53 Tumor Suppressor Gene
- •12.9 General Aspects of Mutagenicity
- •12.10 Usefulness and Limitations of Mutagenicity Assays for the Identification of Carcinogens
- •Suggested Reading
- •13 Teratogenesis
- •13.1 Introduction
- •13.2 Principles of Teratology
- •13.3 Mammalian Embryology Overview
- •13.4 Critical Periods
- •13.5 Historical Teratogens
- •13.5.1 Thalidomide
- •13.5.2 Accutane (Isotetrinoin)
- •13.5.3 Diethylstilbestrol (DES)
- •13.5.4 Alcohol
- •13.6 Testing Protocols
- •13.6.1 FDA Guidelines for Reproduction Studies for Safety Evaluation of Drugs for Human Use
- •13.6.3 Alternative Test Methods
- •13.7 Conclusions
- •Suggested Reading
- •14 Hepatotoxicity
- •14.1 Introduction
- •14.1.1 Liver Structure
- •14.1.2 Liver Function
- •14.2 Susceptibility of the Liver
- •14.3 Types of Liver Injury
- •14.3.1 Fatty Liver
- •14.3.2 Necrosis
- •14.3.3 Apoptosis
- •14.3.4 Cholestasis
- •14.3.5 Cirrhosis
- •14.3.6 Hepatitis
- •14.3.7 Oxidative Stress
- •14.3.8 Carcinogenesis
- •14.4 Mechanisms of Hepatotoxicity
- •14.5 Examples of Hepatotoxicants
- •14.5.1 Carbon Tetrachloride
- •14.5.2 Ethanol
- •14.5.3 Bromobenzene
- •14.5.4 Acetaminophen
- •14.6 Metabolic Activation of Hepatotoxicants
- •Suggested Reading
- •15 Nephrotoxicity
- •15.1 Introduction
- •15.1.1 Structure of the Renal System
- •15.1.2 Function of the Renal System
- •15.2 Susceptibility of the Renal System
- •15.3 Examples of Nephrotoxicants
- •15.3.1 Metals
- •15.3.2 Aminoglycosides
- •15.3.3 Amphotericin B
- •15.3.4 Chloroform
- •15.3.5 Hexachlorobutadiene
- •Suggested Reading
- •16 Toxicology of the Nervous System
- •16.1 Introduction
- •16.2 The Nervous system
- •16.2.1 The Neuron
- •16.2.2 Neurotransmitters and their Receptors
- •16.2.3 Glial Cells
- •16.3 Toxicant Effects on the Nervous System
- •16.3.1 Structural Effects of Toxicants on Neurons
- •16.3.2 Effects of Toxicants on Other Cells
- •16.4 Neurotoxicity Testing
- •16.4.1 In vivo Tests of Human Exposure
- •16.4.2 In vivo Tests of Animal Exposure
- •16.4.3 In vitro Neurochemical and Histopathological End Points
- •16.5 Summary
- •Suggested Reading
- •17 Endocrine System
- •17.1 Introduction
- •17.2 Endocrine System
- •17.2.1 Nuclear Receptors
- •17.3 Endocrine Disruption
- •17.3.1 Hormone Receptor Agonists
- •17.3.2 Hormone Receptor Antagonists
- •17.3.3 Organizational versus Activational Effects of Endocrine Toxicants
- •17.3.4 Inhibitors of Hormone Synthesis
- •17.3.5 Inducers of Hormone Clearance
- •17.3.6 Hormone Displacement from Binding Proteins
- •17.4 Incidents of Endocrine Toxicity
- •17.4.1 Organizational Toxicity
- •17.4.2 Activational Toxicity
- •17.4.3 Hypothyroidism
- •17.5 Conclusion
- •Suggested Reading
- •18 Respiratory Toxicity
- •18.1 Introduction
- •18.1.1 Anatomy
- •18.1.2 Cell Types
- •18.1.3 Function
- •18.2 Susceptibility of the Respiratory System
- •18.2.1 Nasal
- •18.2.2 Lung
- •18.3 Types of Toxic Response
- •18.3.1 Irritation
- •18.3.2 Cell Necrosis
- •18.3.3 Fibrosis
- •18.3.4 Emphysema
- •18.3.5 Allergic Responses
- •18.3.6 Cancer
- •18.3.7 Mediators of Toxic Responses
- •18.4 Examples of Lung Toxicants Requiring Activation
- •18.4.1 Introduction
- •18.4.2 Monocrotaline
- •18.4.3 Ipomeanol
- •18.4.4 Paraquat
- •18.5 Defense Mechanisms
- •Suggested Reading
- •19 Immunotoxicity
- •19.1 Introduction
- •19.2 The Immune System
- •19.3 Immune Suppression
- •19.4 Classification of Immune-Mediated Injury (Hypersensitivity)
- •19.5 Effects of Chemicals on Allergic Disease
- •19.5.1 Allergic Contact Dermatitis
- •19.5.2 Respiratory Allergens
- •19.5.3 Adjuvants
- •19.6 Emerging Issues: Food Allergies, Autoimmunity, and the Developing Immune System
- •Suggested Reading
- •20 Reproductive System
- •20.1 Introduction
- •20.2 Male Reproductive Physiology
- •20.3 Mechanisms and Targets of Male Reproductive Toxicants
- •20.3.1 General Mechanisms
- •20.3.2 Effects on Germ Cells
- •20.3.3 Effects on Spermatogenesis and Sperm Quality
- •20.3.4 Effects on Sexual Behavior
- •20.3.5 Effects on Endocrine Function
- •20.4 Female Reproductive Physiology
- •20.5 Mechanisms and Targets of Female Reproductive Toxicants
- •20.5.1 Tranquilizers, Narcotics, and Social Drugs
- •20.5.2 Endocrine Disruptors (EDs)
- •20.5.3 Effects on Germ Cells
- •20.5.4 Effects on the Ovaries and Uterus
- •20.5.5 Effects on Sexual Behavior
- •Suggested Reading
- •21 Toxicity Testing
- •21.1 Introduction
- •21.2 Experimental Administration of Toxicants
- •21.2.1 Introduction
- •21.2.2 Routes of Administration
- •21.3 Chemical and Physical Properties
- •21.4 Exposure and Environmental Fate
- •21.5 In vivo Tests
- •21.5.1 Acute and Subchronic Toxicity Tests
- •21.5.2 Chronic Tests
- •21.5.3 Reproductive Toxicity and Teratogenicity
- •21.5.4 Special Tests
- •21.6 In vitro and Other Short-Term Tests
- •21.6.1 Introduction
- •21.6.2 Prokaryote Mutagenicity
- •21.6.3 Eukaryote Mutagenicity
- •21.6.4 DNA Damage and Repair
- •21.6.5 Chromosome Aberrations
- •21.6.6 Mammalian Cell Transformation
- •21.6.7 General Considerations and Testing Sequences
- •21.7 Ecological Effects
- •21.7.1 Laboratory Tests
- •21.7.2 Simulated Field Tests
- •21.7.3 Field Tests
- •21.8 Risk Analysis
- •21.9 The Future of Toxicity Testing
- •Suggested Reading
- •22 Forensic and Clinical Toxicology
- •22.1 Introduction
- •22.2 Foundations of Forensic Toxicology
- •22.3 Courtroom Testimony
- •22.4.1 Documentation Practices
- •22.4.2 Considerations for Forensic Toxicological Analysis
- •22.4.3 Drug Concentrations and Distribution
- •22.5 Laboratory Analyses
- •22.5.1 Colorimetric Screening Tests
- •22.5.2 Thermal Desorption
- •22.5.6 Enzymatic Immunoassay
- •22.6 Analytical Schemes for Toxicant Detection
- •22.7 Clinical Toxicology
- •22.7.1 History Taking
- •22.7.2 Basic Operating Rules in the Treatment of Toxicosis
- •22.7.3 Approaches to Selected Toxicoses
- •Suggested Reading
- •23 Prevention of Toxicity
- •23.1 Introduction
- •23.2 Legislation and Regulation
- •23.2.1 Federal Government
- •23.2.2 State Governments
- •23.2.3 Legislation and Regulation in Other Countries
- •23.3 Prevention in Different Environments
- •23.3.1 Home
- •23.3.2 Workplace
- •23.3.3 Pollution of Air, Water, and Land
- •23.4 Education
- •Suggested Reading
- •24 Human Health Risk Assessment
- •24.1 Introduction
- •24.2 Risk Assessment Methods
- •24.2.2 Exposure Assessment
- •24.2.3 Dose Response and Risk Characterization
- •24.3 Noncancer Risk Assessment
- •24.3.1 Default Uncertainty and Modifying Factors
- •24.3.2 Derivation of Developmental Toxicant RfD
- •24.3.3 Determination of RfD and RfC of Naphthalene with the NOAEL Approach
- •24.3.4 Benchmark Dose Approach
- •24.3.5 Determination of BMD and BMDL for ETU
- •24.3.6 Quantifying Risk for Noncarcinogenic Effects: Hazard Quotient
- •24.3.7 Chemical Mixtures
- •24.4 Cancer Risk Assessment
- •24.5 PBPK Modeling
- •Suggested Reading
- •25 Analytical Methods in Toxicology
- •25.1 Introduction
- •25.2 Chemical and Physical Methods
- •25.2.1 Sampling
- •25.2.2 Experimental Studies
- •25.2.3 Forensic Studies
- •25.2.4 Sample Preparation
- •25.2.6 Spectroscopy
- •25.2.7 Other Analytical Methods
- •Suggested Reading
- •26 Basics of Environmental Toxicology
- •26.1 Introduction
- •26.2 Environmental Persistence
- •26.2.1 Abiotic Degradation
- •26.2.2 Biotic Degradation
- •26.2.3 Nondegradative Elimination Processes
- •26.3 Bioaccumulation
- •26.4 Toxicity
- •26.4.1 Acute Toxicity
- •26.4.2 Mechanisms of Acute Toxicity
- •26.4.3 Chronic Toxicity
- •26.4.5 Abiotic and Biotic Interactions
- •26.5 Conclusion
- •Suggested Reading
- •27.1 Introduction
- •27.2 Sources of Toxicants to the Environment
- •27.3 Transport Processes
- •27.3.1 Advection
- •27.3.2 Diffusion
- •27.4 Equilibrium Partitioning
- •27.5 Transformation Processes
- •27.5.1 Reversible Reactions
- •27.5.2 Irreversible Reactions
- •27.6 Environmental Fate Models
- •Suggested Reading
- •28 Environmental Risk Assessment
- •28.1 Introduction
- •28.2 Formulating the Problem
- •28.2.1 Selecting Assessment End Points
- •28.2.2 Developing Conceptual Models
- •28.2.3 Selecting Measures
- •28.3 Analyzing Exposure and Effects Information
- •28.3.1 Characterizing Exposure
- •28.3.2 Characterizing Ecological Effects
- •28.4 Characterizing Risk
- •28.4.1 Estimating Risk
- •28.4.2 Describing Risk
- •28.5 Managing Risk
- •Suggested Reading
- •29 Future Considerations for Environmental and Human Health
- •29.1 Introduction
- •29.2 Risk Management
- •29.3 Risk Assessment
- •29.4 Hazard and Exposure Assessment
- •29.5 In vivo Toxicity
- •29.6 In vitro Toxicity
- •29.7 Biochemical and Molecular Toxicology
- •29.8 Development of Selective Toxicants
- •Glossary
- •Index
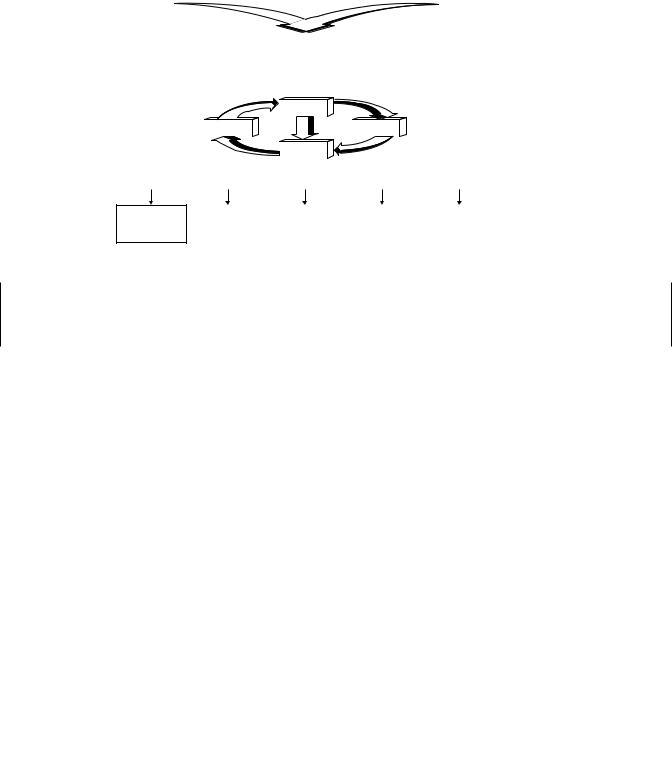
|
|
|
|
|
|
|
|
|
|
|
|
|
|
|
|
ANALYZING EXPOSURE AND EFFECTS INFORMATION |
507 |
|||||||||||||||||||||||
Activities |
|
|
|
|
|
|
|
|
|
|
|
|
|
|
|
|
|
|
|
|
|
|
|
|
|
|
|
|
|
|
|
|
|
|
|
|||||
|
|
|
|
|
|
|
|
|
|
|
|
|
|
|
|
|
|
|
|
|
|
|
|
|
Recreational/ |
|
|
|
|
Regional/ |
|
|
||||||||
|
Agricultural |
|
|
|
Industrial |
|
|
|
|
Municipal |
|
|
|
|
Commercial |
|
|
|
|
Global |
|
|
||||||||||||||||||
|
|
|
|
|
|
|
|
|
|
|
|
|
|
|
|
|
|
|
|
|
|
|
|
|
|
|
|
|
|
|
|
|
|
|
|
|
|
|
|
|
|
Water control measures |
|
|
|
Chemical waste sites |
|
|
|
Mobile sources (autos) |
|
Dredging channelizing |
|
|
|
|
|
|
|
|
|
|
|||||||||||||||||||
|
|
|
|
|
|
|
Sewage discharges |
|
|
|
Shoreline protection |
|
|
Atmospheric deposition |
|
|
|
|||||||||||||||||||||||
|
Pesticide application |
|
|
|
Waste discharges |
|
|
|
|
|
|
|
|
|
|
|
||||||||||||||||||||||||
|
|
|
|
|
|
|
Urban runoff |
|
|
|
Fish/hunting |
|
|
Fossil fuel combustion |
|
|
|
|||||||||||||||||||||||
|
Fertilizer application |
|
|
|
Construction |
|
|
|
|
|
|
|
|
|
|
|
||||||||||||||||||||||||
|
|
|
|
|
|
|
Construction |
|
|
|
Timber harvest |
|
|
Chlorofluorocarbons |
|
|
|
|||||||||||||||||||||||
|
Land alteration |
|
|
|
Spills |
|
|
|
|
|
|
|
|
|
|
|
|
|
|
|
||||||||||||||||||||
|
|
|
|
|
|
|
|
|
|
|
Waste sites |
|
|
|
Boating |
|
|
|
|
|
|
|
|
|
|
|||||||||||||||
|
|
|
|
|
|
|
|
|
|
|
|
|
|
|
|
|
|
|
|
|
|
|
|
|
|
|
|
|
|
|||||||||||
Stressors |
|
|
|
|
|
|
|
|
|
|
|
|
|
|
|
|
|
|
|
|
|
|
|
|
|
|
|
|
|
|
|
|
|
|
|
|||||
|
|
|
|
|
|
|
|
|
|
|
|
|
|
|
|
|
|
|
|
|
|
|
|
|
|
|
|
|
|
|
|
|
|
|
||||||
|
Toxicants |
|
|
|
Nutrients |
|
|
Soil Particles |
|
|
|
Noise |
|
|
|
Disease |
|
|
|
UV/B Radiation |
|
|
||||||||||||||||||
|
|
|
|
|
|
|
|
|
|
|
|
|
|
|
|
|
|
|
|
|
|
|
|
|
|
|
|
|
|
|
|
|
|
|
|
|
||||
|
|
|
|
|
|
|
|
|
|
|
|
|
|
|
|
|
|
|
|
|
|
|
|
|
|
|
|
|
|
|
|
|
|
|
|
|||||
|
|
Hydrologic |
|
|
|
|
Habitat |
|
|
|
|
Harvest |
|
|
|
Climate |
|
|
Invasive/Introduced |
|
|
|
||||||||||||||||||
|
|
Alteration |
|
|
|
|
Alteration |
|
|
|
|
Pressure |
|
|
|
Change |
|
|
|
|
Species |
|
|
|
||||||||||||||||
|
|
|
|
|
|
|
|
|
|
|
|
|
|
|
|
|
|
|
|
|
|
|
|
|
|
|
|
|
|
|
|
|
|
|
|
|
|
|||
Ecological |
|
|
|
|
|
|
|
|
|
|
|
|
|
|
|
|
|
|
|
|
|
|
|
|
|
|
|
|
|
|
|
|
|
|
|
|||||
|
|
|
|
|
|
|
|
|
|
|
|
|
|
|
|
|
|
|
|
|
|
|
|
|
|
|
|
|
|
|
|
|
|
|
||||||
Effects |
|
|
|
|
|
|
|
|
|
|
|
|
|
|
|
|
|
|
|
|
|
|
|
|
|
|
|
|
|
|
|
|
|
|
|
|||||
Models |
|
|
|
|
|
|
|
|
|
|
|
|
|
|
|
|
|
|
|
|
|
|
|
|
|
|
|
|
|
|
|
|
|
|
|
|||||
Assessment |
|
|
|
|
|
|
|
|
|
|
|
|
|
|
|
|
|
|
|
|
|
|
|
|
|
|
|
|
|
|
|
|
|
|
|
|||||
|
|
|
|
|
|
|
|
|
|
|
|
|
|
|
|
|
|
|
|
|
|
|
|
|
|
|
|
|
|
|
|
|
|
|
||||||
|
|
|
|
|
|
|
|
|
|
|
|
|
|
|
|
|
|
|
|
|
|
|
|
|
|
|
|
|
|
|
|
|
|
|
||||||
Endpoints |
|
|
|
|
|
|
|
|
|
|
|
|
|
|
|
|
|
|
|
|
|
|
|
|
|
|
|
|
|
|
|
|
|
|
|
|||||
|
|
|
|
|
|
|
|
|
|
|
|
|
|
|
|
|
|
|
|
|
|
|
|
|
|
|
|
|
|
|
|
|
|
|
||||||
|
|
|
|
|
|
|
|
|
|
|
|
|
|
|
|
|
|
|
|
|
|
|
|
|
||||||||||||||||
|
|
Water-Dependent |
|
|
Benthic |
|
|
|
|
Finfish |
|
|
|
Water/Sediment |
|
|
|
Aquatic Plant |
|
|
|
|||||||||||||||||||
|
|
Wildlife |
|
|
|
|
Invertebrates |
|
|
|
|
Community |
|
|
|
Quality Standards |
|
|
|
|
Habital |
|
|
|
||||||||||||||||
|
|
|
|
|
|
|
|
|
|
|
|
|
|
|
|
|
|
|
|
|
|
|
|
|
|
|
|
|
|
|
|
|
|
|
|
|
|
|
|
|
Measures
Populations/Health Assessment of: colonial water birds amphibians/reptiles
Pond Invertebrates: |
|
Health Assessment: |
|
Water Assessment: |
|
Plant Assessment: |
abundance |
|
gross abnormalities |
|
dissolved oxygen |
|
aquatic plant cover |
diversity |
|
histopathology |
|
turbidity |
|
light attenuation |
health indices |
|
toxicant residues |
|
primary productivity |
|
dissolved nutrients |
|
|
biomarkers |
|
toxicant residues |
|
macroalgae |
|
|
|
|
bioassays |
|
|
|
|
|
|
|
|
|
Figure 28.2 An example of a conceptual model for a watershed. Human activities, shown at the top of the diagram, result in various stressors that induce ecological effects. Assessment end points and related measures that are associated with these effects are shown at the bottom of the diagram.
An important consideration in the identification of these measures is their response sensitivity and ecosystem relevance. Response sensitivity is usually highest with measures at the lower levels of biological organization, but the ecosystem relevance is highest at the higher levels of biological organization. This dichotomy is illustrated in Figure 28.3. In general, the time required to illicit a response also increases with the level of biological organization. Note that toxicologists focus on measures at lower levels of biological organization, relying on an extrapolation of the toxicant effects on populations and communities that are initiated at the molecular/cellular level and, if this insult is not corrected for, or adapted to, then effects on physiological systems and individual organisms. For certain toxic modes of action (e.g., reproductive toxicity), this could result in effects at the population and community levels. In contrast, ecologists focus on measures at the population level or higher for obvious reasons of ecological relevance. A combination of measures often is necessary to provide reasonable sensitivity, ecosystem relevance, and causal relationships.
28.3ANALYZING EXPOSURE AND EFFECTS INFORMATION
The second phase of ecological risk assessment, the analysis phase, includes two principal activities: characterization of exposure and characterization of ecological effects (Figure 28.1).
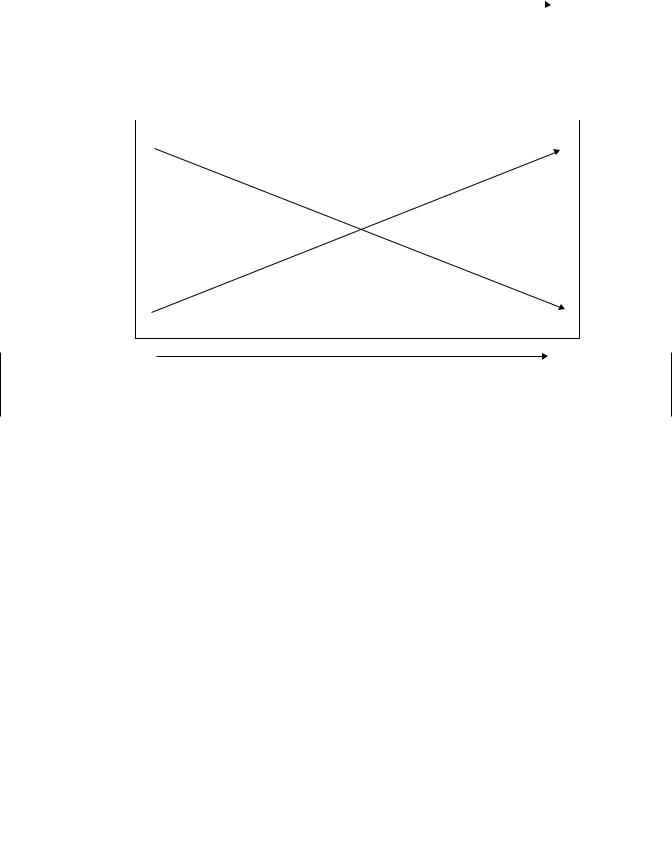
508 |
|
ENVIRONMENTAL RISK ASSESSMENT |
|
|
|
||
|
|
|
|
Level of Biological Organization |
|
|
|
|
|
|
|
|
|
||
|
|
|
Toxicologists |
Ecologists |
|||
Molecular/Cellular |
Individual Organism |
Population/Community |
Ecosystem/Landscape |
||||
|
|
metabolism |
growth/development |
abundance |
productivity |
||
|
|
gene expression |
survival |
diversity |
nutrient cycling |
||
|
|
enzyme induction |
reproduction |
succession |
energy flow |
||
|
|
immune function |
behavior |
structure/function |
food web dynamics |
||
|
|
cellular alteration |
structural alteration |
|
ecosystem interactions |
||
|
|
|
|
target dose/burden |
|
|
|
|
|
|
|
|
|
|
|
|
|
Response Sensitivity |
|
Ecosystem Relevance |
Ecosystem Relevance |
|
Response Sensitivity |
seconds |
Response Time |
decades |
Figure 28.3 The response time and sensitivity of an ecological receptor is a function of the level of biological organization. Higher levels of organization have greater ecosystem relevance. However, as the level of biological organization increases, response time increases, sensitivity decreases, and causal relationships become more uncertain. Ecological risk assessments must balance the need for sensitive, timely, and well-established responses with ecological relevance.
28.3.1Characterizing Exposure
In exposure characterization, credible and relevant data are analyzed to describe the source(s) of stressors, the distribution of stressors in the environment, and the contact or co-occurrence of stressors with ecological receptors. An exposure profile is developed that identifies receptors and exposure pathways, describes the intensity and spatial and temporal extent of exposure, describes the impact of variability and uncertainty on exposure estimates, and presents a conclusion about the likelihood that exposure will occur.
A source description identifies where the stressor originates, describes what stressors are generated, and considers other sources of the stressor. Exposure analysis may start with the source when it is known, but some analyses may begin with known exposures and attempt to link them to sources, while other analyses may start with known stressors and attempt to identify sources and quantify contact or co-occurrence. The source description includes what is known about the intensity, timing, and location of the stressor and whether other constituents emitted by the source influence transport, transformation, or bioavailability of the stressor of interest.
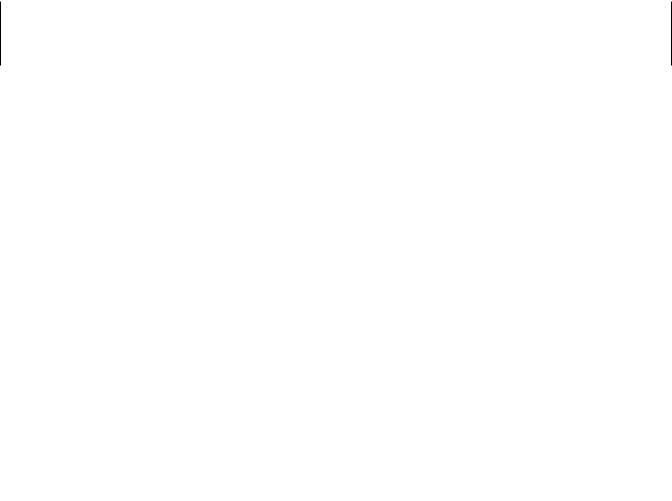
ANALYZING EXPOSURE AND EFFECTS INFORMATION |
509 |
Many stressors have natural counterparts and/or multiple sources that must be considered. For example, many chemicals occur naturally (e.g., most metals), are generally widespread due to multiple sources (e.g., polycyclic aromatic hydrocarbons), or may have significant sources outside the boundaries of the current assessment (e.g., regional atmospheric deposition of PCBs). Many physical stressors also have natural counterparts such as sedimentation from construction activities versus natural erosion. In addition human activities may change the magnitude or frequency of natural disturbance cycles such as the frequency and severity of flooding. Source characterization can be particularly important for new biological stressors (e.g., invasive species), since many of the strategies for reducing risks focus on preventing entry in the first place. Once the source is identified, the likelihood of entry may be characterized qualitatively.
Because exposure occurs where receptors co-occur with or contact stressors in the environment, characterizing the spatial and temporal distribution of a stressor is a necessary precursor to estimating exposure. The stressor’s spatial and temporal distribution in the environment is described by evaluating the pathways that stressors take from the source as well as the formation and subsequent distribution of secondary stressors. For chemical stressors, the evaluation of pathways usually follows the type of transport and fate modeling described in Chapter 27. Some physical stressors such as sedimentation also can be modeled, but other physical stressors require no modeling because they eliminate entire ecosystems or portions of them, such as when a wetland is filled, a resource is harvested, or an area is flooded.
The movement of biological stressors have been described as diffusion and/or jumpdispersal processes. Diffusion involves a gradual spread from the site of introduction and is a function primarily of reproductive rates and motility. Jump-dispersal involves erratic spreads over periods of time, usually by means of a vector. The gypsy moth and zebra mussel have spread this way; the gypsy moth via egg masses on vehicles and the zebra mussel via boat ballast water. Biological stressors can use both diffusion and jump-dispersal strategies, which makes it difficult to predict dispersal rates. An additional complication is that biological stressors are influenced by their own survival and reproduction.
The creation of secondary stressors can greatly alter risk. Secondary stressors can be formed through biotic or abiotic transformation processes and may be of greater or lesser concern than the primary stressor. Physical disturbances can generate secondary stressors, such as when the removal of riparian vegetation results in increased nutrients, sedimentation, and altered stream flow. For chemicals, the evaluation of secondary stressors usually focuses on metabolites or degradation products. In addition secondary stressors can be formed through ecosystem processes. For example, nutrient inputs into an estuary can decrease dissolved oxygen concentrations because they increase primary production and subsequent decomposition. A changeover from an aerobic to an anaerobic environment often is accompanied by the production of sulfide via sulfate-reducing bacteria. Sulfide can act as a secondary stressor to oxygen-dependent organisms, but it also can reduce exposure to metals through the precipitation of metal sulfides (see Chapter 27).
The distribution of stressors in the environment can be described using measurements, models, or a combination of the two. If stressors have already been released, direct measurements of environmental media or a combination of modeling and measurement is preferred. However, a modeling approach may be necessary if the assessment is intended to predict future scenarios or if measurements are not possible or practicable.
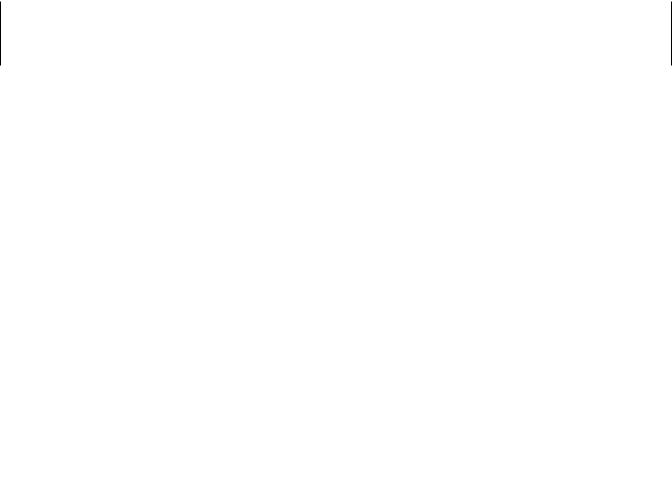
510 ENVIRONMENTAL RISK ASSESSMENT
28.3.2Characterizing Ecological Effects
In ecological effects characterization, relevant data are analyzed to evaluate stressorresponse relationships and/or to provide evidence that exposure to a stressor causes an observed response. The characterization describes the effects that are elicited by a stressor, links these effects with the assessment endpoints, and evaluates how the effects change with varying stressor levels. The conclusions of the ecological effects characterization are summarized in a stressor-response profile.
Analyzing Ecological Response. Ecological response analysis has three primary components: determining the relationship between stressor exposure and ecological effects, evaluating the plausibility that effects may occur or are occurring as a result of the exposure, and linking measurable ecological effects with the assessment end points.
Evaluating ecological risks requires an understanding of the relationships between stressor exposure and resulting ecological responses. The stressor-response relationships used in a particular assessment depend on the scope and nature of the ecological risk assessment as defined in problem formulation and reflected in the analysis plan. For example, a point estimate of an effect (e.g., an LC50) might be compared with point estimates from other stressors. The stressor-response function (e.g., shape of the curve) may be critical for determining the presence or absence of an effects threshold or for evaluating incremental risks, or stressor-response functions may be used as input for ecological effects models. If sufficient data are available, cumulative distribution functions can be constructed using multiple point estimates of effects. Process models that already incorporate empirically derived stressor-response functions also can be used. However, many stressor-response relationships are very complex, and ecological systems frequently show responses to stressors that involve abrupt shifts to new community or system types.
In simple cases the response will be one variable (e.g., mortality) and quantitative univariate analysis can be used. If the response of interest is composed of many individual variables (e.g., species abundances in an aquatic community), multivariate statistical techniques must be used. Multivariate techniques (e.g., factor and cluster analysis) have a long history of use in ecology but have not yet been extensively applied in risk assessment. Stressor-response relationships can be described using any of the dimensions of exposure (i.e., intensity, time, space). Intensity is probably the most familiar dimension and is often used for chemicals (e.g., dose, concentration). The duration of exposure also can be used for chemical stressor-response relationships; for example, median acute effects levels are always associated with a time parameter (e.g., 24 h, 48 h, 96 h). Both the time and spatial dimensions of exposure can be important for physical disturbances such as flooding. Single-point estimates and stressor-response curves can be generated for some biological stressors. For pathogens such as bacteria and fungi, inoculum levels may be related to the level of symptoms in a host or actual signs of the pathogen. For other biological stressors such as introduced species, developing simple stressor-response relationships may be inappropriate.
Causality is the relationship between cause (one or more stressors) and effect (assessment end point response to one or more stressors). Without a sound basis for linking cause and effect, uncertainty in the conclusions of an ecological risk assessment will be high. Developing causal relationships is especially important for risk assessments driven by observed adverse ecological effects such as fish kills or long-term declines
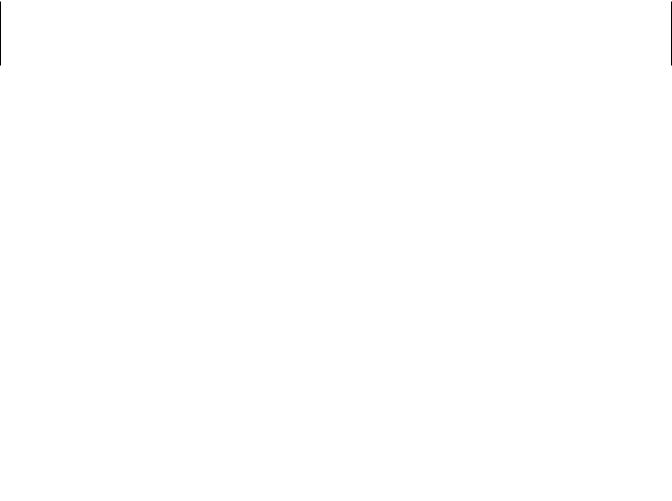
ANALYZING EXPOSURE AND EFFECTS INFORMATION |
511 |
in a population. Criteria need to be established for evaluating causality. For chemicals, ecotoxicologists have slightly modified Koch’s postulates to provide evidence of causality:
1.The injury, dysfunction, or other putative effect of the toxicant must be regularly associated with exposure to the toxicant and any contributory causal factors.
2.Indicators of exposure to the toxicant must be found in the affected organisms.
3.The toxic effects must be seen when normal organisms or communities are exposed to the toxicant under controlled conditions, and any contributory factors should be manifested in the same way during controlled exposures.
4.The same indicators of exposure and effects must be identified in the controlled exposures as in the field.
While useful as an ideal, this approach may not be practical if resources for experimentation are not available or if an adverse effect may be occurring over such a wide spatial extent that experimentation and correlation may prove difficult or yield equivocal results. In most cases extrapolation will be necessary to evaluate causality. The scope of the risk assessment also influences extrapolation through the nature of the assessment end point. Preliminary assessments that evaluate risks to general trophic levels, such as fish and birds, may extrapolate among different genera or families to obtain a range of sensitivity to the stressor. On the other hand, assessments concerned with management strategies for a particular species may employ population models.
Whatever methods are employed to link assessment end points with measures of effect, it is important to apply the methods in a manner consistent with sound ecological and toxicological principles. For example, it is inappropriate to use structure-activity relationships to predict toxicity from chemical structure unless the chemical under consideration has a similar mode of toxic action to the reference chemicals. Similarly extrapolations from upland avian species to waterfowl may be more credible if factors such as differences in food preferences, physiology, and seasonal behavior (e.g., mating and migration habits) are considered.
Finally, many extrapolation methods are limited by the availability of suitable databases. Although these databases are generally largest for chemical stressors and aquatic species, even in these cases data do not exist for all taxa or effects. Chemical effects databases for mammals, amphibians, or reptiles are extremely limited, and there is even less information on most biological and physical stressors. Extrapolations and models are only as useful as the data on which they are based and should recognize the great uncertainties associated with extrapolations that lack an adequate empirical or process-based rationale.
Developing a Stressor-Response Profile. The final activity of the ecological response analysis is developing a stressor-response profile to evaluate single species, populations, general trophic levels, communities, ecosystems, or landscapes—whatever is appropriate for the defined assessment end points. For example, if a single species is affected, effects should represent appropriate parameters such as effects on mortality, growth, and reproduction, while at the community level, effects may be summarized in terms of structure or function depending on the assessment end point. At the landscape level, there may be a suite of assessment end points, and each should be addressed separately. The stressor-response profile summarizes the nature and intensity of effect(s),
- #15.08.20134.04 Mб17Hastie T., Tibshirani R., Friedman J. - The Elements of Statistical Learning Data Mining, Inference and Prediction (2002)(en).djvu
- #
- #
- #
- #
- #
- #
- #
- #15.08.201315.44 Mб26Hudlicky M, Pavlath A.E. (eds.) - Chemistry of Organic Fluorine Compounds 2[c] A critical Review (1995)(en).djvu
- #
- #