
- •Preface
- •Acronyms
- •Introduction
- •Background and objectives
- •Content, format and presentation
- •Radioactive waste management in context
- •Waste sources and classification
- •Introduction
- •Radioactive waste
- •Waste classification
- •Origins of radioactive waste
- •Nuclear fuel cycle
- •Mining
- •Fuel production
- •Reactor operation
- •Reprocessing
- •Reactor decommissioning
- •Medicine, industry and research
- •Medicine
- •Industry
- •Research
- •Military wastes
- •Conditioning of radioactive wastes
- •Treatment
- •Compaction
- •Incineration
- •Conditioning
- •Cementation
- •Bituminisation
- •Resin
- •Vitrification
- •Spent fuel
- •Process qualification/product quality
- •Volumes of waste
- •Inventories
- •Inventory types
- •Types of data recorded
- •Radiological data
- •Chemical data
- •Physical data
- •Secondary data
- •Radionuclides occurring in the nuclear fuel cycle
- •Simplifying the number of waste types
- •Radionuclide inventory priorities
- •Material priorities
- •Inventory evolution
- •Assumptions
- •Errors
- •Uncertainties
- •Conclusions
- •Acknowledgements
- •References
- •Development of geological disposal concepts
- •Introduction
- •Historical evolution of geological disposal concepts
- •Geological disposal
- •Definitions and comparison with near-surface disposal
- •Development of geological disposal concepts
- •Roles of the geosphere in disposal options
- •Physical stability
- •Hydrogeology
- •Geochemistry
- •Overview
- •Alternatives to geological disposal
- •Introduction
- •Politically blocked options: sub-seabed and Antarctic icecap disposal
- •Sea dumping and sub-seabed disposal
- •Antarctic icesheet disposal
- •Technically impractical options; partitioning and transmutation, space disposal and icesheet disposal
- •Partitioning and Transmutation
- •Space disposal
- •Icesheets and permafrost
- •Non-options; long-term surface storage
- •Alternatives to conventional repositories
- •Introduction
- •Alternative geological disposal concepts
- •Utilising existing underground facilities
- •Extended storage options (CARE)
- •Injection into deep aquifers and caverns
- •Deep boreholes
- •Rock melting
- •The international option: technical aspects
- •Alternative concepts: fitting the management option to future boundary conditions
- •Conclusions
- •References
- •Site selection and characterisation
- •Introduction
- •Prescriptive/geologically led
- •Sophisticated/advocacy led
- •Pragmatic/technically led
- •Centralised/geologically led
- •Conclusions to be drawn
- •Lessons to be learned (see Table 4.2)
- •Site characterisation
- •Can we define the natural environment sufficiently thoroughly?
- •Sedimentary environments
- •Hydrogeology
- •The regional hydrogeological model
- •More local hydrogeological model(s)
- •Crystalline rock environments
- •Lithology and structure
- •Hydrogeology
- •Hydrogeochemistry
- •Any geological environment
- •References
- •Repository design
- •Introduction: general framework of the design process
- •Identification of design requirements/constraints
- •Concept development
- •Major components of the disposal system and safety functions
- •A structured approach for concept development
- •Detailed design/specifications of subsystems
- •Near-field processes and design issues
- •Design approach and methodologies
- •Design confirmation and demonstration
- •Interaction with PA/SA
- •Demonstration and QA
- •Repository management
- •Future perspectives
- •References
- •Assessment of the safety and performance of a radioactive waste repository
- •Introduction
- •The role of SA and the safety case in decision-making
- •SA tasks
- •System description
- •Identification of scenarios and cases for analysis
- •Consequence analysis
- •Timescales for evaluation
- •Constructing and presenting a safety case
- •References
- •Repository implementation
- •Legal and regulatory framework; organisational structures
- •Waste management strategies
- •The need for a clear policy and strategy
- •Timetables vary widely
- •Activities in development of a geological repository
- •Concept development
- •Siting
- •Repository design
- •Licensing
- •Construction
- •Operation
- •Monitoring
- •Research and development
- •The staging process
- •Attributes of adaptive staging
- •The decision-making process
- •Status of geological disposal programmes
- •Overview
- •Status of geological disposal projects in selected countries
- •International repositories
- •Costs and financing
- •Cost estimates
- •Financing
- •Conclusions
- •Acknowledgements
- •References
- •Research and development infrastructure
- •Introduction: Management of research and development
- •Drivers for research and development
- •Organisation of R&D
- •R&D in specialised (nuclear) facilities
- •Introduction
- •Inventory
- •Release of radionuclides from waste forms
- •Solubility and sorption
- •Waste form dissolution
- •Colloids
- •Organic degradation products
- •Gas generation
- •Conventional R&D
- •Engineered barriers
- •Corrosion
- •Buffer and backfill materials
- •Container fabrication
- •Natural barriers
- •Geochemistry and groundwater flow
- •Gas transport and two-phase flow
- •Biosphere
- •Radionuclide concentration and dispersion in the biosphere
- •Climate change
- •Landscape change
- •Underground rock laboratories
- •URLs in sediments
- •Nature’s laboratories: studies of the natural environment
- •General
- •Corrosion
- •Cement
- •Clay materials
- •Degradation of organic materials
- •Glass corrosion
- •Radionuclide migration
- •Model and database development
- •Conclusions
- •References
- •Building confidence in the safe disposal of radioactive waste
- •Growing nuclear concerns
- •Communication systems in waste management programmes
- •The Swiss programme
- •The Japanese programme
- •Examples of communication styles in other countries
- •Finland
- •Sweden
- •France
- •United Kingdom
- •Comparisons between communication styles in Finland, France, Sweden and the United Kingdom
- •Lessons for the future
- •What is the way forward?
- •Acknowledgements
- •References
- •A look to the future
- •Introduction
- •Current trends in repository programmes
- •Priorities for future efforts
- •Waste characterisation
- •Operational safety
- •Emplacement technologies
- •Knowledge management
- •Alternative designs and optimisation processes
- •Materials technology
- •Novel construction/immobilisation materials: the example of low pH cement
- •Future SA code development
- •Implications for environmental protection: disposal of other wastes
- •Conclusions
- •References
- •Index
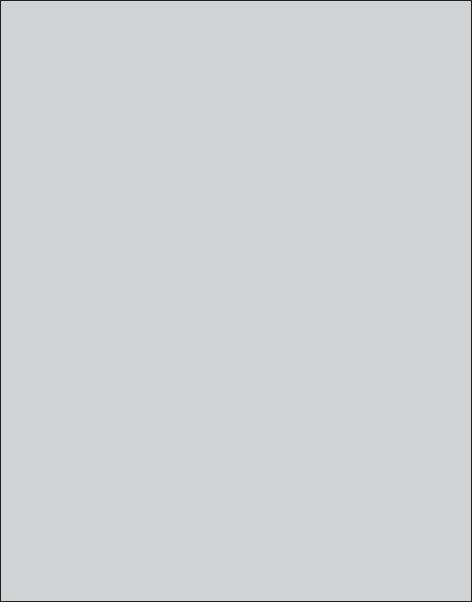
Research and development infrastructure |
207 |
Box 8.1. Examples of URL experiments on two different EBS concepts.
The European Commission-sponsored Prototype Repository Project (PRP) is based on a repository concept similar to SKB’s KBS-3 concept for SF disposal and involved full-scale experiments in the
¨ ¨
Aspo URL in Sweden, with drilling of a deposition hole, installation of a bentonite buffer, deposition of a waste canister and backfilling of tunnels. Electric heaters were used within the waste canister to simulate the heating effect of spent fuel. The project highlighted practical difficulties associated with repository operations and it was noted that these may act as constraints on disposal system design. It was also noted that the effects of these constraints are often not represented in SA models and, thus, the design process must be supported by information from engineering and other studies, as well as SA. Examples of the practical difficulties encountered include:
Accurate drilling of the deposition hole avoiding surface roughness, variations in diameter and curvature.
Having sufficiently powerful pumps to extract water flowing into the 8.5 m deep deposition holes.
Preventing water inflow from affecting the bentonite prior to canister deposition.
Handling and placement of many layers of heavy ( 2 tonne) bentonite blocks to millimetre accuracy.
Accurate positioning of the canister.
Achieving sufficient compaction of the backfill.
The PRP has shown that, despite the difficulties, accurate deposition hole drilling is possible, that the use of larger bentonite blocks would simplify the buffer emplacement process, that engineering solutions exist for limiting the inflow of water to the deposition hole (use of plastic liners) and that sufficient compaction of the backfill can be achieved for mixtures containing up to 30 per cent bentonite, but that the transport of the backfill mixture to its site of emplacement is an inefficient process.
Another example is a large-scale test for gas migration in the EBS and the adjacent geosphere (GMT) for a L/ILW silo-type repository which is being carried out at Nagra’s Grimsel Test Site (Shimura et al., 2006). As mentioned in the previous section, the gas permeability is an important issue in L/ILW repository system design and the GMT experiment considers the events related to gas generation and migration, which could influence the repository performance. Excess gas pressure could cause high stresses on the concrete silo and other EBS components leading to mechanical failure and pushing contaminated water out from the waste packages and the disposal cavern into the geosphere. Escaping gas could transport volatile radionuclides through the EBS and the surrounding host rock.
It is aimed through the experiment to assess the function of the whole system (the EBS and adjacent geosphere) in terms of gas migration, evaluate models of gas migration through the engineered barriers and into the geosphere, provide data for further improvement of the EBS design and emplacement procedures and demonstrate the construction and EBS emplacement for the repository. The experimental procedure consists of excavating a cavern, emplacing the engineered barrier systems (concrete silo, sand/bentonite buffer) and injecting gas in the centre of the concrete silo (Figs 8.5 and 8.6). Laboratory studies, detailed site characterisation activities (hydrogeological, rock mechanical) and modelling studies complement the main experiment. The GMT has demonstrated the feasibility of constructing and instrumenting a silo-type disposal system. The project is still ongoing, but initial results indicate that the neither the concrete nor the buffer suffered catastrophic loss of their containment ability following gas transport across them to the host rock (see Senger et al., 2006, for details).
have investigated the likely effects of gas produced (by metal corrosion and degradation of organics) in a concrete silo for L/ILW (see Figs 8.5 and 8.6 and Box 8.1).
8.3.3. Biosphere
National regulations for the disposal of radioactive waste usually require the proponent to assess the radiological impact of a proposed repository on the exposed population.
208 |
A. Hooper |
Invariably, the radiological impact is defined in terms of risk or dose or, rarely, both (e.g., Nagra, 2002a). Such calculations require information about the habits and lifestyles of the exposed humans; what they eat for example. But, because radionuclides from a repository are unlikely to reach the biosphere for many thousands of years, information about human habits and lifestyle at that time can only be imagined and is accepted by most implementers and regulators as being very difficult to predict. In addition, climate and landscape changes could profoundly affect the environment in which these humans will live and this, too, will have an impact on the dose calculation.
Biosphere R&D therefore aims to provide information to support the construction of assessment biospheres. These are models used to calculate dose and risk for different combinations of biosphere (e.g., climate and landscape) conditions. To achieve this, information is required in three basic areas, discussed in the next three sub-sections.
8.3.3.1. Radionuclide concentration and dispersion in the biosphere
When radionuclides appear in the biosphere, they can become concentrated in environmental media, such as soil and plants, which are subsequently used by humans. This gives rise to a range of exposure pathways, of which ingestion is usually the most important. Calculation of dose starts with the construction of exposure scenarios (e.g., IAEA, 2003); these require information on parameters such as the sorption of radionuclides on soil, uptake of radionuclides by plants, and radionuclide transfer factors for, say, cattle fodder to milk. For radionuclides released in groundwater, such data have been measured and are available for key radionuclides and exposure pathways.
8.3.3.2. Climate change
Interest in climate change forced by anthropogenic carbon dioxide has generated a large body of literature on the subject. Radwaste disposal programmes are mostly interested in climate change over longer time periods than are usually found in the wider literature but, nonetheless, disposal programmes have been fortunate in that they have been able to draw on this information and expertise to build an understanding of how the future climate might develop. Recent R&D has constructed a catalogue of possible future climate states – and corresponding environments – for use in post-closure safety assessment (e.g., Pro¨hl and Texier, 2004).
8.3.3.3. Landscape change
While recognising that landscape and climate are coupled, it is still useful to outline the ways in which landscape can affect dose calculations so that the main areas of R&D can be highlighted. One of the most significant landscape changes – as far as radwaste disposal is concerned – occurs when a repository radionuclide discharge area that is currently covered by water becomes uncovered. Examples are uplift caused by glacial rebound (SKB, 1999) or tectonics (Nagra, 2002a), sea-level fall under ice age conditions (Nirex, 1997a; JNC, 2000) and sedimentation of lakes. Other important factors are the effects of cold climate periods, specifically, glacial erosion (Nagra, 1994; Nirex, 1995) and deep injection of water beneath ice-sheets (Boulton et al., 2001).
The role and treatment of the biosphere in SAs has been discussed extensively in international fora. The consensus from these discussions is that it is reasonable to assess biosphere performance in a schematic manner, compared to the assessment of the engineered barriers and geosphere, e.g., as proposed by the NEA Biosphere Working