
Understanding the Human Machine - A Primer for Bioengineering - Max E. Valentinuzzi
.pdf158 |
Understanding the Human Machine |
2.6. Endocrine System
Perhaps most of our acts and responses are driven by the internal secretions.
2.6.1. Introduction
The general and bold block diagram of Figure 2.1 (bold because it is an overt oversimplification) shows in its upper portion a modest inner rectangle, linked to the Nervous System, representing the compound to be developed in this section, the Endocrine System (ES). The task is quite daring, demanding and, at the same time, fascinatingly attractive because perhaps many of the unknowns still hidden in the ES contain the answers to the flows and ebbs of our behavioral misfortunes and states of elation. And the task is aimed at the young fresh mind, just starting the long biomedical engineering road, with a core material that has to be clearly and succinctly explained. On top of it, it must motivate.
It is a system with a high “engineering content”. In it, control is omnipotent, showing delicate high sensitivities all over, with extremely low concentrations of hormones in many cases. Its derangements lead always to unhappy endings. We will subdivide it in functional subsystems according to the currently accepted knowledge, defining functions, variables and feedback loops, always to the best of our possibilities. The following sections, (2.6.2 through 2.6.7), include respectively, the HypothalamicHypophyseal Axis (HHA), the Catecholamine System: Adrenal Medulla, the Thyroid-Parathyroid System for Calcium Regulation, the InsulinGlucagon System: Pancreas, the Renin-Angiotensin-Cardionatrine System, and the relatively recently revisited Pineal Gland and Biological Clock. In the closing remarks, we will try to pose some of the pragmatic and heuristic sides of this basically scientific specialty of physiology.
The Endocrine System controls, coordinates and regulates different functions in the organism, many times in conjunction with the Nervous System. Its actions are relatively slow to take place because the hormones — with their messages — are released into the blood stream by the internal secretion glands, as briefly described in the previous section. Hormones are highly specific and only trigger effects on well-determined targets
Chapter 2. Source: Physiological Systems and Levels |
159 |
(cells or organs). The elicited response is usually proportional to the stimulating hormonal concentration. For more details, we refer to any textbook of physiology, as for example Ganong (1981) or Guyton and Hall (1996), either in the stated editions or in any of their many others, considering always that the newer, the better.
2.6.2. Hypothalamic-Hypophyseal Axis (HHA)
The hypothalamus — a functionally ubiquitous portion of the diencephalon — is the principal center of this group for it skillfully pulls the strings to keep most of the whole system under control. It receives information from higher centers in the brain, it has vascular connections with the adenohypophysis (anterior pituitary) and it has, too, neural pathways linking it to the neurohypophysis (posterior pituitary). However, both hypophyses, while anatomically attached to each other, are embriologically different. Thus, the hypothalamic-hypophyseal axis (HHA) is, in fact, split in two, the hypothalamic-adenohypophyseal (HAH) and the hypothalamic-neurohypophyseal (HNH) axes. Each axis, in turn, gives rise to a system that projects into the whole organism. Hence, from now on we will rather speak of ‘systems’. Let us start with the first of them.
2.6.2.1. Hypothalamic-Adenohypophyseal System (HAHS)
It is characterized by a portal vascular arrangement transporting minute quantities of hypothalamic releasing and inhibiting hormones directly to their target cells in the anterior pituitary, that is, they are not diluted out in the systemic circulation. The distance they travel is very short. Specific hypothalamic hormones bind to receptors on specific anterior pituitary cells, modulating the release of the hormone they produce. Thus, some of the neurons within the hypothalamus — neurosecretory neurons
— secrete hormones that strictly control secretion of hormones from the anterior pituitary. Hans Selye and Roger Guillemin, in Montreal, put the hypothesis of hypothalamic mediators forward in the early 1950’s and that can be considered the beginning of neuroendocrinology (Guillemin, 1998).
The hypothalamic hormones are referred to as releasing hormones and inhibiting hormones, reflecting their influence on anterior pituitary hormones. In the early years of neuroendocrinology, these hormones were baptized as releasing factors, thus, TRH (thyrode releasing hormone)
160 |
Understanding the Human Machine |
was TRF (thyrode releasing factor), CRH was CRF, and so on. The hypophysis or pituitary gland (both sections), in turn, is often portrayed as the “master gland” of the body. Such praise is justified in the sense that the anterior and posterior pituitaries secrete a battery of hormones that collectively influence all cells and affect virtually all-physiologic processes.
Other secretions of the hypothalamus, still with unsettled functions, include the melanocyte-stimulating hormones (α-MSH and β-MSH) and the endorphins. In lower species, like amphibians and reptiles, the two former seem to be related to skin coloration. In mammals, however, their function remains uncertain for the time being. The latter, which are mor- phine-like substances, have analgesic effects. All hypothalamic secretions are polypeptides, proteins or glycoproteins
Exercise: Compare the hepatic portal system with the hypothalamic portal system. Try to find differences, if any. Are there other portal systems in the organism? Search in the literature.
− Thyroid
The hypothalamus secretes the thyrotropin-releasing hormone (TRH), which binds to receptors on anterior pituitary basophilic cells called thyrotrophs (or thyrotropes), stimulating them to secrete the thyroidstimulating hormone (TSH) or thyrotropin. It is a glycoprotein with a molecular weight of approximately 28,000 daltons. This pituitary hormone enters the systemic circulation and binds to their receptors on other target organs. In the case of TSH, the target organ is the thyroid gland (Figure 2.61) that, in response, produces triiodothyronine (T3) and thyroxine (T4). These two hormones affect the metabolism, growth and cell differentiation of practically all the tissues. Two negative feedback loops presumably control the blood concentration of these hormones, one detecting TSH with hypothalamic sensors and another checking T4 with pituitary sensors. Higher centers always influence the hypothalamic action, implicitly meaning that external perturbations may also have an effect.
Iodine is a raw element essential for thyroid hormone synthesis. Ingested iodine (I2) is converted to iodide (I– ) and absorbed. The minimum daily intake that will maintain normal thyroid function is 100–150 µg in the
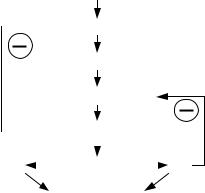
Chapter 2. Source: Physiological Systems and Levels |
161 |
adult. Deficiency of the thyroid hormones (hypothyroidism) leads to cretinism, in children, and myxedema, in the adult. Both are serious conditions. Goiter is another form of hypothyroidism triggered by low dietary content of iodine. In that case, since T3 and T4 synthesis and secretion are low, due to the feedback loop, TSH production increases to abnormally high levels and, thus, to a permanent stimulation of the gland which responds with its excessive growth (hypertrophy) in an effort to unsuccessfully make up for the deficiency. The enlargement of the thyroid gland results in bulging of the neck that may become extremely large. Occasionally, it may cause some difficulty in breathing and swallowing. This is why it is common practice to add iodide to table salt (NaCl) as a goiter prevention measure.
Conversely, excess levels in blood of T3 and T4 are generically termed hyperthyroidism. Graves’ disease is its most common form. Robert Graves discovered it in 1835. It affects approximately three out of 1,000 people and is more prevalent in women and in families with a history of the disorder. Graves' Disease is an autoimmune disorder in which an as yet unknown immunological defect results in production of autoantibodies to the TSH receptor located on the surface of thyroid cells. These antibodies bind the receptor and stimulate it to overproduce thyroid hormones. This activation is not subject to the normal regulatory negative
Hypothalamus
TRH
Adenohypophysis
|
|
|
|
TSH |
|
|
|
|
T3 |
|
|
|
|
T4 |
|||
|
|
|
|
|
|
|||
|
|
|
|
|
|
|||
|
Thyroid |
|
|
|||||
|
|
|
||||||
|
|
|
|
|
|
|
|
|
|
|
|
|
|
|
|
|
|
|
|
|
TISSUES |
|
|
|
||
|
|
|
|
|
|
|
|
|
Figure 2.61. HYPOTHALAMUS- ADENOHYPOPHYSIS-THYROID RELATIONSHIP. TRH is a hypothalamic neurosecretion carried by the portal system to the adenohypophysis where it stimulates the secretion of TSH. The latter, in turn, carried also by the circulatory stream has a specific stimulating action on the thyroid gland which, in response, produces triiodothyronine (T3) and thyroxine (T4). These two hormones affect the metabolism of practically all the tissues. Two negative feedback loops control the blood concentration of these hormones, one detecting TSH with hypothalamic sensors and another checking T4 with pituitary sensors.
162 |
Understanding the Human Machine |
feedback loop even though the blood level of TSH is lower than normal (as low as 1/100 the level of euthyroid subjects). Symptoms of Graves' disease include nervousness, irritability, weight loss, increased appetite, heat intolerance, excessive sweating, rapid pulse, diarrhea, fine tremors in fingers and warm moist skin. About 50% of patients also develop exophtalmia (bulging eyes).
Just to think about. Roger Guillemin (1998) — Nobel Prize of Physiology in 1977 — states in his touching and clear autobiographic recollections: “I consider the isolation and characterization of TRF the major event in the establishment of modern neuroendocrinology, the inflection point that separated confusion and a great deal of doubt from real knowledge. Contemporary neuroendocrinology was born of that event. Isolation of LRF [the luteinizing hormone-releasing factor, now called LRH or LHRH], somatostatin, the endorphins, others later, were all extensions of that major event — the isolation of TRF — a novel molecule in hypothalamic extracts, with hypophysiotropic activity, the first so characterized. The event was the vindication of 14 years of hard work within the paradigm of a hypothalamic neurohumoral control of adenohypophyseal secretions. From observation of what has happened in neuroendocrinology since 1969, the isolation of TRF was also the vindication of my early decision, as a physiologist, that the most heuristic event in neuroendocrinology would be the isolation and characterization of the first one (any one) of the then-hypothetical hypothalamic hypophysiotropic factors.” And he added as a foot note about the cost: “I once calculated that the first 1 mg of native, pure, ovine TRF made 1 kg of pure, native TRF, 2.5 times more expensive than a kilogram of moon rock brought back from the Apollo XI mission. Today the cost of synthetic TRF is a few cents per milligram”. After TRF, pioneering in neuroendocrinology ceased and became the harvesting of a new expanding science, because far more interesting and revolutionary observations were to follow. TRH (or TRF) was isolated in 1968 by Guillemin and collaborators in the Department of Physiology of Baylor College of Medicine, in Houston, Texas.
− Adrenal Cortex
The adrenal glands, which are also called suprarenal glands, are small, triangular pinkish structures located on top of both kidneys. This gland
— essential for life — is made of two parts: the outer region (or the adrenal cortex) and the inner region (or the adrenal medulla). They are quite different from a developmental point of view as they are also functionally. We will consider now the former.
The hypothalamus produces corticotropin-releasing hormone (CRH), which stimulates the adenohypophysis (or anterior pituitary gland) while the latter, in response, produces adrenocorticotropin hormone (ACTH), which in turn stimulates the adrenal cortex to secrete a set of hormones:
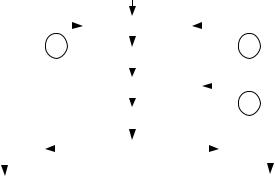
Chapter 2. Source: Physiological Systems and Levels |
163 |
aldosterone (a mineralocorticoid) and glucocorticoids (corticosterone and cortisol). Figure 2.62 briefly summarizes the relationships, including three negative feedback regulatory loops. The hypothalamus senses the blood levels of ACTH and of cortisol so that an increase in them elicits a decrease in CRH and in ACTH triggering in this way an opposite compensatory change. Similarly, the adenohypophysis constantly measures the concentration of glucocorticoids (mainly cortisol) to decrease or increase its ACTH production and, hence, make up for any initial increase or decrease in cortisol. Aldosterone activates the retention of sodium and water playing, as a consequence, a role in the electrolytic balance and in blood pressure regulation. Glucocorticoids have an effect on the intermediary metabolism of carbohydrates, proteins and lipids in different tissues. They also suppress inflammatory reactions in the body and affect the immune system. Besides, the adrenal cortex secretes androgenic steroids (androgen hormones). These hormones have minimal effect on the development of male characteristics.
|
|
|
|
|
|
|
HYPOTHALAMUS |
|
|
|
|
|
|
|
||
|
|
|
|
|
|
|
|
|
|
|
|
|
|
|||
|
|
|
|
|
|
|
|
|
|
|
|
|
|
|
|
|
|
|
|
|
|
|
|
|
CRH |
|
|
|
|
|
|
|
|
|
|
|
|
|
|
|
|
|
|
|
|
|
|
|
||
|
|
|
|
|
|
|
|
|
|
|
|
|
|
|
|
|
|
|
|
|
|
|
|
ADENOHYPOPHYSIS |
|
|
|
|
|
|
|
||
|
|
|
|
|
|
|
|
|
|
|
|
|
|
|||
|
|
|
|
|
|
|
|
|
|
|
|
|
|
|
|
|
|
|
|
|
|
|
|
|
ACTH |
|
|
|
|
|
|
|
|
|
|
|
|
|
|
|
|
|
|
|
|
|
|
|
||
ALDOSTERONE |
|
|
|
|
|
|
|
|
|
|
|
|||||
|
|
|
|
|
|
|
|
|
|
|
|
|
||||
|
|
|
|
|
|
|
|
|
|
|
|
|
||||
|
|
|
|
|
|
|
|
|
|
|
|
|
||||
|
|
|
ADRENAL CORTEX |
|
|
GLUCOCORTICOIDS |
||||||||||
|
|
|
|
|
||||||||||||
|
|
|
|
|
|
|
|
|
|
|
|
|
|
|
|
|
SODIUM AND WATER |
|
|
|
|
|
|
|
TISSUES |
||||||||
RETENTION |
|
|
|
|
|
|
|
|||||||||
|
|
|
|
|
|
|
|
|
|
|
Figure 2.62. HYPOTHALAMUS-ADENOHYPOPHYSIS-ADRENAL CORTEX SYSTEM. The relationship is similar to that of the thyroid gland. Higher nervous centers always influence the hypothalamus. Corticotropin Releasing Hormone (CRH) is another hypothalamic neurosecretion carried by the portal system blood to stimulate the adenohypophysis, which, in response, produces adrenocorticotropic hormone (ACTH). The latter, in turn, via the general circulation, reaches its target organ — the adrenal cortex
— to elicit secretion of two types of hormones: a mineralocorticoid (aldosterone), and glucocorticoids (corticosterone and cortisol).
164 |
Understanding the Human Machine |
Another recent historical tip: Hans Selye (Guillemin, 1998), through his stress concept as related to the adrenal cortex, had a major stimulating role in orienting the early efforts in neuroendocrinology toward the study of the hypothalamus-pituitary ACTH-adrenal cortex functional relationship. According to Guillemin’s comments, strangely enough, and unwittingly on Selye’s part, this is probably about the worst thing that happened to nascent neuroendocrinology. The search for CRF was to prove so complex and baffling that it was not completed until 1981 through the elegant work led by Wylie Vale, one of Guillemin’s students and collaborators. They should have better put the effort on some other hypothalamic secretion. Perseverance and vision are essential characteristics of an investigator.
There are important pathologies associated with adrenal cortex malfunction: Cushing’s syndrome (excess production of glucocorticoids), Conn’s syndrome (excess production of aldosterone), and Addison’s disease (deficiency of corticoids).
Cushing's syndrome (named after Harvey Williams Cushing, American surgeon, 1869–1939) occurs when the body's tissues are exposed to excessive levels of cortisol for long periods of time. Many people suffer the symptoms of Cushing's syndrome because they take glucocorticoid hormones such as prednisone for asthma, rheumatoid arthritis, lupus and other inflammatory diseases (allergies, for example), or for immunosuppression after transplantation. Because of the negative feedback loop, the higher than normal blood concentration of these hormones inhibits production of the physiological hormones and the gland may stop secretion even after treatment is stopped. It is relatively rare and most commonly affects adults’ aged 20 to 50. An estimated 10 to 15 of every million people are affected each year.
The second condition affecting the adrenal gland is Conn’s syndrome, also called primary aldosteronism (named after Jerome W. Conn, an American internist, 1907–1981). It is due to the presence of an adrenal tumor, usually benign. The excess aldosterone secreted in this condition increases sodium reabsorption and potassium loss by the kidneys and results in electrolyte maladjustments (Conn, 1955). Risk factors are being female and being between 30 and 50 years old. The incidence is 2 out of 100,000 people; fewer than 10 children have been reported in the literature with Conn’s syndrome. Secondary aldosteronism originates in other causes, not directly related to the adrenal cortex.
The third significant pathology of the adrenal cortex is Addison’s disease (named after Thomas Addison, English physician, 1793–1860). This

Chapter 2. Source: Physiological Systems and Levels |
165 |
primary adrenal failure may be the result of congenital or acquired lesions. In the early part of the XXth century, tuberculosis was the most common cause. Other infrequent conditions may lead to adrenal cortex damage and insufficiency. However, in recent years it appeared that most cases represent the outcome of an autoimmune process.
For more details about these three pathologies check the website http://www.mc.vanderbilt.edu/peds/pidl/endocr/index.htm, copyright 1998, Vanderbilt University Medical Center, http://www.mc.vanderbilt.edu/cgibin/mail?webmasteror Kaplan (1982) or Hughes (1982). The textbooks of physiology usually give some information on these subjects.
|
External Factors |
|
|
|
HYPOTHALAMUS |
|
|
LHRH |
FSHRH? |
PIH |
PRH |
|
|
AP |
|
LH |
|
FSH |
LTH |
GONADS
SEX HORMONES
ENDOCRINE & REPRODUCTIVE FUNCTIONS
Figure 2.63. HYPOTHALAMIC-ADENOHYPOPHYSEAL-GONADS SYSTEM. Four releasing hormones are produced by the hypothalamus (one of them is still uncertain, as indicated by the interrogation mark). Three are stimulating hormones (thus, a plus sign is shown) and one has an inhibiting effect (negative sign), respectively, on the adenohypophysis or anterior pituitary gland (AP). In response, the latter produces LH, FSH and LTH, which, in turn, having the gonads as target organs, mediate the liberation of the sex hormones to act on the reproductive, developmental and other behavioral functions. The probable negative feedback loops are also shown.
166 |
Understanding the Human Machine |
Thomas Addison committed suicide. He was a sensitive, shy, unhappy man who became increasingly despondent during the last two years of his life. There were indications that he suffered from some mental illness (Cirillo, 1985). Science, medicine, is not always associated with happy events. Human beings are the principal actors and this is something a future bioengineer must learn from the very beginning: to deal with men and women and to accept them as they are.
− The Gonads: Sex Glands
This system follows a scheme similar to the two previously described; perhaps, it is somewhat more complex than them. Figure 2.63 summarizes the hypothalamic-adenohypophysis-gonads relationships. The hypothalamus produces the releasing hormones: luteinizing hormonereleasing hormone (LHRH), follicle-stimulating-hormone-releasing hormone (FSHRH) — still not fully demonstrated — prolactin inhibiting hormone (PIH), and prolactin releasing hormone (PRH). The first one, that is LHRH, stimulates the production of the luteinizing hormone (LH) and of the follicle-stimulating hormone (FSH) from the adenohypophysis or anterior pituitary. This peptide, first characterized and synthesized by Roger Guillemin and Andrew Schally (both shared the 1977 Nobel prize for this labor), has been also called the gonadotropic hormone-releasing hormone, or GnRH (Knobil, 1999). The second, FSHRH, if true, would stimulate the secretion of FSH. In turn, PIH and PRH have opposite actions on the anterior pituitary, the former inhibiting the secretion of luteotropic hormone, LTH, also called prolactin, and the latter stimulating its secretion. Thus, the three hormones from the adenohypophysis are LH, FSH and LTH, with direct actions on the gonads as target organs that, in the end, release the sex hormones. There is evidence of the existence of negative feedback loops to control these hormonal blood levels. In women, the gonadotropins (collective name of the anterior pituitary hormones aimed at the gonads) have effects on the ovaries (the female gonads, as opposed to the testicles, the male gonads): FSH stimulates follicular growth (thus, it is a trophic hormone), while LH stimulates ovulation and luteinization of the corpus luteum. Luteinization is the process by which a postovulatory ovarian follicle transforms into a corpus luteum through vascularization, cell hypertrophy, and lipid accumulation, the latter giving the yellow color indicated by the term (luteus, yellow). Both, follicle and corpus luteum, secrete estrogens (estradiol,
Chapter 2. Source: Physiological Systems and Levels |
167 |
estrone and estriol) while the corpus luteum secretes progesterone. All these are termed the female sex hormones, responsible for uterine endometrial modifications, development of the genitalia and secondary female characteristics. Prolactin causes the postpartum milk secretion from the breasts after estrogen and progesterone priming. Besides, prolactin inhibits the effects of gonadotropins, possibly through an action on the ovaries via negative feedback because it stimulates progesterone production. Surprisingly, it was found that TRH also stimulates the secretion of LTH (Guillemin, 1976).
A fascinating phenomenon is the pulsatile nature of GnRH secretion — demonstrated in all vertebrates studied in this regard — that is in turn responsible for LH pulses with a frequency of approximately one per hour. Moreover, it has also been shown that each LH pulse is associated with electrical activity at the mediobasal hypothalamus (Knobil, 1999).
The central event of the female cycle — ovulation — is initiated by a bolus of LH from the pituitary gland. Such surge is superimposed upon, or perhaps temporarily replaces, the pulsatile pattern of LH secretion. Interestingly enough, the pacemaker for this phenomenon is not the hypothalamus but the ovary itself, which, with a preovulatory estradiol rise, acts on the hypothalamic-hypophyseal axis to start the LH surge. It means, then, that estradiol has negative and positive feedback actions (fall in LH and FSH plasma levels and preovulatory surge), both occurring at the adenohypophysis. How this can happen still remains unknown (Knobil, 1999).
In man, LHRH is the only hypophyseal hormone with definite known stimulating action on FSH and LH, for the existence of the other three is still uncertain. FSH acts on the testicular seminiferous tubules to control spermatogenesis. These tubules would produce inhibin, which acting on the adenohypophysis, would inhibit FSH production and, thus, indicating a regulatory loop for sperm production. LH, in turn, has an action on the Leydig’s cells in the testicles, main source of testosterone and the essential male sex hormone with androgenic and general anabolic effects. Besides, testosterone stimulates spermatogenesis from the seminiferous tubules and feeds back to the hypothalamus to regulate LHRH release, in another negative control loop.