
Understanding the Human Machine - A Primer for Bioengineering - Max E. Valentinuzzi
.pdf178 |
Understanding the Human Machine |
insulin. Juvenile diabetes is insulin dependent as opposed to diabetes of the elder, which can be taken care of by just a well-adjusted diet. It is a disorder characterized by hyperglycemia or elevated blood glucose (blood sugar). Our bodies function best at a certain level of sugar in the bloodstream (80 to 100 V%). If the amount of sugar in our blood runs too high or too low, then we typically feel bad. Diabetes is the name of the condition where the blood sugar level consistently runs too high. Diabetes is the most common endocrine disorder. Sixteen million Americans have diabetes, yet many are not aware of it. Diabetes has potential long-term complications that can affect the kidneys, eyes, heart, blood vessels and nerves. The bibliography is enormous and growing because it is a field of basic and clinical research. The INTERNET sites are countless. It is a very big topic, indeed.
There are two different types of diabetes, which are similar in their elevated blood sugar, but different in many other ways: Type 1 diabetes and Type 2 diabetes. The former is due to insulin deficiency while the latter stems in insulin resistance. Insulin deficiency means there is not enough insulin being made by the pancreas due to a malfunction of the beta cells. Insulin resistance occurs when there is plenty of insulin made by the pancreas but the cells of the body (the ultimate users) are resistant to its action, which results in the blood sugar being too high.
Glucose is absorbed from the intestines into the bloodstream after a meal. Insulin is then secreted by the pancreas in response to this detected increase in blood sugar. Most cells of the body have insulin receptors that bind the insulin, which is in the circulation. When a cell has insulin attached to its surface, the cell activates other receptors designed to absorb glucose from the blood stream into the inside of the cell. Without insulin, a person can eat lots of food and actually be in a state of starvation since many of his/her cells cannot access the calories contained in the glucose. It is as having a refrigerator full of food but closed with a padlock. This is why Type 1 diabetics who do not produce insulin can become very ill without insulin shots. Insulin is an indispensable hormone. Those who develop a deficiency of insulin must have it replaced via shots or pumps. More commonly, people will develop insulin resistance (Type 2 Diabetes) rather than a true deficiency of insulin. In this case, the levels of insulin in the blood are similar or even a little higher than in normal, non-
Chapter 2. Source: Physiological Systems and Levels |
179 |
diabetic individuals. However, many cells of Type 2 diabetics respond sluggishly to the insulin they make and, therefore, their cells cannot absorb the sugar molecules well. This leads to blood sugar levels that run higher than normal. Occasionally Type 2 diabetics will need insulin shots but most of the time other methods of treatment will work.
A superb and dramatic piece of history. In 1920, a Canadian surgeon, Frederick G. Banting (1891–1941) visited the University of Toronto to see the newly appointed head of the department of physiology, John J.R. Macleod (1876–1935). The latter had studied glucose metabolism and diabetes, and Banting had a new idea on how to find not only the cause but a treatment for the so-called “sugar disease.” Late in the nineteenth century, scientists had realized there was a connection between the pancreas and diabetes. The connection was further narrowed down to the islets of Langerhans. From 1910 to 1920, Oscar Minkowski and others tried unsuccessfully to find and extract the active ingredient from the islets. While reading a paper on the subject in 1920, Banting had an inspiration. He realized that the pancreas' digestive juice was destroying the islets of Langerhans hormone before it could be isolated. If he could stop the pancreas from working, but keep the islets of Langerhans going, he should be able to find the stuff. He presented this idea to Macleod, who at first scoffed at it but finally gave him lab space, 10 experimental dogs, and a medical student assistant, Charles Best (1899–1978). In May, 1921, as Macleod took off for a holiday in his native Scotland, Banting and Best began their experiments. By August, they had the first conclusive results: When they gave the material extracted from the islets (called “insulin,” from the Latin for “island”) to diabetic dogs, their high blood sugars were lowered. Macleod, back from holiday, was still skeptical of the results and asked them to repeat the experiment several more times. They did, finding the results the same, but with problems due to the varying purity of their insulin extract. Macleod assigned a chemist, James Bertram Collip (1892–1965) to the group to help with the purification. Within six weeks, the purified insulin was tested on a 14-year-old boy dying of diabetes. Insulin lowered his blood sugar and cleared his urine. Banting and Best published the first paper on their discovery a month later, in February, 1922. In 1923, the Nobel Prize was awarded to Banting and Macleod for the discovery, and each shared their portion of the prize money with the other researchers on the project, Best and Collip. Banting initially threatened to refuse the award because he felt Charles Best's work as research assistant had been vital to the project and that he should be included in the honor.
The discovery of insulin was one of the most revolutionary moments in medicine. Though it took some time to work out proper dosages and to develop manufacturing processes to make enough insulin of consistent strength and purity, the introduction of insulin seemed literally like a miracle. One year the disease was an automatic death sentence; the next, people had hopes of living full and productive lives even with the disease. Estimates show there are more than 15 million diabetics living today who would have died at an early age without insulin. Animal experimentation was essential to obtain this knowledge.
180 |
Understanding the Human Machine |
The first successful insulin preparations came from cows and later on from pigs. The pancreatic islets and the insulin protein contained within them were isolated from animals slaughtered for food in a similar but more complex fashion than was used by our doctor and medical student duo. The bovine and porcine insulin were purified, bottled, and sold. Bovine and porcine insulin worked very well for the vast majority of patients, but some could develop an allergy or other types of reactions to the foreign protein (a protein which is not native to humans). In the 1980's, biotechnology had advanced to the point where we could make human insulin. The advantage would be that human insulin would have a much lower chance of inducing a reaction because it is not a foreign protein (all humans have the exact same insulin). The technology, which made this approach possible, was the development of recombinant DNA techniques. In simple terms, the human gene, which codes for the insulin protein was cloned and then put inside of bacteria (Escherichia coli). A number of tricks were performed on this gene to make the bacteria want to use it to constantly make insulin. Big vats of bacteria now make tons of human insulin. From this, pharmaceutical companies can isolate pure human insulin.
Summarizing: The purpose of insulin is to regulate blood glucose. It forces many cells of the body to absorb and use glucose thereby decreasing blood sugar levels. Insulin is secreted in response to high blood glucose. Conversely, low blood glucose inhibits its secretion from the beta pancreatic cells. There is a type of tumor called insulinoma, which secretes large amounts of insulin, and thus, it produces severe hypoglycemia. Glucagon, instead, has actions opposite of insulin. It assists insulin in the regulation of blood glucose because it forces many cells of the body to release or to produce glucose. It is secreted in response to low blood glucose while its secretion is inhibited by high blood glucose. Deficiency may lead to hypoglycemia, but not always. Tumors called glucagonomas cause hyperglycemia.
Many mathematical models have been devised to predict responses to given stimulations and, thus, to better dose insulin. The group of Claudio Cobelli, in Padova, Italy, has extensive contributions to the field. Another area of development refers to automatic infusion pumps, either for the hospital environment or for ambulatory patients.
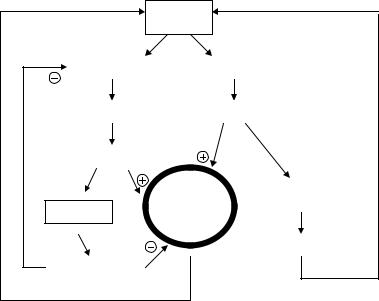
Chapter 2. Source: Physiological Systems and Levels |
181 |
2.6.6. The Renin-Angiotensin-Cardionatrine System
Figure 2.70 outlines in a very simplified manner an accessory system to regulate blood pressure and body fluids. Many concepts are relatively recent and it still poses questions highly attractive to the physiologist, clinician and bioengineer.
Let us consider blood pressure BP as the central variable assuming a momentary decrease in its value. As a consequence, there will be less stretch of the juxtaglomerular apparatuses in the kidneys, located at the afferent arterioles (see Section 2.4). Thus, the hormone called renin will be secreted which, as it enters into the circulation, triggers a complex chain of events that end up with the formation of the polypeptide angiotensin II, in itself a potent vasopressor. This simple negative feedback loop tends to compensate for the initial decrease in BP; however, that is
|
Kidneys |
|
Na Reabsorption |
|
Renin |
Water Retention |
Angiotensin II |
|
ECV increase |
|
|
|
Blood |
ADRENAL |
ATRIA |
Pressure |
CORTEX |
|
|
|
|
|
ALDOSTERONE |
CARDIONATRINE |
|
|
Figure 2.70. RENIN-ANGIOTENSIN-CARDIONATRINE SYSTEM. Angiotensin and cardionatrine have opposite effects. Negative and positive signs indicate activation or inhibition, respectively. It is a slow acting system. Arterial blood pressure is presented as the regulated variable that acts on the juxtaglomerulus apparatuses of the kidneys: when it goes down, they secrete renin. Production of angiotensin II occurs in the blood stream, after a complex chain of biochemical reactions. See text.
182 |
Understanding the Human Machine |
not the main pathway, for angiotensin II stimulates the adrenal cortex to secrete aldosterone. The latter acts on the kidneys enhancing sodium reabsorption, water retention and its consequent increase in extracellular volume ECV, which has also a compensatory effect on BP. This is a second negative feedback loop. The atria, in turn, have stretch volume receptors that activate the secretion of the atrial natriuretic peptide or cardionatrine. Surprisingly, the heart appears also as an endocrine organ. This relatively new substance stimulates the kidneys to excrete sodium (natriuresis), opposite to sodium reabsorption. Besides, it has a vasodilating effect, thus, tending to lower BP (Wardener and Clarkson, 1985).
Natriuretic peptides are a group of naturally occurring substances that act in the body to oppose the activity of the renin-angiotensin system. Heart failure is a leading cause of morbidity and mortality. In the United States, there are more than 5 million patients with heart failure and over 500,000 newly diagnosed cases each year. Sodium and water retention play a significant role in this disease. There are three major natriuretic peptides: atrial natriuretic peptide (ANP), synthesized in the atria; brain natriuretic peptide (BNP), synthesized in the cardiac ventricles; and C-type natriuretic peptide (CNP), synthesized in the brain. Both ANP and BNP are released in response to atrial and ventricular stretch, respectively, and cause vasorelaxation, inhibition of aldosterone secretion in the adrenal cortex, and inhibition of renin secretion in the kidneys. Both ANP and BNP cause natriuresis and a reduction in intravascular volume, effects amplified by antagonism of antidiuretic hormone (ADH). Increased blood levels of natriuretic peptides have been found in certain disease states, suggesting a role in the pathophysiology of them, including congestive heart failure (CHF), systemic hypertension, and acute myocardial infarction. For more details, see James A Hill (hillja@medmac.ufl.edu) and B.J. Strickland (stricbj@shands.ufl.edu). Produced by the Office of Medical Informatics, University of Florida College of Medicine http://www.medinfo.ufl.edu/cme/grounds/hill/intro.html.
De Bold and co-workers, in 1981, first demonstrated that atrial extracts contain a substance that produced natriuresis and diuresis. Soon after that, in 1983-4, the ANP molecule was purified and sequenced. Some years later two other major new peptides of this family were discovered. BNP was originally isolated from porcine brain in 1988.
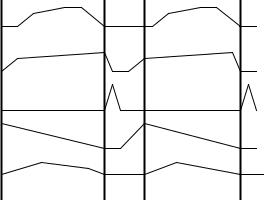
Chapter 2. Source: Physiological Systems and Levels |
183 |
2.6.7. The Pineal Gland and the Biological Clock
by Veronica S. Valentinuzzi and Max E. Valentinuzzi
Time permeates the Universe and timing permeates animal and human life.
Every day our serum cortisol level peaks at approximately our wake-up time, growth hormone reaches its maximum blood level at the beginning of our sleep phase, prolactin does so soon after, while thyrotropin hormone peaks in the last part of our sleep phase. In the same way, mostly every hypothalamic, hypophyseal and hypophysis-dependent hormones show a fixed temporal pattern with peaks and troughs everyday at the same time and with a stable phase-relationship between them as well as with other types of physiological and behavioral rhythmic variables. For example, blood pressure and core body temperature, subjective alertness and potassium excretion, also vary predictably day by day, with values raising during the day and falling during sleep. Figure 2.71 illustrates the
6 |
24 |
6 |
24 |
|
HRS |
|
|
Figure 2.71. PHYSIOLOGICAL CIRCADIAN RHYTHMS of a person sleeping 6 hours per day, from midnight to 6:00 A.M. From top to bottom: Alertness (in arbitrary subjective units); Body Temperature (from about 36 to 37°C); Growth Hormone and Cortisol (relative blood levels); and Potassium Excretion (in flow units), during two day-night cycles.
184 |
Understanding the Human Machine |
approximate phase relationship among alertness, body temperature (with a range of about 1°C), growth hormone and cortisol blood levels (shown in relative terms), and potassium excretion through the kidneys in flow units, during two 24 hr cycles. This temporal organization is achieved due to an internal clock with a free-running period of about 25 hours that, in normal conditions, is entrained (i.e., it determines or modifies the phase or period) to the 24-hour solar period resulting from the Earth's rotation.
The period and phases of these physiological parameters must be synchronized with respect to each other as well as with the environmental cycles in order for them to have an optimal effect on body organs and systems. Regular alteration of peaks and troughs is now known to be essential for normal function. Evidence showing that pathological states are associated with abnormal rhythms has accumulated, and there is now substantial support for the hypothesis that disorders of temporal organization may be involved in the etiology of various diseases.
Any biological process that varies predictably with a period in the range of 20–28 hours may be described as a circadian rhythm (circa, about; dies, day). The current theory is that multiple independent oscillators exist in the body and that their periods are locked together with different phases by a pacemaker system resulting in coherent circadian rhythms. This circadian pacemaker system has three major components:
1)the biological clock, generator of circadian rhythms;
2)neural inputs, or entrainers, into the clock from other parts of the brain; and
3)output from the clock to target tissues which couple their internal rhythmicity to that of the clock.
The result of all this is a stable, organized and predictable expression of circadian rhythms. In mammals, the master biological clock is located in the anterior hypothalamus at each side of the third ventricle on top of the optic chiasm, the suprachiasmatic nucleus (SCN). The cells that compose these nucleuses have the capacity to generate rhythmicity in diverse cell parameters due to a mechanism of negative feedback loops of gene expression and protein synthesis. Gene expression is the process by which the information contained in genes is used to produce proteins or functional RNA (ribonucleic acid) molecules.
Chapter 2. Source: Physiological Systems and Levels |
185 |
It is of interest to point out that the molecular mechanism of the clock is essentially the same in every living species, from the fruit fly to human beings. In evolutionary terms, this reveals the importance of a time keeping mechanism for the survival of an organism to an environment that is also essentially rhythmic (the regular alternation of day and night and all the other resulting environmental rhythms such as social cycles, food availability cycles, temperature cycles and the like).
Circadian rhythms are entrained by external factors termed zeitgebers (from German, zeit, time; geber, one that gives) leading to a synchronization or temporal adaptation of our internal clocks with the environment. The light/dark cycle seems to be the most important factor in synchronization of the endogenous circadian rhythms in animals and humans. The importance of this zeitgeber is reflected in the neural connections between the retina (photosensitive structure of the eye) and the SCN, the retinohypotalamic tract (RHT), as the most obvious afferent connections of the circadian system. Light reaching the eyes every day is transduced into a neural signal and this information travels through the RHT reaching the biological clock. Let us underline that this transduction is done by photoreceptors that are independent from the traditional visual photoreceptors (cones and rods).
A brand-new class of light-responsive cells in the mammalian retina was discovered that connect directly to the brain's clock. First, researchers found a pigment called melanopsin in a small subset of retinal ganglion cells (RGCs) in the eyes of rats. Most RGCs do not respond to light, but it turned out that the melanopsin-containing ones do, making them a brand-new class of previously unknown light-responsive retinal cells. Researchers traced their connections and found that they hook up directly to the suprachiasmatic nucleus. See, for example, D. M. Berson et al. (2002), Phototransduction by retinal ganglion cells that set the circadian clock, Science, 295:1070. Several other issues of the same journal during the year 2002 published specific articles on melanopsin, showing the interest attracted by this subject.
With input from the environment, such as the light/dark cycle, the endogenous period is entrained to 24 hours. If the external stimulus is artificially removed by creating a “constant” condition, the organism would continue to show circadian rhythms, but it would have a free-running period consistent with its own internal clock; in humans this is approximately 24.2 to 24.9 hours. Finally, the SCN conveys the endogenous temporal information (in free-running conditions) or the synchronized
186 |
Understanding the Human Machine |
temporal information (when in a cycling environment), to the rest of the organism through many neural and neurohormonal paths that guarantee adequate arrival of rhythmic information to brain structures and body organs. A hormonal path could be the rhythmic synthesis in the SCN and rhythmic local secretion of hormones directly into the cerebral spinal fluid as well as in the extra-cellular space. For example, vasopressin is liberated by the SCN only during the light phase. The SCN also sends many neural connections to different brain areas. Most of them terminate directly within the hypothalamus and a few others reach other more distant brain structures. The neurotransmitters secreted rhythmically in these nerve endings may be important time markers for the different brain structures they innervate, including the hypothalamic nucleus that control the endocrine system.
Finally, another path for conveying temporal information is through a relatively complex connection of the SCN with the pineal gland. Under the SCN’s command, the pineal’s main product, melatonin (N-acetyl-5- methoxytryptamine), is secreted rhythmically in the systemic circulation. The abundance of melatonin-binding sites in several brain areas suggests that this hormone is an important coupler of circadian rhythms and endocrine functions. Although many considered the pineal gland a functional vestige just three decades ago, relatively recent research has clearly established it as an integral and important component of the neuroendocrine system. The pineal gland and its hormonal products have been functionally related to virtually every endocrine gland in the organism.
In 1917, Carey Pratt McCord and Floyd Pierpont Allen, at Johns Hopkins University, demonstrated that when the pineal gland was crushed and added to water, tadpoles released in the water would lighten significantly in color, a phenomenon that defied explanation at the time. The pigment of the melanophores (surface pigmentation cells), would concentrate around the nucleus, thus lightening the color of the amphibians' skin. Since this pigment was known as melanine, the unkown substance was called melatonin (melanin + tonus). Melatonin was isolated and its chemical structure determined in 1958 by Aaron B. Lerner, at Yale University. One of the first experiments were to test if this hormone would lighten the skin of mammals as it did in amphibians. It was shown that skin pigmentation in mammals was not altered by this hormone.
The human pineal gland is a very small structure attached by a stalk to the posterodorsal aspect of the diencephalon (see Section 7). Melatonin levels are low during the day and high during the night and this rhythm is
Chapter 2. Source: Physiological Systems and Levels |
187 |
dependent on the light portion of the light/dark cycle. Exposure to bright light (2500 lux, but not to the normal room light of about 250 lux) when melatonin levels are highest, quickly causes suppressing of further melatonin production. In addition, phase shifts in the light-dark cycle, as in the case of individuals who take a transmeridian flight, produce phase shifts in melatonin rhythm that take a few days to re-entrain to the postflight light-dark cycle. Usually, it takes one day per out-of-phase hour to resynchronize, that is, if the passenger travels to a place where the time difference with his/her original place is, say, 5 hours, he/she would need 5 days to readapt to the new environment. Melatonin cycles in old individuals seem to adjust more slowly than in younger subjects. Since readjustment of the melatonin periodicity requires several days, a number of authors feel that jet-lag may, in part, be a manifestation of the lack of reentrainment of the melatonin cycle.
The RHT and its connection to the clock play a critical role in entrainment of this melatonin circadian rhythm. This is accomplished via a long and circuitous multi-synaptic pathway by which light information that reaches the SCN through the RHT ends up reaching the pineal, leading to entrainment. In some blind human subjects, those that have retinal destruction including the melanopsin-containing RGCs, the melatonin rhythm free-runs with a period of 24.7 hours. Under such conditions, peak serum melatonin levels may be achieved at any time during the daily light or dark period.
The production of melatonin within the pinealocytes (the secretory unit of the pineal) requires the uptake of the amino acid tryptophan from the circulation, which, in turn, is obtained mainly from ingested proteins. This amino acid is converted through a series of steps, first to serotonin and then to melatonin. Two of the enzymes essential for this conversion, hydroxyindol-O-methyltransferase (HIOMT) and N-acetylserotonin (NAT), show circadian rhythmicity in their concentration and activity level. Assays that quantify NAT and HIOMT activity are often used in legal medicine to determine the approximate time of death. The activity of these two enzymes at the time of autopsy is higher in the pineal gland of humans who died during the night than in those who succumbed during the day.