
Understanding the Human Machine - A Primer for Bioengineering - Max E. Valentinuzzi
.pdf98 |
Understanding the Human Machine |
vector and the heart vector. The final subject refers to the derangements of the cardiac signal. The biomedical engineers, especially those headed to the medical environment or to cardiologic research, should get a good grasp of these concepts. We gave here a bird’s eye view, pointing mostly to the big print. Arrhythmias were tentatively and grossly divided in rhythmic and arrhythmic, that is, originated in more or less regular blocks and those related to instabilities.
2.3. Respiratory System
Is Earth so unique as to be alone in the Universe, coated with its feeble oxygen film? Can you imagine the challenge for a human being to survive, say, in Mars? And man is getting closer and closer to it…
2.3.1. Introduction
The respiratory system is physically related to the cardiovascular system (Figure 2.1) through the exchanger E2, where the pulmonary capillaries are located. On one side, the airways via the mouth and nose connect the organism to the external atmosphere. On the inner side, there is the pulmonary circulation and its blood stream. In simple words, respiration can be described as the optimal adjustment of the depth and rate of breathing to meet the metabolic demands of the body (input of O2, output of CO2) with minimal work of the respiratory muscles. This is valid for any mammal, including the human being.
However, the respiratory system takes care of other secondary functions: it cooperates in the maintenance of the acid–base equilibrium, it helps in the regulation of water loss (in some species, as the dog, this function is extremely important because this animal does not have sweating glands), it helps in the heat loss process (especially when the environmental temperature goes up), it collaborates in the circulation of blood through the pulmonary vessels with its mechanical pumping action, it plays a role in emotional manifestations such as crying, sobbing and laughing, it is essential in protecting reflexes (coughing, sneezing, vomiting), and it is central to other reflexes of imprecise and yet not well understood nature (yawning and hiccupping).
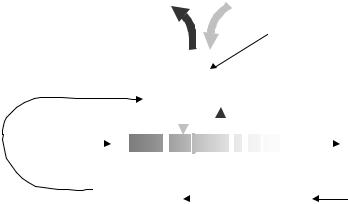
Chapter 2. Source: Physiological Systems and Levels |
99 |
|||||||||||||||||||||
|
|
|
|
|
|
Expired Air |
|
Inspired Air |
|
|||||||||||||
|
|
|
|
|
|
|
|
|
|
|
|
|
|
|
|
Airways |
|
|||||
|
|
|
|
|
|
|
|
|
|
|
|
|
|
|
|
|||||||
|
|
|
|
|
|
|
|
|
|
|
|
|
|
|
|
|
Alveolar wall |
|||||
|
|
|
|
|
|
|
|
|
|
|
Lungs |
|
|
|
|
Interstitial space |
||||||
|
|
|
|
|
|
|
|
|
|
|
|
|
|
|
|
|
Capillary wall |
|||||
|
|
|
|
|
|
|
|
|
|
|
|
|
|
|
|
|
|
|||||
From |
|
|
|
|
|
|
|
|
|
|
|
|
|
|
|
|
|
|
|
|
|
To Systemic |
|
|
|
|
|
|
|
|
|
|
|
|
|
|
|
|
|
|
|
|
|
||
|
|
|
|
|
|
|
|
|
|
|
|
|
|
|
|
|
|
|
|
|
||
|
|
|
|
|
|
|
|
|
|
|
|
|
|
|
|
|
|
|
|
|
||
Right |
|
|
|
|
|
|
|
|
|
|
|
|
|
|
|
|
|
|
|
|
|
|
|
|
|
|
|
|
|
BLOOD |
|
|
|
|
|
|
|
|
|
|
|||||
Heart |
|
|
|
|
|
|
|
|
|
|
|
|
|
|
|
|
|
Circulation |
||||
|
|
|
|
|
|
|
|
|
|
|
|
|
|
|
|
|
|
|
|
|
||
|
|
|
|
|
|
|
|
|
|
|
|
|
|
|||||||||
|
|
|
|
|
|
Motor |
|
|
|
|
|
Neural |
|
From |
||||||||
|
|
|
|
|
System |
|
|
|
|
|
Control |
|
Sensors |
|||||||||
|
|
|
|
|
|
|
|
|
|
|
|
|
|
|
|
|
|
|
|
|
|
|
Figure 2.40. RESPIRATORY SYSTEM. The lungs, mechanically operated by the motor system (respiratory muscles), in turn driven by a nervous control system which also receives signals from specific sensors, on its external side moves air in and out, and on its internal interface exchanges oxygen and carbon dioxide with the pulmonary circulation through and exchanger formed by the alveolar membrane, the interstitial space and the capillary wall. Blood low in oxygen and high in carbon dioxide enters the pulmonary circulation (left side of figure, shaded area) and leaves it with these concentrations reversed (right side of figure, white area). See text for details.
The inspired air contains O2 at 158 mmHg and CO2 at 0.3 mmHg while partial pressures in the expired mixture are, respectively, 116 and 32 mmHg (Figure 2.40). The normal concentration of these two gases in alveolar air is 100 and 40, respectively, always expressed in mmHg, clearly marking an inward gradient of O2 and an outward one for CO2 Blood from the right heart carries oxygen at 40 mmHg and carbon dioxide at 46 mmHg while, after equilibration at the outflow heading to the left heart, the respective levels are 100 and 40 mmHg.
Problem: Based on a sea level atmospheric pressure, calculate the values given above for inspired air. What physics law are you applying?
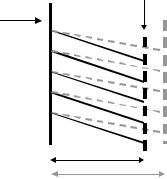
100 |
Understanding the Human Machine |
2.3.2. Mechanics of Respiration
2.3.2.1. Basic mechanisms and principal muscles
Air inflow to the pulmonary chambers requires their expansion and return to the original volume means outflow of air. Two basic mechanisms are responsible for such events:
1.A downward movement of the diaphragm (during inspiration) and an upward movement of the same muscle (during expiration). Thus, this upwardly convex or domed muscle behaves as some kind of piston increasing and decreasing the thoracic cage volume. Approximately 70– 75% of the normal respiration takes place by this mechanism. The phrenic nerves (one on each side) command, respectively, the two halves of the diaphragm (which is a skeletal muscle).
2.Rising and lowering of the frontal face of the ribs, which pivot on the vertebral column. Hence, the net effect is an increase and decrease of the anteroposterior diameter of the thorax and almost no change on the lateral diameter (Figure 2.41). The external intercostal muscles act as reins or straps to lift up the ribs. This mechanism accounts for the other 25–30% of the respiratory act.
Study subject: What other muscles act during forced respiration? Think of these muscles in paraplegic patients with spinal lesions. If the lesion affects the respiratory center, what muscle would you stimulate to obtain a relatively normal respiratory action?
Figure 2.42 illustrates the action of the intercostal muscles after section-
Vertebral
Column
Sternum
D1
D2
Figure 2.41. ANTEROPOSTERIOR DIAMETER CHANGE. Lateral view. The arrow indicates pulling up of the external intercostal muscles. As the ribs rotate, the diameter increases from D1 to D2. No change occurs in the perpendicular direction (lateral). Only during forced expiration the internal intercostal muscles pull down the ribs to decrease the diameter, otherwise this occurs passively.
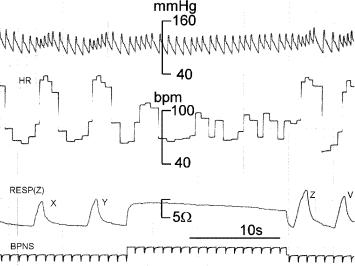
Chapter 2. Source: Physiological Systems and Levels |
101 |
BP
Figure 2.42. INTERCOSTAL AND DIAPHRAGMATIC COMPONENTS OF THE RESPIRATORY MOVEMENTS. Records obtained from an experimental dog whose two phrenic nerves had been sectioned. Thus, the animal was breathing only with its intercostal muscles. First channel: Carotid artery blood pressure. Second channel: Heart rate in beats/min. Third channel: Respiration detected with two transthoracic lateral electrodes connected to an impedance meter. Inspiration is shown by an increase in impedance and expiration by a decrease. Calibration of the third channel was 22.5 ohms per 500 ml of respired air. Records obtained by the author at the Department of Physiology, Baylor College of Medicine, Houston, TX, 1970.
ing of the two phrenic nerves in an experimental animal. Respiration was detected with two electrodes connected to an impedance meter. During inspiration there was an increase in impedance and expiration corresponds to a decrease (fourth channel). Breaths X and Y were produced only by the action of the intercostal muscles. Electrical stimulation of the two phrenic nerves at BPNS (during approximately 21 s) produced a good diaphragmatic contraction and consequent long inspiratory phase, as manifested by the plateau. Hence, even though the nerves had been cut, they were still responsive. Breaths Z and W were also due to the intercostal muscle contractions.
Problem: Using the calibration given in Figure 2.42 for the respiratory channel, calculate the amount of air moved by the intercostal and the diaphragmatic contractions, respectively.
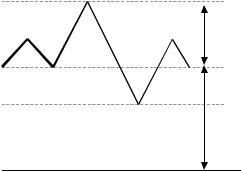
102 |
Understanding the Human Machine |
2.3.2.2. Pulmonary capacities and volumes
A physiological air volume detecting apparatus (as for example, a spirometer) may show changes with time that shift from one level to another, either upward or downward, as the subject breathes following instructions. As illustrated in Figure 2.43, there are four important levels to define: Maximal Inspiratory Level (MIL), reached when the subject is asked to take air in all the way up; Normal Inspiratory Level (NIL), which is a maximum value during normal respiration and, thus, it may be either higher or lower; the resting Normal Expiratory Level (NEL), a minimum obtained also during a normal respiratory act; and the Maximum Expiratory Level (MEL), only reached when the subject voluntarily expels all the air he/she is able to.
The difference between NIL and NEL is called tidal volume TV (about 400 to 500 mL of air/breath in the normal adult). The gas remaining in the lung at the end tidal position NEL is called Functional Reserve Capacity (FRC). If one keeps in mind that “capacities” are comprised of one or more “volumes”, the lung volume subdivisions are easy to deduce. Say, a maximal expiration measured from NEL is called Expiratory Reserve Volume (or NEL–MEL). The air remaining in the lungs at the end of this maneuver is termed Residual Volume (RV, about 1.2 liters). Therefore, the sum of the two volumes, RV and ERV, results in FRC (about 2.2 liters). Similarly, the sum of tidal volume (TV) and Inspiratory Reserve Volume (IRV) equals Inspiratory Capacity (IC, in the order of
MIL mL
Inspiratory Capacity
NIL
NEL
MEL
Functional Reserve Capacity
0
Time
Figure 2.43. PULMONARY CAPACITIES AND VOLUMES. A physiological air volume detecting apparatus (as for example a spirometer) may show changes with time that shift from one level to another as the subject breathes following instructions.
Chapter 2. Source: Physiological Systems and Levels |
103 |
3.8 liters), remembering that IRV (about 3.3 liters) is obtained after (MIL–NIL). In turn, the sum of IC and FRC equals Total Lung Capacity (TLC, 5 to 6 liters), i.e., from zero level up to MIL. The subject can never expel the RV, even after full expiratory exertion
Study subject: If a newborn mammal is found dead, how can it be determined whether it died before or after birth?
Exercise: Write down, as a summary, the simple equations that describe ERV, FRC, IC, IRV and TLC. They are very handy in respiratory volumetry. Find out what a spirometer is. Subject to be refreshed: Review the laws of gases, especially the general law expressed as PV=nRT, where P and V stand, respectively, for pressure and volume, and n represents the number of moles. What is R, what is T? These concepts are extremely important when the numerical experimental values need to be standardized. Hint: R is a universal constant and T is expressed in a unit honoring a famous physicist. Search in INTERNET entering with words like “gases and respiratory volumes”. You will find in the WEB a concise hand out by Dr. Robert A. Furrilla (rfurilla@prtc.net), currently with the University of Puerto Rico.
2.3.2.3. Respiratory variables
− Rate and ventilation
Identification and clear definition of the variables is a basic requirement for any measurement. We have referred to this concept when discussing the cardiovascular system in Section 2. Respiratory rate RR or respiratory frequency fr is perhaps the easiest and most salient. In any emergency situation, it should be checked right away by the attending person (physician, nurse or even a responsible passerby). Typical values in a normal adult range between 12 and 16 breaths/min, however, such rate is not constant for it shows variations. When tidal volume is multiplied by rate, a new variable is defined, ventilation, or
Q ' = RR × TV |
(breaths/min)x(mL/breath = mL/min) |
(2.76) |
In other words, it is flow of air, conceptually similar to flow of blood or of any fluid, either gas or liquid. Accepting 15 breaths/min and 500 mL of air/breath as average normal resting values, Q’ turns out to be 7.5 liters of air/min. During exercise, these values change considerably depending on the physical fitness of the individual (McArdle, Katch & Katch, 1991)
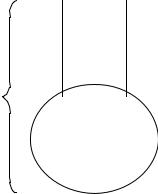
104
VD
VE
VA
Understanding the Human Machine
Figure 2.44. BASES FOR THE DETERMINATION OF THE DEAD SPACE. The lower shaded area represents the alveolar useful space and the vertical tubing stands for the dead volume (not participating in the exchange process). VE is just the sum of the two former.
− Dead space
However, an apparent normal ventilation value does not necessarily mean that the subject is well ventilated. It is required that air reaches the alveolar space in order to actually accomplish the gaseous exchange. A fraction of the air in the respiratory compartments (airways like mouth, nose, throat, trachea, bronchi, bronchioles) doest not get in contact with the diffusion alveolar surface and, as a consequence, does not participate in the respiratory act. All this non-operative volume is called the dead space, VD. As rule of thumb and for a normal adult, either the body weight directly in pounds, or the same body weight in kilograms multiplied by two, both yield a number equal to the dead space in mL. As an example, a person weighing 83 kgs (≈166 lbs) would have a VD = 166 mL.
Thus, assuming a normal tidal volume of 500 ml, about 30% of this air is useless in the sense that it does not participate in gas exchange. Physiologic dead space includes all the previously mentioned non-respiratory parts of the anatomic dead space plus alveoli which are well-ventilated but poorly perfused (or viceversa) and are, therefore, less efficient in the gas exchange process.
Let us work with a simple model (Figure 2.44) to obtain a method of measurement of the dead space. In it, the total volume VE is the sum of the other two volumes VD (dead volume) and VA (alveolar volume). Besides, we recall that the concentration of a gas in any volume multiplied
Chapter 2. Source: Physiological Systems and Levels |
105 |
by the respective volume is the amount of that gas in it. Thus, the following mass equation for any specific gas holds true,
VE CE =VD CD +VACA |
(2.77) |
where the V’s represent volumes (Figure 2.44) and the C’s stand for concentrations of a trace gas within the respective volumes. Solving the equation for VD, and considering the sum of the two volumes, leads to,
VD |
=VE |
CE |
−CA |
(2.78) |
|
CD |
−CA |
||||
|
|
|
Since the atmospheric carbon dioxide content is negligible, all the CO2 expired in a breath comes essentially from the alveoli and none from the dead space, thus, CD = 0, and the equation becomes,
VD |
=VE |
CA |
−CE |
(2.79) |
|
CA |
|||||
|
|
|
because always the concentration in the expired mixture, CE, is smaller
than CA.
Hence, by measuring the CO2 partial pressure in the alveolar region, which is the same as that in arterial blood (by definition, at the outflow of an exchanger the concentration equals the concentration of the supplying side) and the carbon dioxide partial pressure in the expired air, one can determine the dead space. Remember that the partial pressure of a gas in a mixture is directly related to its concentration. Expression (2.79) is called Bohr’s Equation.
In healthy individuals, the anatomic and physiologic dead spaces are roughly equivalent, since all areas of the lung are well perfused. However, in disease states where portions of the lung are poorly perfused, the physiologic dead space may be considerably larger than the anatomic dead space. Hence, physiologic dead space is a more clinically useful concept than is anatomic dead space. The dead space is significantly augmented in emphysema and silicosis.
Christian Bohr (1855–1911), father to the Nobel laureate physicist Niels Bohr, was one of the founders of modern respiratory physiology. In his laboratory at the University of Copenhagen, students like August and Marie Krogh bloomed, heavily contributing to the field (Schmidt–Nielsen, 1984). August Krogh got the Nobel Prize for Physiology in 1920.
106 |
Understanding the Human Machine |
− Alveolar ventilation
From the functional point of view, this is the important variable, for it removes the undesirable effect of the dead space. It is defined as,
QA´=RR×(TV−VD ) |
(2.80) |
and so clearly establishing that only a fraction of the tidal volume (about 70%) is actually involved in the gas exchange. For 15 breaths/min, TV = 500 mL/breath and VD = 150 mL/breath, the alveolar ventilation is only 5.25 L/min and not the 7.5 predicted by the overall ventilation.
Numerical exercise: If you breath with shallow breaths of only 200 mL and at a high rate of 30 breaths/min, do you hypoor hyper-ventilate yourself? If you do the opposite, say at deep breaths of 600 mL/breath but at a low frequency of 10 breaths/min, do you hypoor hyperventilate yourself? Based on this information, assume you are training a group of kids in a swimming club and you want them to dive and swim underwater for the whole pool length, what type of the two previously described respirations would you recommend them to do just before diving, say for a few breaths?
Thinking exercise: Kids like to play tricks on girls when they play in a pool or, better, at the beach, as for example swimming underwater and grabbing their legs. One smarty gets hold of a long plastic tubing with a small short curve at one end in order to place it in his mouth, dive in and breathe through it while the other pipe end just vertically sticks out of the water surface, as in a submarine. Now he can swim underneath undisturbed as long as he likes while he has the heck of a time creating a nice mess among the poor defenseless scary gals above! Do you think he can really stay down there as long as he likes? Could he go as deep as he wants?
− Pressures
Three absolute pressures play a role during the respiratory act: alveolar pressure PA, pressure in the pleural cavity PPL, and the atmospheric or barometric pressure PB. With this in mind, three differences can be defined that are of daily use,
PA − PB = Ptw |
or transairway pressure |
(2.81) |
||
PPL |
− PA |
= Ptp |
or transpleural pressure |
(2.82) |
PPL |
− PB |
= Ptt |
or transthoracic pressure |
(2.83) |
From the three latter equations, it is easily seen that Ptt = Ptp + Ptw or, in words, the transthoracic pressure is the sum of the transpleural and the transairway pressures. These pressures vary over the respiratory cycle
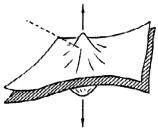
Chapter 2. Source: Physiological Systems and Levels |
107 |
Figure 2.45. GENERATION OF A SUBATMOSPHERIC PRESSURE. Two wet rubber sheet tend to stick together when one is set over the other. A virtual space or cavity is generated.
and the most important and interesting is Ptp. The pressure in the pleural cavity is always lower than the atmospheric pressure, thus, Ptp is always negative (slightly subatmospheric). Let us describe its mechanism of generation.
Two wet rubber sheets tend to stick together when one is set over the other (Figure 2.45). If we try to separate them off by pulling out perpendicularly in opposite directions, a partial vacuum volume will be created by simple forced expansion. The inner thoracic wall and the outer pulmonary wall are in apposition, filled with a small amount of fluid so that they can easily slide over each other. Due to their intrinsic elastic properties, the former tends to recoil outwardly and the latter tends to do it inwardly. The net result is a phenomenon similar to the subatmospheric pressure effect illustrated in the picture. In fact, there is no space between the two sheet or between the lungs and the thorax, thus, it is called a virtual space or cavity.
Under resting expiratory conditions, intrapleural pressure is about 2 mmHg lower than atmospheric pressure because of the basic passive mechanism depicted in Figure 2.45. Due to the action of the inspiratory muscles, the thorax expands and intrapulmonary pressure decreases. Meanwhile, the added pulling out of the chest wall decreases intrapleural pressure even more. The net result is air flowing in with a concomitant increase in intrapulmonary pressure ending up inspiration when lung recoil counteracts chest recoil. Thereafter, intrapleural pressure goes up, but always below atmospheric, because of passive exhalation (not forced expiration) due to temporary predominance of lung recoil and everything