
Photochemistry_of_Organic
.pdf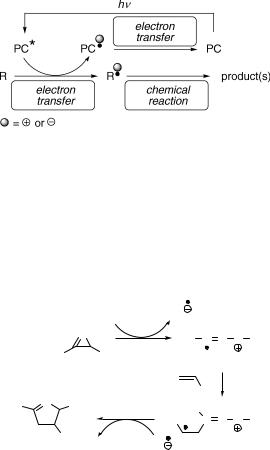
426 |
Chemistry of Excited Molecules |
Organic Photocatalysts: Electron Transfer
The term photosensitization can also be employed for a process initiated by electron transfer (Section 6.4.4), in which the photosensitizer serves as an electron donor or acceptor.1469 Such a compound will be called a photocatalyst (PC) if it is regenerated in an associated (auxiliary) electron transfer reaction elsewhere in the reaction cycle (Scheme 6.271). Lower than stoichiometric amounts of PC can logically be used in this case.
Scheme 6.271
Section 6.4.4 already presented several examples of photocatalysts such as metalloporphyrins and other organic metal complexes. In another example, a singlet excited photocatalyst, 1,4-dicyanonaphthalene (DCN), initiates the photochemical electron transfer-induced ring opening of an azirine 568 (Scheme 6.272).1473 Subsequent addition of an intermediate 569 to acrylonitrile leads to 570 in two steps, while DCN is regenerated.883
|
|
1DCN* |
DCN |
|
|
|
|
N |
|
H |
|
|
|
|
Ph C N C Ph |
||
|
Ph |
568 Ph |
|
569 |
|
|
N |
|
|
CN |
|
Ph |
Ph |
Ph |
H |
||
|
|||||
|
|
|
|||
|
|
|
NC |
C N C Ph |
|
|
CN |
|
|
||
|
570 |
DCN |
DCN |
|
Scheme 6.272
Scheme 6.273 illustrates the photocatalytic addition of a tertiary amine (571) to the furanone 572,1474 which is initiated by electron transfer from the amine to a photoexcited benzophenone to form a radical ion pair.1475 The ketyl radical anion abstracts a proton from an amine radical cation and subsequently donates a hydrogen atom to the previously coupled radical intermediate 573 to regenerate the benzophenone molecule and to give the final addition product 574 (<94% chemical yield).
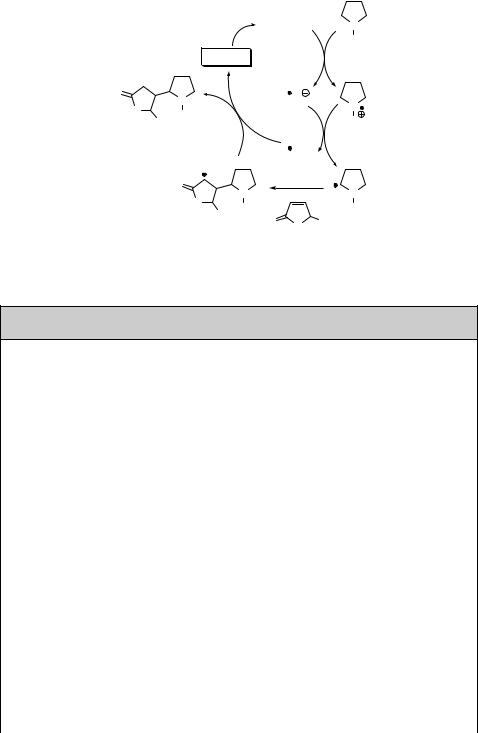
Photosensitizers, Photoinitiators and Photocatalysts |
427 |
|||||
|
|
1. hν |
3Ph2C=O* |
N |
|
|
|
|
2. ISC |
|
|||
|
|
|
|
|
R |
|
|
|
Ph2C=O |
|
|
571 |
|
|
|
|
|
|
|
|
O |
N |
|
Ph2C-O |
|
|
|
O |
|
N |
|
|||
R |
|
|
|
R |
|
|
R' |
|
|
|
|
||
|
|
|
|
|
||
574 |
|
|
|
|
|
|
R = alkyl |
|
|
Ph2C-OH |
|
|
|
R' = alkoxy |
|
|
|
|
||
|
|
|
|
|
|
|
|
O |
N |
|
|
N |
|
|
O |
|
|
|
||
|
R |
|
|
R |
|
|
|
|
|
|
|
||
|
|
R' |
O |
O |
R' |
|
|
|
573 |
|
|||
|
|
|
|
|
||
|
|
|
572 |
|
|
|
|
|
|
|
|
|
Scheme 6.273
Special Topic 6.25: Photosynthesis in bacteria and plants
Photosynthesis is the conversion of light energy into chemical potential in living organisms. It involves direct excitation, rapid and efficient energy and electron transfer
processes and subsequent dark chemical reactions.
The simplest photosynthetic system is that found in purple bacteria.1476,1477 The initial event is absorption of a photon by the light-harvesting antenna system LH2, consisting of a ring of 18 (or nine) bacteriochlorophyll (BC) molecules (see the
absorption spectrum in Figure 6.9) and associated with the organic pigments carotenoids within the walls of two helical protein molecules (Figure 6.18).1478,1479 BC molecules
are largely exciton-coupled (see also Special Topic 6.29), i.e., the exciton state is delocalized over several chromophores. The excitation energy is then rapidly transferred to a similar but larger antenna complex (LH1) consisting of 32 BC molecules, surrounding the reaction centre (RC; see below). The excitation energy migration among the rings occurs on a time scale of picoseconds. The singlet–singlet resonance energy transfer (section 2.2.2) takes place possibly through quantum coherence to locate the most efficient transfer path.1480 There are several LH2 complexes surrounding LH1. The energy transfer from LH1 to RC is then slower by an order of magnitude.
The primary photosynthetic process is carried out by a pigment–protein complex –
the reaction centre (RC) – embedded in a lipid bilayer membrane (Figure 6.19) and surrounded by light-harvesting complexes.1477,1481,1482 Thus energy is transferred from
LH1 to a bacteriochlorophyll special pair (P) and then through a bacteriochlorophyll molecule (BC; monomer) to bacteriopheophytin (BP; a chlorophyll molecule lacking the central Mg2 þ ion), followed by electron transfer to a quinone QA in hundreds of ps. The neutral P is then restored by electron transfer from the nearest intermembrane space protein cytochrome c (Cyt c) in hundreds of ns. The rate constants of the
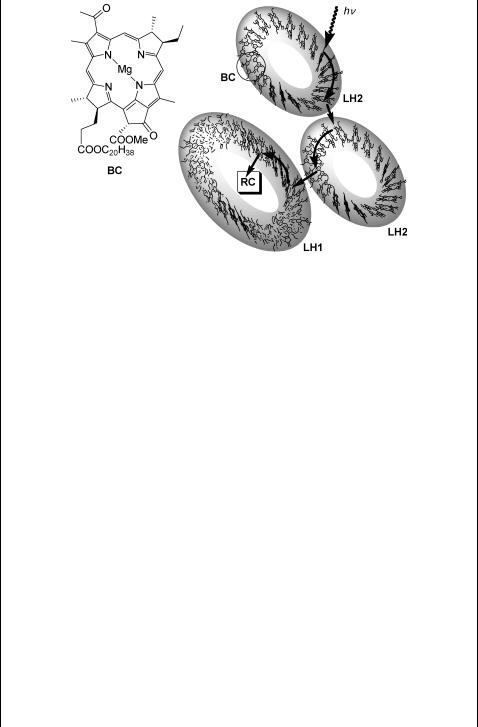
428 |
Chemistry of Excited Molecules |
Figure 6.18 Energy transfer in light-harvesting complexes
electron transfer steps are shown in Figure 6.20; these charge separation processes over large distances (across the membrane) are highly efficient and essentially nonreversible because charge recombination rates lie in the Marcus inverted region (Section 5.2).1477 The whole charge separation process converts light energy in about 10 ms and with a quantum yield of near unity, but only a fraction of the excited-state energy is conserved in the final charge-separated state. The final electron acceptor QA is reduced in a two-electron–two-proton process to a hydroquinone (QAH2). This compound diffuses to cytochrome bc, also called a proton pump, where it oxidizes back to QA (Figure 6.21). The energy released is used to transfer a proton through the membrane to ATP synthase, the enzyme that synthesizes ATP from ADP and inorganic phosphate (Pi). ATP is then used as a high-energy compound to drive various endergonic biochemical reactions.
The biophysics of anaerobic photosynthesis in purple bacteria described above is now well understood. Photosynthesis in green plants and cyanobacteria is similar but more complex and differs mainly by the presence of two associated lightharvesting centres and oxygen production. In the case of plants, photosynthesis occurs in organelles called chloroplasts utilizing two protein photosystems. Photosystem II, absorbing light at lmax ¼ 680 nm, provides an oxidation potential that is sufficient to remove an electron from water in a manganese-containing metalloenzyme (called an oxygen-evolving complex) to produce oxygen.1483 The electron is transferred via several carriers to an electron-deficient photosystem I
where |
chlorophyll a serves as the reaction centre. Upon absorption of light at |
lmax |
700 nm, an electron is transported to the iron–sulfur protein ferredoxin, |
where it reduces coenzyme NADP þ to NADPH or provides the energy for the phosphorylation of ADP to ATP.
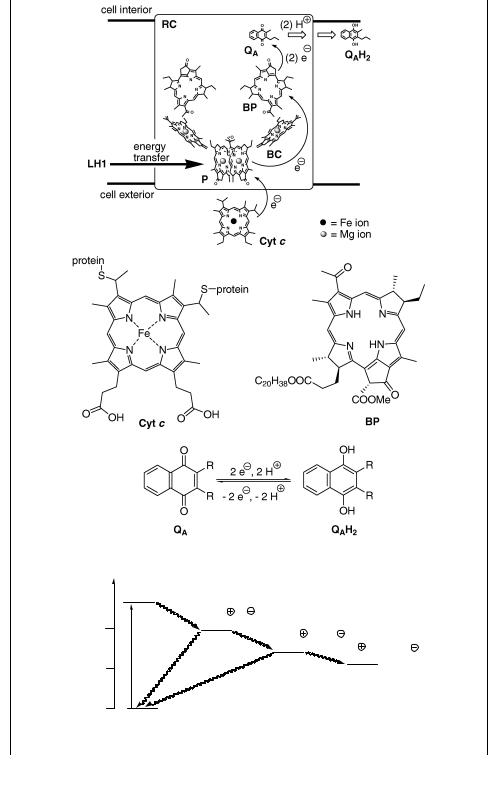
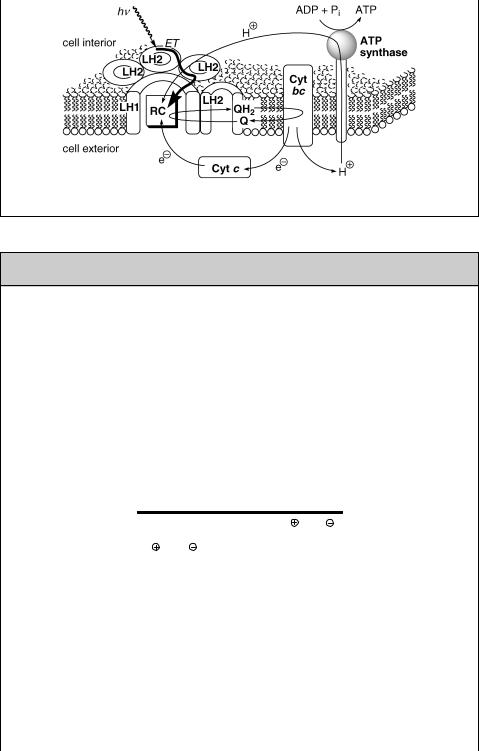
430 |
Chemistry of Excited Molecules |
Figure 6.21 Photosynthetic membrane
Special Topic 6.26: Artificial photosynthesis
There is increasing demand for inexpensive, clean energy sources. Although this demand could be met with fossil (limited) fuels, CO2 emissions in the atmosphere have already risen and may be an important contributor to global warming. Novel chemical systems capable of solar energy conversion, that is, artificial nanoscale devices and rudimentary semibiological hybrids (artificial photosynthetic systems) that can perform many of the
functions of the natural photosynthetic process (Special Topic 6.25), are being developed.5,7,1248,1477,1484–1487 The conversion of solar energy into the form of fuels is
challenging and attractive at the same time. Molecular hydrogen, as the most promising and environmentally friendly fuel accessible by a photoinduced water splitting process, is expected to be widely used in fuel cell-powered vehicles in the future. This process, by which water can be converted to hydrogen and oxygen using light:
hν
2H2O 2H2 + O2 cat.
2H2O O2 + 4H + 4e
4H + 4e 2H2
can be photocatalysed by many inorganic semiconductors.1484,1488 As yet, no catalytic process is capable of making the conversion with a quantum yield that is sufficient for commercially profitable applications (i.e. >10%) using visible light.1489 Hydrogen molecule can, however, be successfully produced also from various organic molecules and materials.1486 Alkanes or alcohols are, for example, dehydrogenated photochemically in the presence of various rhodium phosphine complexes, such as RhI(PR3)2(CO)Cl or RhI(PR3)3Cl.
Mimicking natural photosynthesis requires that all synthesized chemical building blocks linked covalently by self-assembly into a working molecular device, which basically consists of a light-harvesting antenna to capture light, reaction centres converting the excitation energy to chemical potential in the form of long-lived charge-
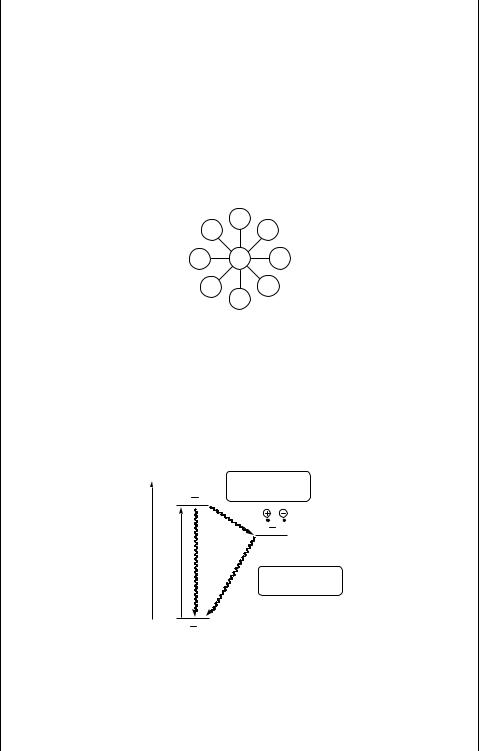
Photosensitizers, Photoinitiators and Photocatalysts |
431 |
separated systems, a catalyst converting the redox intermediates hereby formed to storable fuel and systems performing the physical separation of the products.
A light-harvesting antenna is a complex multi-chromophore system in which each component can absorb (visible) light and is able to transfer the excitation energy to the nearest neighbour chromophore before undergoing deactivation. Its efficiency depends on the distance and relative orientation of the chromophores. In recent years, the development of supramolecular chemistry has allowed the construction of very complex and efficient light-harvesting antennae, based for example on dendritic structures.1248,1477 Porphyrin, metalloporphyrins or phthalocyanine arrays are the first logical choice as the synthetic analogues of natural systems. Energy transfer occurs, for example, in 10–100 ps between Mg or Zn porphyrin (MP) and free-base porphyrin (P) assemblies. Figure 6.22 shows an example of MP and P assembly.
MP
MP MP
MP P MP
MP MP
MP
Figure 6.22 Light-harvesting antenna
The simplest reaction centre is a dyad, consisting of weakly electronically coupled electron donor (or acceptor) and electron acceptor (or donor) components to produce a charge-separated ion pair upon photoinitiated electron transfer (ket). Figure 6.23 implies that this system may undergo excited-state deactivation (kd) or charge recombination (kcr); the former process is overcome in nature with an array of efficient
short-range electron-transfer steps, thereby creating a charge separation that extends over a much greater distance (Special Topic 6.25).1477,1487
|
|
electron |
E |
1D* A |
transfer |
|
|
ket |
|
|
D A |
hν kd
kcr charge recombination
D A
Figure 6.23 Dyad1477
The scheme for a three-component system (triad),1485 which produces the charge separation over two bonds, is shown in Figure 6.24. After the initial excitation of the primary chromophore C (step 1), an electron is exergonically transferred to an acceptor (step 2), followed by a second thermal electron transfer step from a donor moiety to the oxidized chromophore C. Again, deactivation and charge recombination processes compete.
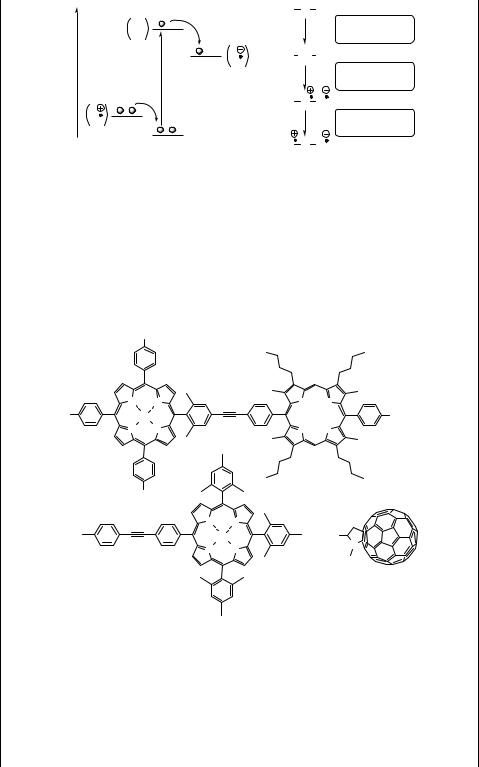
432 |
|
Chemistry of Excited Molecules |
|
||
|
|
|
|
D C A |
|
E |
C* |
2 |
|
hν |
excitation |
|
|
||||
|
|
|
A |
D C* A |
|
|
|
|
|
electron |
|
|
|
1 |
|
|
|
|
|
|
|
transfer |
|
|
3 |
|
|
D C A |
|
|
|
|
|
|
|
D |
|
|
|
|
charge |
|
|
C |
|
|
separation |
|
|
|
D C A |
|
|
|
|
|
|
|
Figure 6.24 Triad
Figure 6.25 shows an example of a six-component array (hexad) composed of four zinc porphyrin units (3 ZnP and 1 ZnPC) connected to a reaction centre (fullerene as an electron acceptor; F) through a free-base porphyrin (P) via acetylene bridges, which ensure weak electronic coupling.1490 Thus, excitation of any one of the peripheral zinc porphyrin moieties (ZnP ZnPC P F) is followed by singlet–singlet energy transfer to the central zinc porphyrin (ZnPC) in 50 ps to form ZnP ZnPC P F. The excitation is then passed on to the free base porphyrin in 240 ps (ZnP ZnPC P –F), which decays by electron transfer to the fullerene with a lifetime of 3 ps to produce a chargeseparated pair (ZnP–ZnPC–P . þ –F . ) having a lifetime of 1 ns.
R
N |
N |
NH |
N |
R |
Zn |
|
F |
N |
N |
N |
HN |
ZnPC |
|
P |
|
|
|
||
|
R |
|
|
|
N |
N |
|
R = |
|
Zn |
F = |
|
N |
N |
N |
|
|
ZnP
Figure 6.25 An example of a hexad
Water splitting to oxygen and hydrogen can be accomplished by photocatalysis.1484,1487 Some progress has been made in the field of artificial photosynthesis.
However, full integration of all components mimicking the natural process has not yet been achieved.1477 Figure 6.26 provides a schematic representation of water splitting using artificial photosynthesis. A triad D–C–A produces a charge-separated pair D. þ —C—A. upon excitation through a light-harvesting antenna (C0). A membrane physically separates the two reaction sites, the hydrogenand oxygen-evolving centres.

Photosensitizers, Photoinitiators and Photocatalysts |
433 |
Figure 6.26 Water splitting1477
Organic Photocatalysts: Radical Reaction
In a few less common procedures, the photocatalyst serves as a carrier of the hydrogen atom, subsequently forming a radical intermediate that must be regenerated via another hydrogen transfer step. For instance, a lower than stoichiometric amount of benzophenone is sufficient to trigger a photocatalysed addition of alkynes substituted with electronwithdrawing groups to cycloalkanes (575 is produced in less than 70% chemical yield; Scheme 6.274).1491 In addition, photosensitized isomerization via the Schenck mechanism (Scheme 6.15 in Section 6.1.1) may also represent a photocatalytic radical process.
1. hν
Ph2C=O 2. ISC 3Ph2C=O*
R |
|
Ph |
H |
H |
O |
Ph |
|
575 R
R
R = electron withdrawing group
Scheme 6.274
Organic Photoinitiators
In the event that the photoactive auxiliary molecule irreversibly degrades, that is, it is not regenerated and cannot be involved in another reaction cycle, the initial process should be more generically termed photoinitiation rather than photosensitization and the absorbing molecule a photoinitiator (Scheme 6.275; PI). If no chain propagation steps follow, equimolar amounts of both photoinitiator and substrate are required (see, for example, Scheme 6.200 in Section 6.4.4).

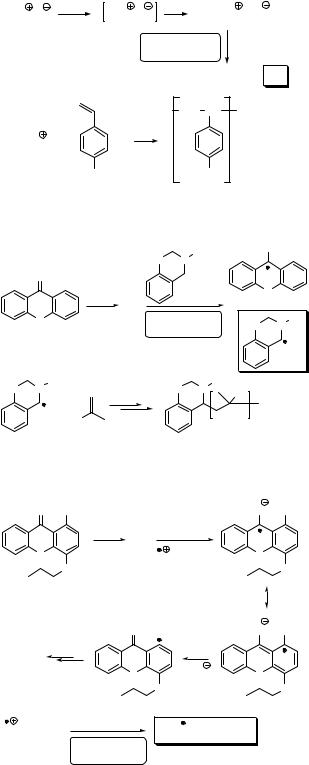