
книги студ / Color Atlas of Pathophysiology (S Silbernagl et al, Thieme 2000)
.pdf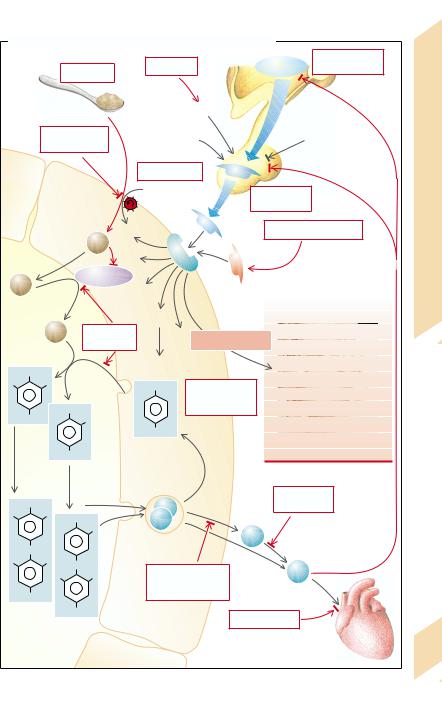
A. Causes of Hypothyroidism, Hyperthyroidism and Goitre
|
1 |
|
Stress |
|
|
Hypothalamic |
|
Iodine |
|
TRH |
lesion |
||
|
|
deficiency |
|
|
|
|
|
|
Food |
Catecholamines |
|
|
|
|
|
|
|
|
||
|
Iodine excess, |
Estrogens |
|
Dopamine |
||
|
|
Somatostatin |
||||
|
rhodanide, etc. |
|
|
|
||
|
|
|
|
Glucocorticoids |
||
|
|
|
|
|
|
|
|
|
2 |
Carrier defect |
TSH |
|
|
|
|
|
Na+ |
|
Pituitary |
|
|
|
|
|
|
|
|
|
|
|
|
|
insufficiency |
|
|
|
|
|
|
Autoimmune disease |
|
|
|
J– |
|
|
TSI |
|
|
|
|
|
|
|
|
J– |
|
Peroxidase |
|
|
|
|
|
3 |
|
|
|
|
|
|
|
|
|
|
|
|
|
J2° |
Peroxidase |
Cell proliferation |
|
||
|
|
deficiency |
|
|
||
OH |
|
|
Thyroglobulin |
|
|
|
|
5 |
4 |
|
|
|
|
J |
J |
OH |
Cell damage |
|
||
|
|
|||||
|
|
|
|
by tumor, |
|
|
|
|
OH |
|
inflammation etc. |
|
|
TG |
|
|
|
|
|
|
|
J |
|
|
|
|
|
~DJT |
|
TG |
|
|
|
|
|
|
TG |
|
|
|
Goitre |
|
|
|
|
|
|
|
Coupling |
~MJT |
Proteolysis |
|
|
||
|
|
|
||||
|
|
|
|
|
||
|
6 |
|
|
|
Defective |
|
OH |
|
|
|
|
||
|
|
|
7 |
conversion |
||
J |
J |
OH |
|
|
|
|
|
|
|
|
|
|
|
|
|
J |
|
|
T4 |
|
|
|
|
|
|
|
|
O |
|
|
|
|
8 |
|
J |
J |
O |
|
|
T3 |
|
|
|
Genetic defect, |
|
|||
|
J |
J |
|
|||
|
iodine excess, |
|
|
|||
|
|
|
|
|
||
TG |
|
|
lithium |
|
|
|
~T4 |
|
|
|
|
|
|
|
TG |
Follicle epithelial cell |
|
|
||
|
|
Receptor defect |
|
|||
|
|
~T3 |
|
|||
Storage |
of the thyroid |
|
|
|||
|
|
|
|
|||
|
Colloid |
|
|
Target organs, |
||
|
|
|
|
e. g. heart |
Plate 9.13 Hypo-/Hyperthyroidism, Goitre: Causes
281
Silbernagl/Lang, Color Atlas of Pathophysiology © 2000 Thieme
All rights reserved. Usage subject to terms and conditions of license.

9 Hormones
282
Effects and Symptoms of Hyperthyroidism
In many tissues the thyroid hormones (T3, T4) increase enzyme synthesis, Na+/K+-ATPase activity and oxygen consumption, leading to an increase in basal metabolism and a rise in body temperature. By stimulating glycogenolysis and gluconeogenesis, the thyroid hormones cause an increase in blood glucose concentration, while on the other hand also increasing glycolysis. They stimulate lipolysis, the breakdown of VLDL and LDL as well the excretion of bile acids in the bile. They stimulate, via increased oxygen consumption, the release of erythropoietin and thus erythropoiesis. The high 2,3-bisphosphoglycerate (DPG) content in newly formed erythrocytes decreases O2 affinity and thus favors the peripheral release of O2. Thyroid hormones sensitize the target organs to catecholamines (especially by an increase in β-receptors) and thus increase, for example, cardiac contractility and heart rate. In addition, they raise intestinal motility and stimulate the transport processes in the gut and kidneys. They promote physical (e.g., longitudinal growth) and mental (especially intellectual) development. T3 and T4 stimulate the restructuring of bone and muscle, the catabolic effect predominating and increase neuromuscular excitability. T3 and T4 act mainly through enhanced gene expression, which takes several days. Beyond this their prolonged action is due to the long half-life in blood (T3 = one day; T4 = seven days). Maternal T3 and T4 are largely inactivated in the placenta and thus have only a slight effect on the fetus.
In hyperthyroidism metabolism and heat production are raised (→ A1). Basal metabolism can nearly double. The affected patients prefer cold ambient temperature; in a hot environment they tend to break into a sweat (heat intolerance). The increased O2 demand requires hyperventilation and stimulates erythropoiesis. The raised lipolysis leads to weight loss, on the one hand, and to hyperlipidacidemia, on the other (→ A1). At the same time, the concentrations of VLDL, LDL, and cholesterol are diminished (→ A2). The effects on carbohydrate metabolism (→ A3) favor the development of (reversible) diabetes mellitus. When glucose is given (glucose tolerance test), plasma glucose concentration rises
more quickly and more markedly than in healthy people; the rise is followed by a rapid fall (abnormal glucose tolerance). Although the thyroid hormones promote protein synthesis, hyperthyroidism increases proteolytic enzymes, and thus causes excess proteolysis with an increase in urea formation and excretion. Muscle mass is reduced (→ A1). Breakdown in bone matrix can lead to osteoporosis, hypercalcemia, and hypercalciuria (→ A4). As a result of the stimulating action on the heart, cardiac output (CO) and systolic blood pressure are raised (→ A5). Atrial fibrillation may occasionally occur. The peripheral vessels are dilated. The glomerular filtration rate (GFR), renal plasma flow (RPF), and tubular transport are increased in the kidneys (→ A6), while in the liver the breakdown of steroid hormones and drugs is accelerated. Stimulation of the intestinal musculature leads to diarrhea; the increase in neuromuscular excitability to hyperreflexia, tremor, muscular weakness, and insomnia (→ A7). In children, accelerated growth may sometimes occur (→ A4). T3 and T4 promote the expression of their receptors and thereby sensitize their target organs to their actions, thus increasing the effects of hyperthyroidism.
In immunogenic hyperthyroidism (Graves’ disease; → p. 280) exophthalmos may be added to the increased effects of thyroid hormones (→ A8); protrusion of the eyes with diplopia, excessive tear flow, and increased photophobia also occur. Its cause lies in an immune reaction against retrobulbar antigens that are apparently similar to the TSH receptors. The result is a retrobulbar inflammation with swelling of the eye muscles, lymphocytic infiltration, accumulation of acid mucopolysaccharides, and an increase in retrobulbar connective tissue. Sometimes similar changes can be found in the pretibial region.
Silbernagl/Lang, Color Atlas of Pathophysiology © 2000 Thieme
All rights reserved. Usage subject to terms and conditions of license.
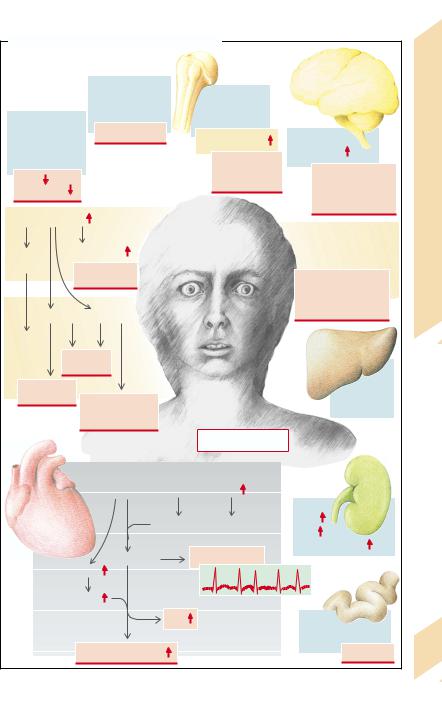
A. Effects and Symptoms of Hyperthyroidism |
|
|
|||||
|
|
|
3 |
|
4 |
|
Symptoms |
|
|
|
|
|
|
||
|
|
|
Stimulation of |
|
Accelerated |
|
|
2 |
|
|
glycogenolysis, |
|
|
||
|
|
gluconeogenesis |
|
growth |
|
||
Accelerated |
|
|
in children |
7 |
and |
||
|
|
|
|||||
breakdown of |
|
Hyperglycemia |
|
|
|
||
VLDL and LDL, |
|
|
|
Bone metabolism |
Neuromuscular |
Effects |
|
stimulation of |
|
|
|
|
excitability |
||
bile secretion |
|
|
|
Osteoporosis |
|
||
|
|
|
|
|
Hypercalcemia |
Hyperreflexia |
|
VLDL |
|
|
|
|
Hyperthyroidism: |
||
|
|
|
|
Hypercalciuria |
Tremor |
||
Cholesterol |
|
|
|
|
Insomnia |
||
|
|
|
|
|
|
||
1Energy metabolism |
|
|
|
Muscle weakness |
|||
|
|
|
8 |
||||
|
|
|
|
|
|
||
O2 con- |
Heat metabolism |
|
|
In Grave’s disease: |
|||
|
|
retrobulbar inflammation |
|||||
|
|
|
|
|
|
||
sumption |
|
Hyperthermia |
|
|
|
9.14 |
|
|
|
|
|
Exophthalmos |
|||
|
|
Sweating |
|
|
|||
|
|
|
|
|
|
Tear flow |
|
|
|
|
|
|
|
Plate |
|
|
|
|
|
|
|
Light sensitivity |
|
|
Lipolysis |
Proteolysis |
|
|
Diplopia |
||
|
|
|
|
|
|||
Hyper- |
|
|
|
|
|
|
|
ventilation |
Weight |
|
|
|
|
||
|
|
|
|
|
|||
|
|
loss |
|
|
|
|
|
Hyperlipid- |
|
|
|
|
Breakdown |
|
|
acidemia |
|
|
|
|
|
||
Decrease in |
|
|
of steroids |
|
|||
|
|
|
|
|
|||
|
|
muscle mass |
|
|
and drugs |
|
|
|
|
Muscle weakness |
|
|
|
|
|
|
|
|
|
|
Hyperthyroidism |
|
|
|
|
5 |
Stimulation |
|
|
|
|
|
|
|
|
|
|
|
|
|
|
|
of the heart |
Oxygen consumption |
|
|
|
|
|
|
Vasodilation |
Erythropoiesis |
GFR |
|
|
|
|
|
RPF Sodium |
|
|||
|
|
|
|
|
|
|
|
|
|
|
Tachycardia |
|
Atrial fibrillation |
reabsorption |
|
|
Cardiac |
|
|
|
|||
|
|
|
|
|
|
||
|
contractility |
|
|
|
|
||
|
Stroke volume |
|
|
|
|
||
|
|
|
|
CO |
|
Stimulation of |
|
|
|
|
|
|
|
|
|
|
|
|
|
|
|
intestinal musculature |
|
|
|
|
Blood pressure |
|
|
Diarrhea |
283 |
|
|
|
amplitude |
|
|
||
|
|
|
|
|
|
|
Silbernagl/Lang, Color Atlas of Pathophysiology © 2000 Thieme
All rights reserved. Usage subject to terms and conditions of license.

9 Hormones
284
Effects and Symptoms of Hypothyroidism
For a description of the functions of the thyroid hormones, see p. 282. Metabolism and heat production are reduced in hypothyroidism. Basal metabolic rate may fall by half (→A1), and the patients easily feel cold (cold intolerance). Oxygen consumption, ventilation, and erythropoiesis are diminished. In addition, the development of anemia is encouraged by the impaired absorption in the gut of iron, folic acid, and vitamin B12. Reduced lipolysis promotes weight increase and hyperlipidemia
(VLDL, LDL), while the reduced breakdown of cholesterol to bile acids quickly leads to hypercholesterolemia, and thus favors the development of atherosclerosis (→A2). Impairment of glycogenolysis and gluconeogenesis can result in hypoglycemia (→A3). Reduced breakdown of glycosaminoglycans (mucopolysaccharides, mucin) causes them to be deposited in various tissues and a dough-like consistency of the skin, which is why the disease has been called myxedema (→A4). In addition, fibronectin, collagen, and plasma albumin are deposited in the skin. Reduced transformation of carotene into vitamin A causes hyperkeratosis. Also, because of reduced sweat and sebaceous secretion, the skin is dry and the reduced heat production makes it feel cold. The patient often has a hoarse voice.
Reduced stimulation of the heart by thyroid hormones decreases contractility, heart rate, stroke volume, cardiac output and occasionally also the systolic blood pressure (→A5). In marked thyroid hormone deficiency heart failure can develop. Pleural and pericardial effusions are common. The rate of breathing is slowed and the ventilatory reaction to hypercapnia and hypoxia is impaired.
The glomeruli and tubules in the kidneys are smaller. Glomerular filtration rate, renal plasma flow, and tubular transport capacity are reduced. Decreased renal elimination leads to water and NaCl retention (→A6). Due to the accumulation of fat, glycosaminoglycans, NaCl, and water, the patient may look somewhat bloated.
In addition, protein synthesis in the liver is impaired and the breakdown of steroid hormones and drugs is delayed.
The reduced stimulation of the intestinal
musculature leads to constipation. Impaired function of the esophageal musculature and of the gastroesophageal sphincter may cause gastric reflux and esophagitis.
The activity and effectiveness of the autonomic nervous system is reduced in hypothyroidism (→A7). Neuromuscular excitability is also reduced, resulting in abnormal sensory functions, hyporeflexia, loss of appetite, loss of memory, depression, and clouding of consciousness progressing even to coma. These defects are reversible in adults. However, a lack of thyroid hormone in fetuses and neonates will produce irreversible brain damage. The thyroid hormones are necessary for the full development of dendrites and axons, the formation of synapses, myelination, and glial formation—all processes that are absolutely essential for brain development in the fetus and up to two years after birth. Intrauterine deficiency of thyroid hormones thus massively impairs this development. If substitution with thyroid hormones after birth is omitted, brain damage occurs that cannot be reversed by later thyroid hormone administration. Affected children are often deaf.
Furthermore, bone growth is delayed in these children (→A8). Retarded growth and impaired mental capacity lead to the typical feature of cretinism.
The functional effects of thyroid hormone deficiency are accentuated by a reduced expression of T3 and T4 receptors.
A T3/T4 deficiency disinhibits the formation of TRH and TSH (→p. 280). TSH not only stimulates the formation of TSH, but also of prolactin, and can thus trigger hyperprolactinemia (→p. 260). TSH also promotes the growth of the thyroid gland, causing goitre (→p. 280). Lastly, abnormal release of gonadotropins can impair fertility.
Silbernagl/Lang, Color Atlas of Pathophysiology © 2000 Thieme
All rights reserved. Usage subject to terms and conditions of license.
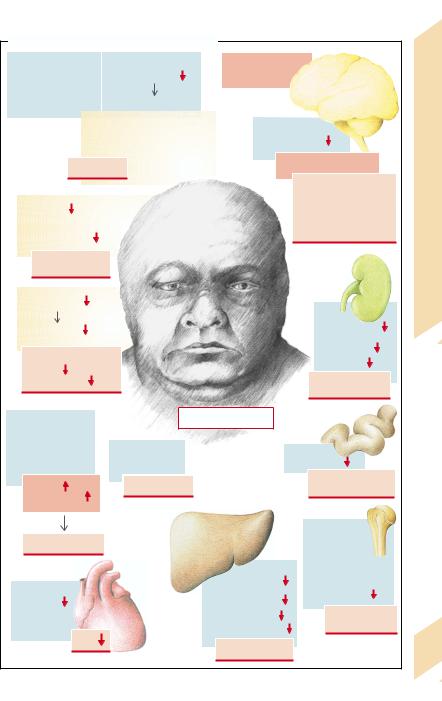
A. Effects and Symptoms of Hypothyroidism |
|
|
|
|||
|
Depostion of |
|
Filtration of albumin |
Impaired cerebral |
andSymptoms |
|
|
|
Na+ retention |
development |
|
||
|
glycosamineglycans |
|
|
|
|
|
|
(mucin) |
|
Edema |
|
|
|
|
|
|
7 |
|
||
|
|
|
|
|
||
|
|
|
Effusion, |
Neuromuscular |
||
|
4 |
|
hoarse voice, |
excitability |
Effects |
|
|
|
puffed-up face |
|
|
||
|
|
|
|
|
||
|
Myxedema |
|
Sensory disturbances |
|||
|
|
|
|
|
Hyporeflexia |
Hypothyroidism: |
|
Vitamin A |
|
|
|
Lack of drive |
|
|
|
|
|
Depression |
||
|
Sebaceous |
|
|
|
||
|
|
|
|
Clouded consciousness |
||
|
and sweat |
|
|
|
||
|
|
|
|
|
||
|
gland activity |
|
|
|
|
|
|
|
|
|
|
|
|
|
Dry, scaly skin |
|
|
|
|
9.15 |
|
|
|
|
|
|
|
1 |
Energy |
|
|
|
|
Plate |
|
metabolism |
|
|
|
|
|
|
|
|
|
|
Renal |
|
|
|
|
|
|
|
|
|
Heat |
|
|
|
blood flow |
|
|
metabolism |
|
|
|
Glomerular |
|
|
Sensitivity to cold |
|
|
|
filtration |
|
|
|
|
|
Transport |
|
|
|
Appetite |
|
|
|
|
|
|
|
|
|
|
|
|
|
Erythropoiesis |
|
|
|
Salt and |
|
|
|
|
|
|
water retention |
|
|
|
|
Hypothyroidism |
6 |
|
|
|
Delayed breakdown |
|
|
|
||
|
|
|
|
|
|
|
|
of VLDL |
|
|
|
|
|
|
Reduced |
2 |
Reduced lipolysis |
|
Intestinal |
|
|
bile secretion |
|
motility |
|
||
|
VLDL |
|
Overweight |
|
Obstipation |
|
|
|
|
Reflux esophagitis |
|
||
|
Cholesterol |
|
|
|
|
|
|
|
|
|
|
8 |
|
|
|
|
|
|
In children: |
|
|
Atherosclerosis |
|
|
|
retarded |
|
|
|
|
|
|
longitudinal growth |
|
|
5 |
|
|
|
and delayed |
|
|
|
|
Protein synthesis |
epiphyseal fusion |
|
|
|
|
|
|
somatotropin |
|
|
|
Cardiac |
|
|
Elimination of |
|
|
|
|
3 |
release |
|
||
|
contractility |
|
drugs |
|
||
|
|
|
|
|||
|
Bradycardia |
|
|
Glycogenolysis |
Small stature |
|
|
|
|
|
Gluconeogenesis |
Cretinism |
|
|
CO |
|
|
|
|
|
|
|
|
Hypoglycemia |
|
|
|
|
|
|
|
|
285 |
Silbernagl/Lang, Color Atlas of Pathophysiology © 2000 Thieme
All rights reserved. Usage subject to terms and conditions of license.

9 Hormones
286
Causes of Diabetes Mellitus
Diabetes mellitus is caused by an absolute or relative lack of insulin that, among other consequences, leads to an increase in plasma glucose concentration (see p. 288 for the way in which insulin acts). The disease was given its name because of the glucose excretion in the urine. The disease can be classified into several types, depending on its cause and course. This classification is useful, even though it is greatly simplified.
In type I (insulin-dependent diabetes mellitus [IDDM], previously called juvenile diabetes; →A) there is an absolute lack of insulin, so that the patient needs an external supply of insulin. The condition is caused by a lesion in the beta cells of the pancreas, as a rule produced by an autoimmune mechanism that may, in certain circumstances, have been triggered by a viral infection. The pancreatic islets are infiltrated by T lymphocytes and autoantibodies against islet tissue (islet cell antibodies [ICA]) and insulin (insulin autoantibodies [IAA]) can be detected. ICA may in some cases be detected years before the onset of the disease. After the death of the beta cells, the ICA again disappear. 80% of patients form antibodies against glutamatedecarboxylase expressed in the beta cells. Type I diabetes mellitus occurs more frequently in the carriers of certain HLA antigens (HLA-DR3 and HLADR4), i.e., there is a genetic disposition.
Type II (non-insulin-dependent diabetes mellitus [NIDDM], formerly called maturityonset diabetes; →B) is by far the most common form of diabetes. Here, too, genetic disposition is important. However, there is a relative insulin deficiency: the patients are not necessarily dependent on an exogenous supply of insulin. Insulin release can be normal or even increased, but the target organs have a diminished sensitivity to insulin.
Most of the patients with type II diabetes are overweight. The obesity is the result of a genetic disposition, too large an intake of food, and too little physical activity. The imbalance between energy supply and expenditure increases the concentration of fatty acids in the blood. This in turn reduces glucose utilization in muscle and fatty tissues. The result is a resistance to insulin, forcing an increase of in-
sulin release. The resulting down-regulation of the receptors further raises insulin resistance. Obesity is an important trigger, but not the sole cause of type II diabetes. More important is the already existing genetic disposition to reduced insulin sensitivity. Frequently, insulin release has always been abnormal. Several genes have already been defined that promote the development to obesity and type II diabetes. Among other factors, the genetic defect of a mitochondrial decoupling protein limits substrate consumption. If there is a strong genetic disposition, type II diabetes can already occur at a young age (maturity-onset diabetes of the young [MODY]).
Reduced insulin sensitivity predominantly affects the insulin effect on glucose metabolism, while the effects on fat and protein metabolism are still well maintained. Thus, type II diabetics tend especially toward massive hyperglycemia without corresponding impairment of fat metabolism (ketoacidosis, → p. 288).
Relative insulin deficiency can also be caused by autoantibodies against receptors or insulin as well as by very rare defects in the biosynthesis of insulin, of insulin receptors, or of intracellular transmission (→C).
Even without any genetic disposition, diabetes can occur in the course of other diseases, such as pancreatitis, with destruction of the beta cells (pancreas-deprived diabetes; →C), or by toxic damage to these cells. The development of diabetes mellitus is promoted by an increased release of antagonistic hormones. Among these are somatotropin (in acromegaly), glucocorticoids (in Cushing’s disease or stress [so-called steroid diabetes]), epinephrine (in stress), progestogens and choriomammotropin (in pregnancy), ACTH, thyroid hormone, and glucagon. Severe infections increase the release of several of the above hormones and thus the manifestation of diabetes mellitus (→C). A somatostatinoma can cause diabetes because the stomatostatin secreted by it inhibits the release of insulin.
Silbernagl/Lang, Color Atlas of Pathophysiology © 2000 Thieme
All rights reserved. Usage subject to terms and conditions of license.
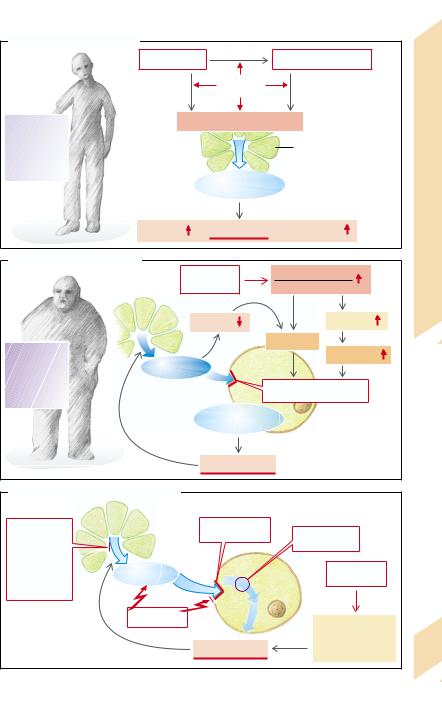
A. Diabetes Mellitus: Type I |
|
|
|
|
|
Viral infection |
? |
Autoimmune disease |
|
||
|
|
|
|
||
|
|
Genetic |
|
|
|
|
? |
disposition |
? |
|
|
|
|
? |
|
|
|
TYPE l |
Destruction of beta cells |
|
|||
|
|
|
Causes |
||
10 % |
|
|
Beta cells |
||
of all |
|
|
|||
diabetics |
|
|
(pancreas) |
||
|
|
|
|||
|
|
|
|
||
|
|
Absolute |
|
Mellitus: |
|
|
insulin deficiency |
||||
|
|
|
|
||
Lipolysis |
Hyperglycemia |
Protein |
Diabetes |
||
breakdown |
|||||
|
|
|
|||
B. Diabetes Mellitus: Type II |
|
|
|
||
|
|
|
|
||
|
Familial |
|
Substrate uptake |
9.16 |
|
|
disposition |
Substrate consumption |
|||
|
|
|
|
Plate |
Lipolysis |
Fatty acids |
|
Obesity |
TYPE lI |
Ketone bodies |
90 % |
Insulin |
Target cell |
|
|
of all |
|
|
|
|
diabetics |
|
|
Insulin insensitivity |
|
|
|
|
|
|
|
|
Relative |
|
|
|
|
insulin deficiency |
|
|
|
|
Hyperglycemia |
|
|
C. Other Causes of Diabetes Mellitus |
|
|
|
|
Abnormal |
|
Unresponsive |
Postreceptor |
|
|
receptor |
|
||
insulin release |
|
|
defect |
|
Removal of |
|
|
|
|
pancreas |
|
|
Severe |
|
Pancreatic |
Insulin |
|
|
|
|
infections |
|
||
disease |
|
Target cell |
|
|
|
Antibodies |
|
|
|
|
|
|
Antagonistic |
|
|
|
|
hormones |
|
|
|
Hyperglycemia |
(e. g. in pregnancy) |
287 |
|
|
|
Silbernagl/Lang, Color Atlas of Pathophysiology © 2000 Thieme
All rights reserved. Usage subject to terms and conditions of license.

9 Hormones
288
Acute Effects of Insulin Deficiency (Diabetes Mellitus)
Insulin acts to create energy reserves. It promotes the uptake of amino acids and glucose, especially in the muscle and fat cells. In hepatic, muscle, and fat cells (among others) insulin stimulates protein synthesis and inhibitis protein breakdown; in the liver and muscles it promotes glycogen synthesis, inhibits its breakdown, stimulates glycolysis, and inhibits gluconeogenesis from amino acids. Also in the liver, insulin promotes the formation of triglycerides and lipoproteins as well as the hepatic release of VLDL. At the same time it stimulates lipoprotein lipase and thus accelerates the splitting of triglycerides into lipoproteins in blood (especially chylomicrons). The free fatty acids and glycerol are then taken up by the fat cells and stored again as triglycerides. Insulin stimulates lipogenesis and inhibits lipolysis in the fat cells. Lastly, it promotes cell growth, increases renal tubular absorption of Na+, and cardiac contractility. Part of insulin action is mediated by cell swelling (especially antiproteolysis) and intracellular alkalosis (stimulation of glycolysis, increased cardiac contractility). Insulin achieves this effect by activating the Na+/H+ exchanger (cell swelling and alkalinization), the Na+-K+-2 Cl– cotransporter (cell swelling), and Na+-K+-ATPase. This results in K+ uptake by the cell and hypokalemia. As glucose is coupled to phosphate in the cell, insulin also reduces plasma phosphate concentration. It further stimulates the cellular uptake of Mg2+. Insulin also paracrinally inhibits the release of glucagon and thus diminishes its stimulating action on glycogenolysis, gluconeogenesis, lipolysis, and ketogenesis.
In acute insulin deficiency the absence of its effect on glucose metabolism results in hyperglycemia (→ A1). The extracellular accumulation of glucose leads to hyperosmolarity. The transport maximum of glucose is exceeded in the kidney so that glucose is excreted in the urine (→ A2). This results in an osmotic diuresis with renal loss of water (polyuria), Na+, and K+, dehydration, and thirst. Despite the renal loss of K+, there is no hypokalemia because the cells give up K+ as a result of reduced activity of Na+-K+-2 Cl– cotransport and of Na+-K+- ATPase. The extracellular K+ concentration, which is therefore more likely to be high, dis-
guises the negative K+ balance. Administration of insulin then causes a life-threatening hypokalemia (→ p.124). Dehydration leads to hypovolemia with corresponding impairment of the circulation. The resulting release of aldosterone increases the K+ deficiency, while the release of epinephrine and glucocorticoids exacerbates the catabolism. The reduced renal blood flow diminishes the renal excretion of glucose and thus encourages the hyperglycemia.
The cells further lose phosphate (Pi) and magnesium that are also excreted by the kidney. If there is an insulin deficiency, proteins are broken down to amino acids in muscles and other tissues. This breakdown of muscles will, together with electrolyte abnormalities, lead to muscular weakness. Prevailing lipolysis leads to release of fatty acids into blood (hyperlipidacidemia). The liver produces acetoacetic acid and β-hydroxybutyric acid from the fatty acids. Accumulation of these acids leads to acidosis, which forces the patient to breathe deeply (Kussmaul breathing; → A3). Some of the acids are broken down to acetone (ketone bodies). In addition, triglycerides are formed in the liver from fatty acids and incorporated into VLDL. As the insulin deficiency delays the breakdown of lipoproteins, the hyperlipidemia is further aggravated. Some of the triglycerides remain in the liver and a fatty liver will develop.
The breakdown of proteins and fat as well as polyuria will result in weight loss. The abnormal metabolism, electrolyte disorders and the changes in cell volume brought about by changed osmolarities can impair neuronal function and cause hyperosmolar or ketoacidotic coma.
The main effects of relative insulin deficiency are hyperglycemia and hyperosmolarity, while in absolute insulin deficiency the consequences of increased proteolysis and lipolysis (ketoacidosis) are added to these effects.
Silbernagl/Lang, Color Atlas of Pathophysiology © 2000 Thieme
All rights reserved. Usage subject to terms and conditions of license.

A. Acute Effects of Diabetes Mellitus |
|
|
|
|
||
|
Acute insulin deficiency |
|
|
|
||
Proteolysis |
|
|
Lipolysis |
|
|
|
|
|
|
Weight |
|
|
Effects |
Muscle weakness |
|
loss |
|
|
||
|
|
|
|
|||
|
|
|
|
|
|
|
|
|
Fatty liver |
Fatty acids in blood |
Acute |
||
|
|
|
|
|||
|
|
|
|
|
|
|
Amino acids |
|
|
|
|
|
Mellitus: |
|
|
Glycogen |
VLDL |
|
||
|
Gluco- |
breakdown |
|
|
||
|
|
|
|
|
||
|
|
|
|
|
Diabetes |
|
|
neogenesis |
|
LDL |
|
||
|
|
|
|
|
||
|
|
|
1 |
|
|
|
|
Glucose excess |
|
|
|
9.17 |
|
|
Mg2+ |
|
|
Osmolarity |
|
Plate |
|
Pi |
|
|
Ketone bodies |
|
|
|
H2O |
H |
+ |
Reduced |
|
|
H+ |
glycolysis |
|
|
|||
|
|
|
||||
|
|
|
|
|||
|
Na+ |
Na+ |
H+ |
|
|
|
|
|
|
|
|
|
|
|
|
K+ |
|
|
|
|
|
K+ |
Na+ |
Acidosis |
|
||
|
|
|
|
|||
|
|
2Cl– |
|
|
|
|
Kidney |
|
|
Coma |
3 |
|
|
|
Aldosterone |
|
|
|
|
|
|
Dehydration |
|
Fruit-lozenge |
|
|
|
|
|
breath |
|
|
||
2 |
|
|
|
|
|
|
|
|
|
|
|
|
|
Glucosuria |
|
Thirst |
|
Kussmaul breathing |
|
|
|
|
|
|
|
||
Aminoaciduria |
|
|
|
|
|
|
Polyuria |
|
|
|
|
|
|
Electrolyte loss |
|
|
|
|
|
|
|
|
|
|
|
|
289 |
Silbernagl/Lang, Color Atlas of Pathophysiology © 2000 Thieme
All rights reserved. Usage subject to terms and conditions of license.

9 Hormones
290
Late Complications of Prolonged Hyperglycemia (Diabetes Mellitus)
The metabolic abnormalities of inadequately treated relative or absolute insulin deficiency (→ p. 286 – 289) will in the course of years or decades lead to extensive irreversible changes in the organism. Hyperglycemia plays a central role in this.
Glucose is reduced to sorbitol in cells that contain the enzyme aldosereductase. This hexahydric alcohol cannot pass across the cell membrane, as a result of which its cellular concentration increases and the cell swells (→ A1). Due to an accumulation of sorbitol in the lens of the eye, water is incorporated, impairing lenticular transparency (clouding of the lens [cataract]; → A2). Accumulation of sorbitol in the Schwann cells and neurons reduces nerve conduction (polyneuropathy), affecting mainly the autonomic nervous system, reflexes, and sensory functions (→ A3). To avoid swelling, the cells compensate by giving off myoinositol which then, however, will not be available for other functions.
Cells that do not take up glucose in sufficient amounts will shrink as a result of extracellular hyperosmolarity (→ A4). The functions of lymphocytes that have shrunk are impaired (e.g., the formation of superoxides, which are important for immune defense). Diabetics are thus more prone to infection (→ A5), for example, of the skin (boils) or kidney (pyelonephritis). These infections, in turn, increase the demand for insulin, because they lead to an increased release of insulin-antago- nistic hormones (→ p. 286).
Hyperglycemia promotes the formation of sugar-containing plasma proteins such as fibrinogen, haptoglobin, α2-macroglobulin as well as clotting factors V– VIII (→ A6). In this way clotting tendency and blood viscosity may be increased and thus the risk of thrombosis raised.
By binding of glucose to free amino-groups of proteins and a subsequent, not fully understood, irreversible Amadori reaction, advanced glycation end products (AGEs) are formed. They also occur in increasing amounts in the elderly. A protein network can be formed through the formation of pentosin. AGEs bind to respective receptors of the cell membrane and can thus promote the deposition of col-
lagen in the basement membranes of the blood vessels. The formation of connective tissue is in part stimulated via transforming growth factor β (TGF-β). Additionally, however, the collagen fibers can be changed by glycosylation. Both changes produce thickening of the basement membranes with reduced permeability and luminal narrowing (microangiopathy; → A7). Changes occur in the retina, also as a result of microangiopathies, that ultimately may lead to blindness (retinopathy; → A8). In the kidney glomerulosclerosis (Kimmelstiel– Wilson) develops, which can result in proteinuria, reduced glomerular filtration rate due to a loss of glomeruli, hypertension, and renal failure (→ A9). Because of the high amino acid concentration in plasma, hyperfiltration takes place in the remaining intact glomeruli, which as a result are also damaged.
Together with a rise of VLDL in blood (→ p. 288) and the raised clotting tendency of the blood (see above), hypertension promotes the development of a macroangiopathy (→ A10) that can further damage the kidneys and cause myocardial infarction, cerebral infarction, and peripheral vascular disease.
Lastly, glucose can react with hemoglobin (HbA) to form HbA1c, whose increased concentration in blood points to a hyperglycemia that has been present for some time. HbA1 c has a higher oxygen affinity than HbA and thus releases oxygen in the periphery less readily (→ A11). The persisting insulin deficiency further leads to a reduction in the erythrocytic concentration of 2,3-bisphosphoglycerate (BPG), which, as allosteric regulator of hemoglobin, reduces its oxygen affinity. The BPG deficiency also results in an increased oxygen affinity of HbA.
Diabetic mothers have a statistically higher chance of giving birth to a heavier than normal baby (→ A12). This may be the result of an increased concentration of amino acids in blood, producing an increased release of somatotropin.
Silbernagl/Lang, Color Atlas of Pathophysiology © 2000 Thieme
All rights reserved. Usage subject to terms and conditions of license.