
Micro-Nano Technology for Genomics and Proteomics BioMEMs - Ozkan
.pdfPEPTIDE ARRAYS IN PROTEOMICS AND DRUG DISCOVERY |
163 |
A planar support has to fulfill several requirements in order to be suitable for step by step solid phase peptide synthesis: these include chemical stability against a variety of solvents, reagents and reaction conditions as well as mechanical stability towards repetitive steps of solvent deposition, washing and deprotection. Moreover, surface functional groups must be accessible for chemical derivatization and the final surface properties should allow the biochemical interaction of the immobilized peptides with a binding partner during solid phase binding assays with limited non-specific interactions.
Cellulose-bound peptides paved the way in the field of peptide arrays. The SPOT technology, a very rapid, robust and easy-to-use method for preparing peptide arrays in a stepwise manner directly on cellulose or other membranes and its applications have been reviewed extensively (Wenschuh et al., 2000; [160–162, 443, 454]). Apart from several special applications these peptide arrays are mainly used for antibody epitope and paratope mapping, mapping of protein-protein interactions in general, and investigation of enzyme/substrate/inhibitor interactions (kinases, proteases, isomerases, and phosphatases). Obviously, for biochemical assays impermeable, rigid materials, such as glass, have a number of practical advantages over porous supports. Binding partners interacting with the immobilized peptides find immediate access to the ligands without diffusing into pores, thereby accelerating the binding process. Washing steps following incubation are not limited by diffusion, leading to improved reproducibility. Finally, the flatness and transparency of glass or plastic supports improve image acquisition due to the much better definition of probes compared to flexible membranes. High image definition is a critical factor for determining the final density that can be achieved on peptide microarrays. Additionally, rigidity permits incorporation into flow cells necessary for automated processing during high throughput analysis. Moreover, the interaction kinetics on peptide microarrays fixed on impermeable supports is not complicated by solvent and interacting partner diffusion into and/or out of pores (as described for DNA/DNA interactions [329]. The focus of this review will be peptide arrays composed of peptides preferably bound covalently to planar supports through one defined terminus.
7.2.1. Coherent Surfaces and Surface Modification
Polymer surface modification has been the subject of increased research in different areas such as the development of biocompatible implants [228] or biosensors, for affinity chromatography, and for immobilization of molecules in ELISA experiments.
Generally, the quality of peptide arrays is determined by surface properties such as homogeneity, roughness, hydrophobicity, density of functional groups, spacing between surface and biologically active compounds, and amenability to interaction with proteins or enzymes. Surface-sensitive analytical methods used to characterize nonporous materials such as silicon wafer, glass and titanium include goniometry (contact angle measurement), variable angle spectral ellipsometry, X-ray photoelectron spectroscopy, attenuated total reflection–Fourier transform infrared spectroscopy, secondary ion mass spectroscopy and atomic force microscopy.
In most cases amino acids (step by step synthesis) or peptides (immobilization of presynthesized peptides) cannot be coupled directly to the surface of the applied membranes or rigid materials (metal, glass or plastic). It is therefore necessary to transform such surfaces into amino functions or other appropriate reactive groups (hydroxy, mercapto, aldehyde,
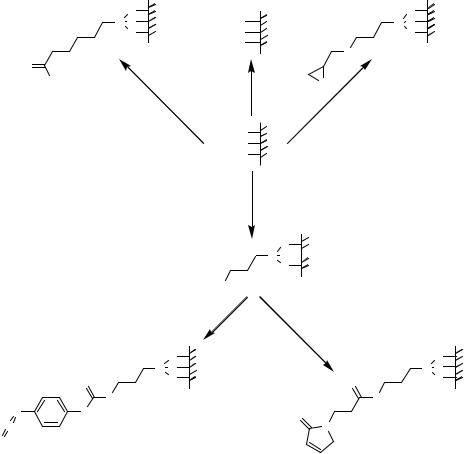
164 |
ULRICH REINEKE, JENS SCHNEIDER-MERGENER AND MIKE SCHUTKOWSKI |
maleimide, epoxy) to allow either initiation of the solid phase peptide synthesis or immobilization of pre-synthesized peptides. A further important factor is that the penultimate amino acid linkage to the surface must have a chemical stability at least equal to that of the peptide backbone. Moreover, it is desirable that the surface is optically transparent since radioactivity has largely been replaced by spectroscopic, especially fluorescent detection techniques.
Polymer surfaces can be modified by silylation, corona treatment, γ-radiation or graft copolymerization (Figures 7.1, 7.2, 7.3, 7.19). Such treatments can enhance the polymer’s reactivity and/or modify the hydrophobic/hydrophilic character of the surface. Polystyrene, for example, may be surface activated by glow discharge and graft co-polymerized with acrylic acid to provide a reactive surface for peptide immobilization. Polyurethane films can
O |
|
|
O |
Si O |
Cl |
|
Si O |
O |
Cl |
|
O |
|
Cl |
|
O |
|
|
|
|
O |
|
|
|
H |
i |
ii |
O |
|
|
||
|
|
|
|
|
|
|
iii |
|
HO |
|
|
|
HO |
|
|
|
HO |
|
|
iv
O
Si O
O
|
|
|
H2N |
|
|
|
|
v |
|
|
|
|
vi |
|
|
|
O |
|
O |
|
|
Si O |
|
Si O |
|
S |
O |
O |
O |
|
|
|
||
|
|
NH |
|
NH |
N |
NH |
|
O |
|
C |
|
|
|
|
|
|
N |
|
S O
FIGURE 7.1. Functionalization of glass surfaces. i = 6-triethoxysilyl-hexanal; ii = H2SO4/H2O2, 16h, washing with water, thionyl cloride in THF, cat. DMF [204], iii = 3-glycidyloxypropyltrimethoxysilane/toluene [553]; iv = 3-aminopropyltrimethoxysilane/toluene; v = phenyldiisothiocyanate in pyridine/dimethylformamide 1:9, 2 h [31]; vi = N-succinimidyl-3-maleimidylpropionate in acetonitrile.
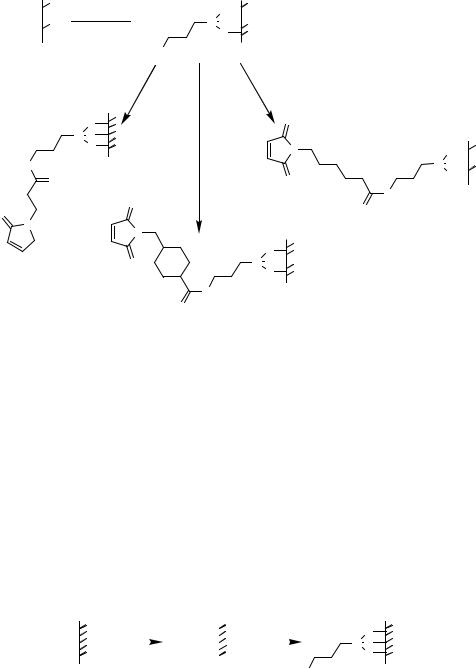
PEPTIDE ARRAYS IN PROTEOMICS AND DRUG DISCOVERY
HO
HO
HO
HN
O
O
N
O
i O Si O
O
|
H2N |
|
|
ii |
iv |
|
|
|
O |
|
O |
|
|
|
Si O |
iii |
|
O |
N |
|
|
|
|
|
|
O |
NH
O
O
N
O
O Si O
O
NH
O
165
O Si O
O
FIGURE 7.2. Functionalization of titanium surfaces [603]. i = silanization of water-vapor-plasma pretreated titanium using (3-aminopropyl)triethoxysilane in anhydrous toluene; ii = N-succinimidyl-3-maleimidylpropionate in acetonitrile, iii = N-succinimidyl trans-4-(maleimidylmethyl)cyclohexane-1-carboxylate in acetonitrile; iv = N-succinimidyl-6-maleimidylhexanoate in acetonitrile.
be modified by plasma deposition of 1,2-diaminocyclohexane followed by carbodiimidemediated grafting of carboxylated dextran onto the amino-modified polyurethane yielding surface-bound carboxylic functions [513]. Polypropylene is readily aminated by plasma discharge in the presence of anhydrous ammonia [354]. Polypropylene and polyethylene can be functionalized by vapor phase photo-grafting of methyl methacrylate, acrylic acid or methacrylic acid in the presence of sensitizers such as benzoin ethyl ester or benzophenone [13, 383, 387]. The photochemical grafting of styrene onto polypropylene using photosensitizers is described [313]. Additionally, the photo-induced graft copolymerization of polypropylene with acrylates was reported to be effective for the generation of chemically defined ultrafiltration membranes with a hydrophilic surface modification [557]. Several different acrylates were used as monomers for the functionalization
i, ii, iii |
HO |
|
|
iv |
O |
|
|||||
|
HO |
|
|
|
Si O |
|
|
|
|
||
|
HO |
|
|
|
O |
|
|
|
|
||
|
|
|
|
|
|
HS
FIGURE 7.3. Functionalization of silicon wafers [308]. i = 90% H2SO4, 10% H2O2, 125◦ C, 10 min; ii = 2% hydrofluoric acid, 1 min; iii = potassium permanganate in concentrated H2SO4, 1.5 min; iv = 2-propanol/H2O/(3- mercaptopropyl)trimethoxysilane 100:1:2.5 (v,v,v), reflux, 30 min, curing 115◦ C.
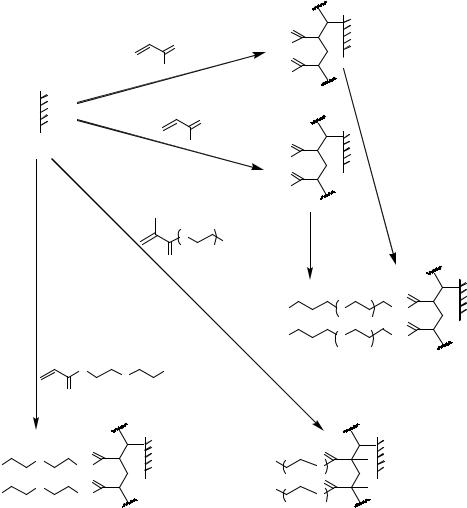
166 |
ULRICH REINEKE, JENS SCHNEIDER-MERGENER AND MIKE SCHUTKOWSKI |
||||
|
|
|
O |
|
|
|
|
O |
HO |
|
|
|
|
|
O |
|
|
|
|
OH |
HO |
|
|
|
|
|
|
|
|
|
|
i |
|
|
|
|
|
O |
|
|
|
|
|
OMe |
O |
|
|
|
|
ii |
MeO |
|
|
|
|
|
|
iv |
|
|
|
|
O |
|
|
|
|
|
|
|
|
|
|
|
MeO |
|
|
|
|
O |
|
|
|
|
|
9 OH |
iii |
|
|
|
|
O |
|
|
|
|
v |
vi |
|
|
O |
|
|
|
|
||
|
|
|
H2N |
O |
3 HN |
|
|
|
|
|
O |
|
|
|
H2N |
O |
HN |
|
|
|
|
|
3 |
|
H |
OH |
|
|
|
|
N |
|
|
|
|
|
|
O |
|
|
|
|
O |
|
|
|
|
|
O |
|
O |
|
|
|
O |
|
HO |
|
|
HO |
HN |
|
O |
9 |
|
|
O |
|
O |
|
|
HO |
O |
|
HO |
|
|
HN |
|
O 9 |
|
FIGURE 7.4. Modification of polypropylene surfaces (Wenschuh et al., 2000). i = acrylic acid, ii = acrylic acid methylester, iii = 4,7,10-trioxa-1,13-tridecanediamine, 100◦ C, 72 h, iv = PCl5 / methylene chloride / 4,7,10- trioxa-1,13-tridecanediamine, v = grafting using aryloyl-aminoethoxyethanol, vi = grafting using polyethylene glycol methacrylate.
of polypropylene membranes (Figure 7.4) or Teflon membranes [130]. Acrylic acid and acrylic acid methylester yielded carboxylic acid and methylester-modified polypropylene membranes, respectively (Figures 7.4i, 7.ii), with loadings between 7.5 and 12.5 nmol/mm2 (Table 7.1) (Wenschuh et al., 2000). The acrylic acid modified membranes were activated and amidated with 4,7,10-trioxa-1,13-tridecanediamine yielding aminofunctionalized polypropylene surfaces suitable for peptide synthesis (Figure 7.4iv). Alternatively, the methylester-modified membrane was treated directly with 4,7,10-trioxa- 1,13-tridecanediamine resulting in an amino function linked to the membrane surface by a

PEPTIDE ARRAYS IN PROTEOMICS AND DRUG DISCOVERY |
167 |
TABLE 7.1. Reported loadings of different surfaces with functional groups
|
surface |
surface loading |
|
material |
function |
amount/mm2 |
reference |
paracyclophane coated stainless steel foil |
isocyanate |
7.6 pmol |
[283] |
trioxa cellulose |
amino |
1 nmol |
[591] |
glycinester cellulose |
amino |
12 nmol |
[591] |
epoxypropylamine cellulose |
amino |
5 nmol |
[591] |
trioxa polypropylene |
amino |
5 nmol |
[591] |
acylic acid modified polypropylene |
carboxylic acid |
12.5 nmol |
[591] |
acylate modified polypropylene |
methylester |
7 nmol |
[591] |
modified polypropylene |
hydroxyl |
3 nmol |
[591] |
poly(aminoethylmethacrylamide) coated |
amino |
3–4 nmol |
[97] |
polypropylene |
|
|
|
aminopropyldiethoxymethylsilane treated glass |
amino |
6 pmol |
[388] |
aminopropylmonomethoxydimethylsilane treated |
amino |
2 pmol |
[388] |
glass |
|
|
|
aziridine treated aminosilylated glass |
amino |
68 pmol |
[388] |
glycidyloxipropylsilylated glass |
epoxide |
5.7 pmol |
[412] |
hexadecanethiol and glycidyloxipropylsilylated |
mercapto |
5.5 pmol |
[412] |
glass |
|
|
|
diamino PEG and glycidyloxipropylsilylated glass |
amino |
1.1 pmol |
[412] |
aminosilylated titanium |
amino |
10 pmol |
[603] |
aminosilylated glass |
amino |
<1 pmol |
[405] |
RGD grafted polypyrrole on titanium |
pyrrole |
30 pmol |
[100] |
polypropylene |
amino |
10 pmol |
[523] |
aminosilylated glass |
amino |
100 fmol |
[523] |
self assembled monolayer on gold surfaces |
benzoquinone |
100 fmol |
[218] |
poly((D), L-lactide grafted with polyacylic acid |
carboxyl |
3–5 nmol |
[525] |
functionalized quartz surface |
amino |
40–60 fmol |
[467] |
3-(aminopropyl)diethoxymethylsilane treated |
amino |
5.5 pmol |
[257] |
Si(100) wavers |
|
|
|
3-(aminopropyl)dimethylethoxysilane treated |
amino |
2.5 pmol |
[257] |
Si(100) wavers |
|
|
|
aziridine treated aminosilylated Si(100) wavers |
amino |
80–110 pmol |
[257] |
glycidyloxipropylsilylated glass |
epoxy |
34 fmol |
US-5474796 |
self-assembled monolayer of w-alkanethiol on gold |
mercapto |
250 fmol |
[581] |
surfaces |
|
|
|
|
|
|
|
hydrophilic spacer and a relatively stable amide bond (Figure 7.4iii). Moreover, acryloylaminoethoxyethanol or polyethylene glycol methacrylates gave hydroxy-functionalized surfaces by means of a one step grafting procedure (Figure 7.4v, 7.4vi; [591]). The quality of model peptides synthesized on these surfaces by SPOT synthesis was demonstrated to be similar or even better than that obtained with cellulose under comparable conditions [589]. In addition, it was shown that polypropylene membranes could be used to assemble peptoids, peptide nucleic acids, glycopeptides, and triazinyl peptides [590] using the SPOT technique. Further modifications (useful for solid phase peptide synthesis) of functionalized polypropylene (Figure 7.5; [97, 578]) and polypropylene coated with crosslinked polyhydroxypropylacrylate (Figure 7.6; [97]) are reported. A variety of plastic polymers can be functionalized directly by chemical treatment yielding carboxylic functions (Figure 7.7; [241]) that are easily transformed into pentafluorophenylesters. Recently, it was
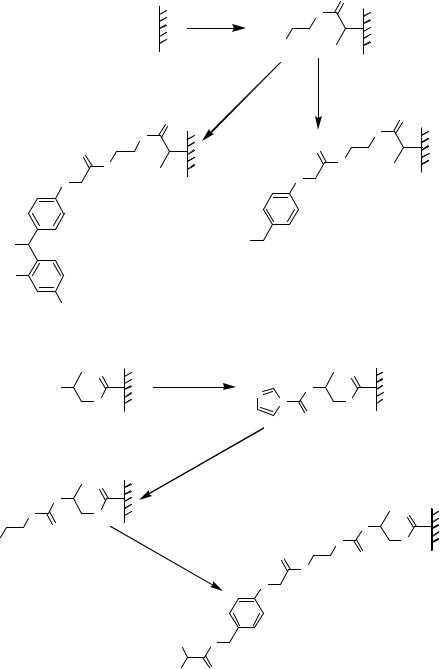
168 |
|
|
ULRICH REINEKE, JENS SCHNEIDER-MERGENER AND MIKE SCHUTKOWSKI |
||||
|
|
|
|
|
|
|
O |
|
|
|
|
|
|
|
HN |
|
|
|
|
|
|
|
i |
|
|
|
|
|
|
|
H2N |
|
|
|
|
|
|
|
ii |
|
|
|
|
|
|
O |
O |
|
|
|
|
|
|
HN |
|
|
|
|
|
|
HN |
|
|
|
|
|
|
|
|
O |
|
|
|
|
O |
|
|
|
|
|
|
|
|
|
|
NH |
|
|
|
|
|
NH |
|
|
|
|
|
|
|
|
|
O |
|
|
|
O |
|
|
|
|
|
|
|
|
|
|
|
|
|
|
H2N |
|
|
|
|
|
HO |
|
|
|
|
|
|
|
|
|
MeO |
|
|
|
|
|
|
OMe
FIGURE 7.5. Modification of polypropylene surfaces [97]. i = aminoethylmethacrylamide/ h*ν; ii = (4- hydroxymethyl)phenoxyacetic acid pentafluorophenyl ester.
|
O |
|
i |
|
O |
|
HO |
|
O |
|
|
|
|
N |
|
||
|
O |
|
|
O |
|
|
|
N |
|
||
|
|
|
|
O |
|
|
|
|
ii |
|
|
|
O |
|
|
|
|
|
O |
|
|
|
|
HN |
O |
|
|
|
O |
|
O |
|
|
|
O |
H2N |
|
iii , iv |
|
HN |
O |
|
|
O |
|
O |
|
|
|
|
|
NH
O
H2N O
RO
FIGURE 7.6. Modification of polypropylene coated with crosslinked polyhydroxypropylacrylate [97, 578]. i = carbonyldiimidazole/DMF; ii = 1,3-diaminopropane/DMF; iii = Fmoc-amino acyl-oxymethylphenoxyacetic acid 2,4-dichlorophenylester/ 4 eq pyridine/DMF; iv = 20% piperidine/DMF.
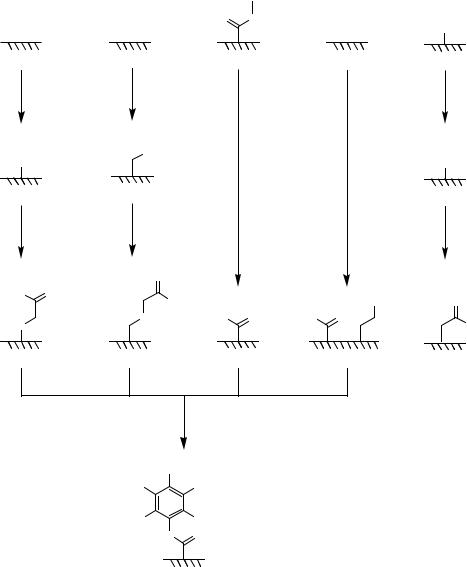
PEPTIDE ARRAYS IN PROTEOMICS AND DRUG DISCOVERY
A) PE |
B) PS |
C) PMMA |
D) PET |
O O
OH
OH
|
|
O |
|
HO |
O |
OH |
OH |
|
|
||
O |
O |
HO O |
HO O |
|
|
|
iii
F
FF
FF O O
169
E)PMPP
CH3
i
CH2Li
ii
O
OH
FIGURE 7.7. Modification of different plastic surfaces. A) PE = polyethylene; B) PS = polystyrene; C) PMMA = polymethylmethacrylate; D) PET = polyethylenterephthalate; E) PMPP = polymethylphenylphosphazene; i = 2.5 M n butyl lithium, hexane, −78◦ C, 1 h, room temperature; ii = CO2, 0.01 M HNO3 [241]; iii = pentafluorophenol, DIC.
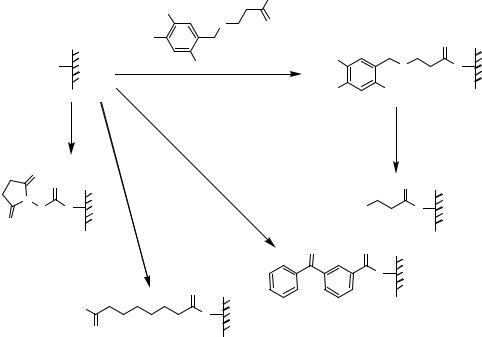
170 |
ULRICH REINEKE, JENS SCHNEIDER-MERGENER AND MIKE SCHUTKOWSKI |
OH
MeO
SO
|
MeO |
|
O |
|
|
|
|
|
MeO |
|
|
H2N |
NO2 |
S |
N |
|
i |
|
H |
|
NO2 |
|
|
|
MeO |
|
|
|
iii |
ii |
|
|
|
|
|
|
iv |
|
|
O |
|
|
|
O |
|
O |
|
N |
v |
HS |
N |
O |
N |
||
O |
H |
|
H |
|
|
|
|
|
O |
O |
|
|
|
N |
|
|
O |
H |
|
|
|
|
MeO
N
H
O
FIGURE 7.8. Functionalization of aminosilylated glass surfaces. i = (2-nitro-4,5-dimethoxy-benzyl)-3- mercaptopropionic acid/1-(3-dimethylaminopropyl)-3-ethylcarbodiimide hydrochloride; ii = h*ν; iii = disuccinimidylcarbonate, diisopropylamine, acetonitrile, 4 h [31]; iv = N-succinimidyl-3-benzoylbenzoate in DMF [480]; v = dimethylsuberimidate in saturated NaHCO3, 1 h [31].
demonstrated that the polycarbonate surface of a normal CD-ROM could be converted into a chlorophosphate moiety that allows immobilization of biomolecules [282].
Glass, silicon dioxide, silica surfaces and transition metal oxides are readily functionalized by silanization with reactive silanes containing alkyl chains terminated with functional groups (Figure 7.1). Coatings with aminopropyltriethoxysilane yielding primary amino functions that can be easily modified are widely used (Figures 7.1v, 7.1vi, 7.8). Side reactions such as oligomerization and the formation of multiple layers normally prevent the formation of ordered alkyl silane monolayers [527] and result in hydrophobic silanized surfaces displaying strong unspecific adsorption [410]. This situation can be circumvented by either using different aminosilanes, such as aminopropyldiethoxymonomethylsilane or aminopropylmonoethoxydimethylsilane [388], or attaching ultra-thin layers of bifunctional polyethylene glycols to the surfaces [375, 411, 412, 608].
Additionally, multistep functionalization of pretreated titan surfaces has been described using aminopropyltriethoxysilane followed by reaction with N-succinimidyl- 3-maleimidylpropionate, N-succinimidyl-6-maleimidylhexanoate or N-succinimidyl trans-4-(maleimidylmethyl)cyclohexane-1-carboxylate in acetonitrile (Figure 7.2; [603]). The resulting maleinimide-modified surface enables the directed immobilization of peptides containing cysteine by Michael addition. Moreover, the published modification
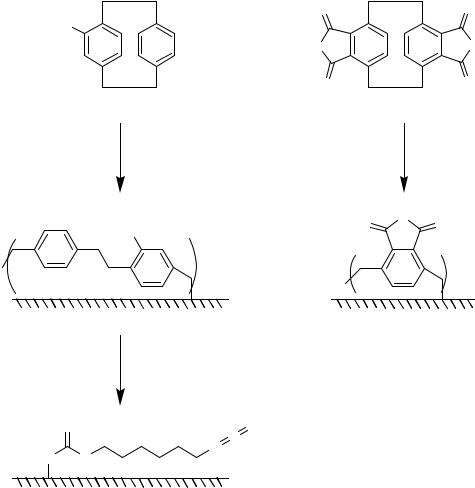
PEPTIDE ARRAYS IN PROTEOMICS AND DRUG DISCOVERY |
171 |
of oxidized silicon wavers using (3-mercaptopropyl)trimethoxysilane in aqueous propanol [308] or in a vacuum chamber [333], yielded mercapto functions suitable for the covalent attachment of cysteinyl peptides via S-S-bond formation (Figure 7.3; [308]).
Functionalization of stainless steel foils or nickel/titanium alloys by chemical vapour deposition polymerization of substituted [2.2]paracyclophanes resulting in poly(amino-p- xylylene-co-p-xylylene) or poly(hydroxy methyl-p-xylylene-co-p-xylylene) coatings (Figure 7.9) was demonstrated by Lahann et al. [283, 284].
O |
O |
HX |
|
O |
O |
O |
O |
i |
|
|
ii |
HX |
O |
O |
O |
|
|||
|
|
|
|
|
n |
|
n |
iii
O O
C
N
XN H
FIGURE 7.9. Surface coatings on stainless steel foils or nickel/titanium alloy [283, 284]. i = chemical vapor deposition polymerization of 4-amino[2.2]paracyclophane (X = NH) or 4-hydroxymethyl[2.2]paracyclophane (X = CH2-O) yielding poly(amino-p-xylylene-co-p-xylylene) or poly(hydroxy methyl-p-xylylene-co-p-xylylene) coatings, respectively; ii = chemical vapor deposition polymerization of [2.2]paracyclophane 4,5,12,13-tetracarboxylic acid anhydride yielding poly(p-xylylene-2,3-dicarboxylic acid anhydride coatings; iii = hexamethylene diisocyanate, diethylether.
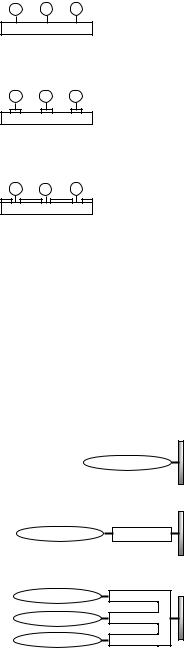
172 |
ULRICH REINEKE, JENS SCHNEIDER-MERGENER AND MIKE SCHUTKOWSKI |
A B C
coherent chip
A B C
feature top
A B C
feature down
FIGURE 7.10. General principles of array architecture. The coherent chips represent the “standard” peptide array. Micropattered arrays can be separated depending on the position of the immobilized peptides (A, B and C) into feature top or down arrays.
In principle, peptide arrays show different architectures: besides the classical coherent peptide array, structured arrays are also used (Figure 7.10). Two different types of structured arrays can be distinguished depending on the position of the features: feature top exemplified in gel pad arrays [136, 190, 367, 430, 565] or arrays of gold electrodes [345] and feature down [88, 225, 230, 392, 502, 604]. The direct attachment of peptides to the surface (Figure 7.11A) is only possible if the planar surface used is equipped with functional groups per se, such as hydroxyl groups of natural polymer cellulose, allowing
A |
|
peptide |
B |
peptide |
spacer |
|
peptide |
|
C |
peptide |
dendrimer |
|
peptide |
|
FIGURE 7.11. Different possible architectures of immobilized peptides. A = peptides directly attached to the surface, B = immobilized peptide is anchored apart from the surface by a chemical moiety introduced as spacer molecule, C = dendrimeric molecules inserted between the peptide and the surface enabling both appropriate spacing and increased density of immobilized peptide: combinations of C and B are used.