
Photochemistry_of_Organic
.pdf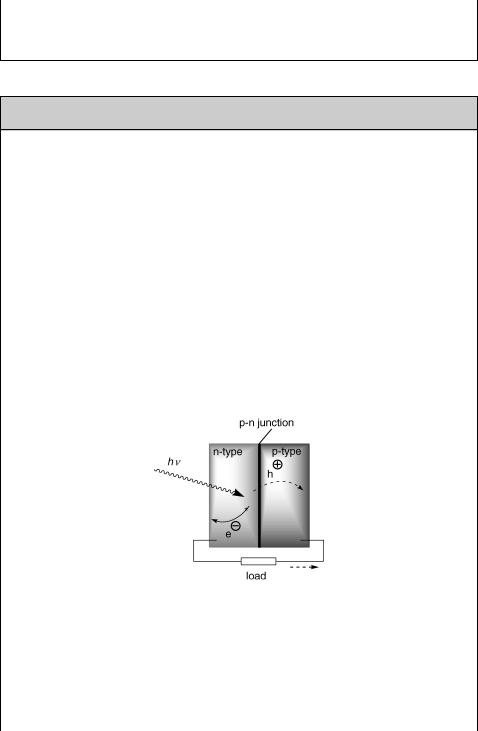
446 |
Chemistry of Excited Molecules |
corresponding to an agglomeration number of 300 CdS units. When generated in propan-2-ol, the mixture is colourless because the average size of the particles is less than 3 nm.
Special Topic 6.31: Photovoltaic and photoelectrochemical cells
Photovoltaic and photoelectrochemical cells convert solar energy to electric energy.5,1477,1533,1540,1541 In a photovoltaic cell,1542 light excites electrons across the
band gap of an inorganic semiconductor to form electron (e )–hole (h þ ) pairs, which are subsequently separated by a p–n junction, that is, by an interface between doped n- type and p-type semiconductor layers (Figure 6.30). This drives the electrons in one direction and holes in the other, generating a potential difference at the external electrodes (with a load). Today, the best commercially available solar cells based on
crystalline or amorphous silicon have 15–20% efficiency and lifetimes of about 30 years.1543,1544 The cell production is a trade-off between price (mostly dictated by
the purity of the material used) and overall operational efficiency. Even for cells that operate close to the theoretical efficiency limit (the maximum power divided by the input light irradiance and the surface area of the solar cell), cost-effective cell manufacturing and electric power storage are still the greatest challenge for both research and technological development. Recent scientific efforts have been focused on utilizing nanocrystalline and conducting polymer devices, which might be relatively cheap to fabricate.
Figure 6.30 Photovoltaic cell
Photoelectrochemical cells that benefit from the sensitization of wide-gap semiconductors (Scheme 6.293) by organic dyes are referred to as Gra¨tzel cells.1533,1540,1545,1546 Figure 6.31 shows a cell, consisting of (1) a photoanode, a mesoporous semiconductor [typically TiO2 (anatase) film] deposited on a conductive glass (current collector) by screen printing, with a photosensitizer adsorbed on a semiconductor surface, (2) a metallic cathode and (3) an electrolyte solution containing
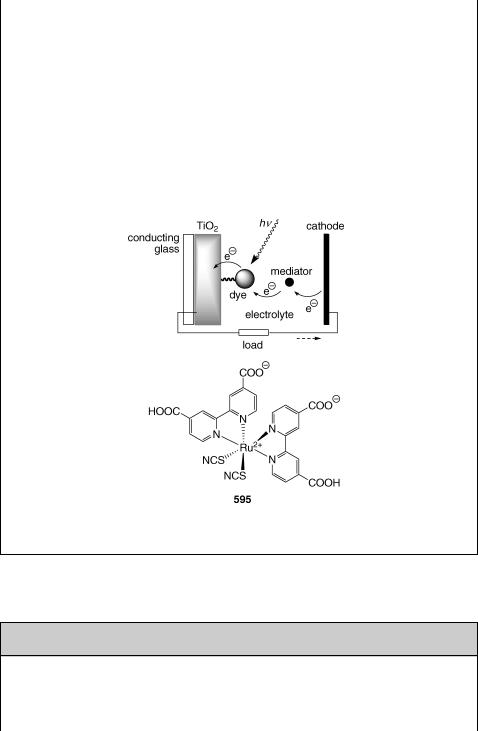
Photosensitizers, Photoinitiators and Photocatalysts |
447 |
a redox mediator. In such an arrangement, light is absorbed by a dye, which upon excitation injects an electron to the conduction band of a semiconductor and is subsequently reduced by a mediator (usually the I3 /I couple). The oxidized mediator travels to the cathode and is discharged to promote electric current when the external circuit has a load. The semiconductor structure is typically 10 mm thick and with a high porosity, which provides a large surface area for efficient chemisorption of a dye. Many different photosensitizers have been studied. An exceptional photovoltaic performance has so far been accomplished with polypyridyl complexes of ruthenium and osmium (e.g. 595).1545 They exhibit metal-to-ligand charge transfer and often bear carboxylic groups attached to the semiconductor surface. Overall solar conversion efficiencies by photoelectrochemical cells of over 10% have already been reached in the laboratory.1545
Figure 6.31 Photoelectrochemical cell and a sensitizer
Special Topic 6.32: Photography, xerography and photoconductivity
The photographic process, another photoimaging technique (see also Special Topic 6.27), is still the most widespread application of photochemistry.1547 IR, visible, UV and X-ray irradiation of photosensitive silver halide (Ag þ X ) crystals in a gelatine emulsion, coated on a film base, glass or paper substrate, causes reduction of interstitial
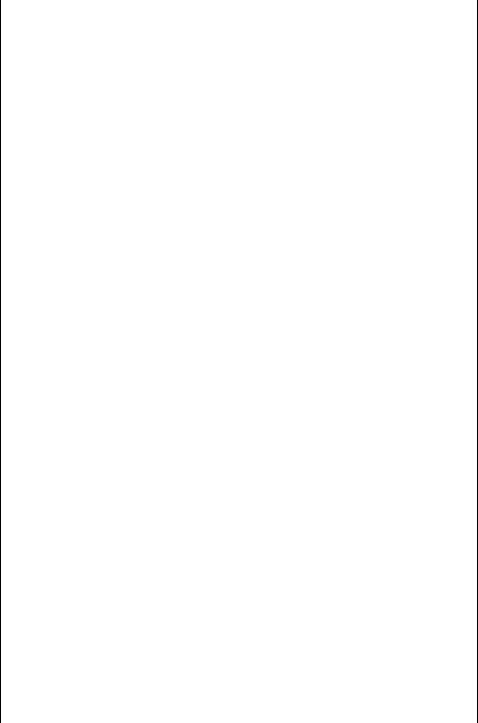
448 |
Chemistry of Excited Molecules |
silver ions to form atomic silver (Ag0). Silver halides are known to exhibit an increase in electrical conductivity due to the absorption of light (photoconductivity), in which electrons are excited from the valence band to the conduction band; this excited state (exciton) either decays or forms an electron–hole pair. The process then involves the migration of the electrons and the interstitial silver ions to preferential sites. Their combination then produces silver clusters, which further efficiently trap photoelectrons produced in the vicinity. As a result, specks of silver are gradually formed:
X þ hn ! X. þ e
Ag þ þ e ! Ag0
2 Ag þ þ e ! Ag2þ
Ag2þ þ e ! Ag2; etc:
The electron holes are also mobile; they diffuse towards the surface of the grain, where oxidation of halide ions can take place. A short exposure to light creates a low concentration of metallic silver atoms on silver halide grains, producing the so-called latent image, which becomes enhanced and stabilized in a developer solution containing an organic reducing agent, such as hydroquinone or N-methyl-4- aminophenol. Prolonged exposure causes darkening of the surface due to extensive metallic silver formation (print-out effect). Silver halides absorb considerably in the blue, violet and ultraviolet regions; however, addition of specific dyes (sensitizers; Section 6.8.1) to a photographic emulsion increases the sensitivity of AgX to other wavelengths. Sensitization specifically to the three primary colours (blue, green and red) is utilized in conventional colour photography, in which the dyes are located on the surfaces of three superimposed layers.
Xerography (from Greek, dry writing ) is a photocopying technique that combines electrostatic printing with photography.1548 In this technique, the surface of a cylindrical drum, coated with a photoconductive material, such as selenium or an organic dye,1549 is electrostatically negatively charged at a high voltage (Figure 6.32). In the next step, an illuminated original document reflects the light on to a drum surface. The sites that are exposed to light (corresponding to bright areas in the document) become conductive and discharge to ground. Positively charged toner attaches to the non-illuminated areas that are still negatively charged and is subsequently transferred to a paper with a higher electrostatic negative charge than that of the drum. Laser printers utilize the same xerographic printing process; however, the primary image is obtained by sequential irradiation of the photosensitive charged surface with the laser beam.
The semiconductor selenium is an excellent photoconductor; however, its
photoresponsiveness decreases sharply at wavelengths over 550 nm, which has motivated research on organic photoconductive materials.1550,1551 Many different
organic compounds display photoconductive behaviour, such as phthalocyanines (596; M ¼ metal) or squaraines (597) (Figure 6.33); today more than 90% of xerographic photoreceptors are composed of organic photoconductors.

Photosensitizers, Photoinitiators and Photocatalysts |
449 |
Figure 6.32 Xerography
N |
R |
O |
R |
N M N |
|
||
N |
2+ |
N |
|
N |
R |
|
R |
|
O |
|
|
|
|
|
|
|
|
597 |
|
596
Figure 6.33 Photoconductive compounds
Semiconductor-photocatalysed transformations of functional groups often provide high
chemical yields, although the reaction quantum yields are usually not very high because a semiconductor surface may reflect radiation.170,1521,1525,1526 In general, an organic
functionality that contains a p-bond or a lone electron pair can be oxidized, which on the other hand considerably reduces the synthetic potential for applications involving substrates that have multiple reactive functional groups.
For example, alkenes such as propenylbenzene (598) and 1,1-diphenylethene (599) are selectively oxidized to the corresponding ketones in a very high chemical yield in
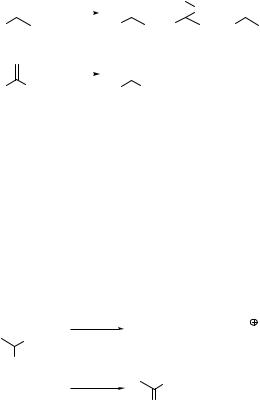
450 |
Chemistry of Excited Molecules |
air-saturated acetonitrile solutions (Scheme 6.294).1552 Only a small amount of other sideproducts, such as epoxides and aldehydes, are formed in the former case. Irradiation of the TiO2 band gap in acetonitrile promotes an electron into the conduction band (the band edge is 0.8 V vs SCE), forming a hole in the valence band ( þ 2.4 V vs SCE). As a result, molecules with standard potentials of oxidation less positive than 2.4 V may react, provided that the reduction couple has a redox potential less negative than 0.8 V, for
instance, because the standard potential of reduction of oxygen in acetonitrile is0.78 V.1525
|
|
|
|
hν, TiO2 |
|
|
O |
|
O |
CHO |
||
|
|
|
|
|
||||||||
|
|
|
|
|
+ |
|
+ |
|
||||
Ph |
|
|
|
O2, MeCN |
Ph |
|
Ph |
|
|
Ph |
||
|
598 |
|
|
|
|
84% |
14 % |
2% |
||||
|
|
|
|
hν, TiO2 |
|
|
O |
|
|
|
|
|
|
|
|
|
|
|
|
|
|
|
|
|
|
Ph |
|
Ph O2, MeCN |
Ph |
|
Ph |
|
|
|
|
|||
|
|
|
|
|
|
|||||||
|
599 |
|
|
|
sole product |
|
|
|
|
Scheme 6.294
Photocatalytic oxidation of lactic acid (600) on platinized TiO2 or CdS is strongly regioselective (Scheme 6.295).1553 Irradiation in the presence of Pt/TiO2 at 360–420 nm leads to the cleavage oxidation products acetaldehyde and carbon dioxide, whereas pyruvic acid is exclusively obtained by irradiation of the Pt CdS mixture at 440–520 nm. Since the standard potential of oxidation of acetic acid is known to be more positive than the valence band edge of CdS (CdS has a less positive valence band edge than does TiO2), both catalysts readily oxidize aliphatic alcohols and the CdS photocatalyst is apparently capable of specific oxidation.
hν, Pt/TiO2
COOH H2O
CH3CHO + CO2 + H
OH
600 |
hν, Pt/CdS |
COOH |
|
H2O O
Scheme 6.295
The mechanism of photoinduced oxidation of aromatic compounds mediated by TiO2 in aqueous media is demonstrated by the reaction of 4-chlorophenol (601). Its degradation is
principally based on oxidation by photocatalytically produced hydroxyl radicals, most likely adsorbed on the surface of a semiconductor catalyst.1554,1555 The initial reaction
affords a 4-chlorodihydroxycyclodienyl radical 602, which releases the chlorine atom to form hydroquinone in a radical substitution reaction or loses the hydrogen atom via
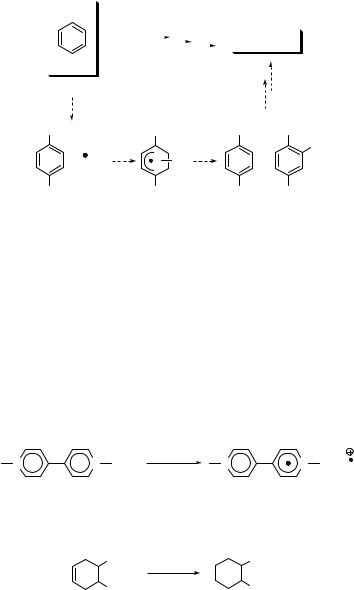
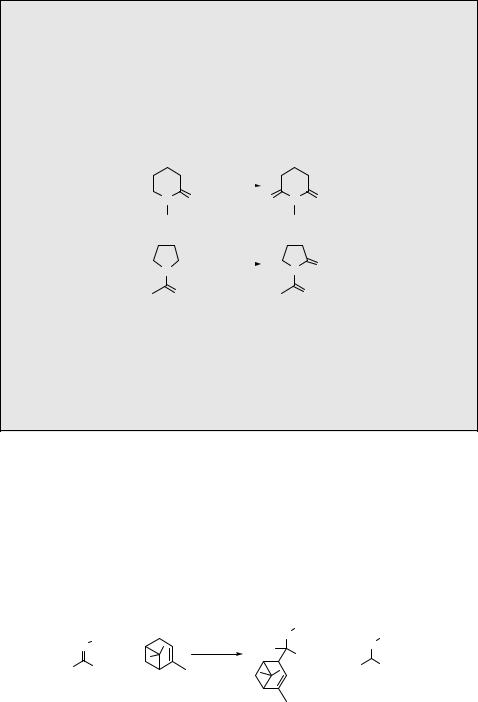
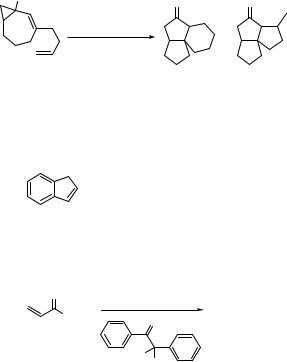
Photosensitizers, Photoinitiators and Photocatalysts |
453 |
(b)
OSiMe3 |
O |
O |
|
hν |
+ |
|
phenanthrene, |
|
|
|
|
|
dicyanoanthracene |
|
[ref. 1558]
3.Predict the major photoproduct(s):
(a)
hν
+ CH3OH phenanthrene
p-dicyanobenzene
[ref. 1231]
(b)
O |
hν |
NH2 O
MeO OMe (water-soluble host/guest complex)
[ref. 1559]

7
Retrosynthetic Photochemistry
Although photochemistry is widely used in many chemical and biological applications (Chapter 6), photochemical synthesis unfortunately remains far from being adopted by the general chemical community, despite the fact that a great number of procedures have been developed that afford products in high chemical yields and structures that can be made only with difficulty by conventional methods. Once a photochemical reaction is selected for an organic synthesis, it typically represents only one step in a multistep construction process of other dark reactions. Such a light-induced transformation, then, represents a challenge for the synthetic chemist, who should be relatively knowledgeable about certain theoretical and experimental aspects.
Photochemistry is a very promising tool in many applications indeed:
. Photochemical reactions can be green to the extent that no other reagents but light are used.
. Unlike thermal processes, photochemical reactions usually do not require heating; quite the opposite, they can be performed at very low temperatures and even in the solid phase.
. Photochemistry offers many unique means of reaction control, such as selective irradiation wavelength, multi-photon absorption, indirect (sensitizer-mediated) excitation, sensitizer-mediated electron transfer initiation, temperature and phase variations, timing and spatial control.
. Reactions proceeding after the excitation can be very fast and efficient, because reactive (unstable) intermediates, such as radicals, biradicals, radical ions, carbenes and strained rings, are often involved.
This short chapter may serve as a problem-solving list of target structures, which can be synthesized by photochemical means. The information is sorted according to structural types of products and is limited to the reactions described in Chapter 6. A double arrow ()) indicates the retrosynthetic direction,1560 starting from the product and showing the most important photochemical step in the course of the reaction. This does not necessarily mean that we can choose the corresponding procedure under all circumstances. Potential
Photochemistry of Organic Compounds: From Concepts to Practice Petr Klán and Jakob Wirz © 2009 P. Klán and J. Wirz. ISBN: 978-1-405-19088-6
456 |
Retrosynthetic Photochemistry |
obstacles and limitations must be considered carefully, because no known (photo)chemical procedure is truly universally applicable.
The following list of potential obstacles may prompt the reader to go back over the preceding chapters, which provide sufficient details to handle most of the photochemical procedures successfully. The obstacles are listed here not to discourage, but, on the contrary, to accentuate that careful preparation for the experiment and consideration of possible difficulties will help in achieving fast and efficient photoprocesses, which selectively produce the desired compounds in very high chemical yields. Thus we should be aware that:
. The excited chromophore may undergo other competing chemical processes, which are dependent on the substrate structure and also on the presence of other compounds in the reaction environment.
. The excited chromophore may undergo physical deactivation, which lowers the quantum yield of the reaction.
. Other chromophores present in the substrate or the reaction system may impede the reaction and/or undergo unwanted reactions themselves.
. Solvent may interfere with the reaction as a reagent or as an optical filter.
. The presence of acids, bases, electrophilic and nucleophilic species or good electron donors and acceptors may strongly affect many photoreactions.
. The reaction intermediates may attack the reacting molecules.
. Oxygen and many other species can quench the excited state.
. Singlet oxygen produced in situ by sensitization can react with most molecules.
. The products or long-lived intermediates may absorb light and thereby become optical filters.
. The target product may undergo subsequent unwanted thermal or photochemical transformations.
Tables 7.1–7.13 are self-explanatory and the reactions represent only simple examples. New target product skeletons or bonds are shown in bold. Detailed mechanistic descriptions and reaction limitations are discussed in Chapter 6.