
биохимия атеросклероза
.pdf98 Kathryn E. Naus and Cheryl L. Wellington
particularly important during lesion progression when there is a progressive increase in the FC:CE ratio in lipid-laden macrophages. Additionally, because this pathway requires a functional Neimann–Pick type C gene [99], it may also contribute to impaired ABCA1-dependent lipid efflux and reduced HDL levels in NPC disease [100]. Recently, Albrecht et al. [101] showed that ABCA1 protein levels are dramatically reduced in advanced atherosclerotic lesions despite high levels of ABCA1 mRNA, suggesting that the microenvironment surrounding the atherosclerotic plaque triggers signaling pathways that regulate ABCA1 abundance. This study demonstrates that posttranscriptional regulation of ABCA1 occurs in vivo, and that a deeper understanding of these pathways may lead to an improved ability to retard foam cell formation and reduce the development of atherosclerotic lesions.
Lack of ABCA1 in humans and mice results in a nearly complete absence of plasma HDL, demonstrating that apoAI requires functional ABCA1 activity to acquire lipids and contribute to HDL biogenesis [57–59, 102–104]. In addition to apoAI, however, ABCA1 can also deliver lipids to other exchangeable lipoproteins including apoAIV, apoE, and apoC [79, 105], suggesting that ABCA1 can efflux lipids to proteins that contain amphipathic α-helices. The ability of synthetic amphipathic helices to accept lipids from ABCA1 further supports this hypothesis [106–108]. Exactly how ABCA1 transfers lipids to apoAI remains a subject of considerable investigation. One model proposes that contact between ABCA1 and helices 9/10 of apoAI induces the net transfer of phospholipids and cholesterol [109–112]. Recently, the interaction with apoAI has been suggested to occur with dimeric and tetrameric, but not with monomeric ABCA1 [113], and the formation of higher order ABCA1 structures could explain previous observations of dominant negative effects of truncation mutations on ABCA1 protein levels, which implies a physical interaction among ABCA1 molecules [114]. A second model proposes that ABCA1 translocates lipids to the exofacial leaflet of the plasma membrane independent of apoAI, generating a membrane microenvironment required for subsequent apoAI docking and lipid efflux [115–117]. Whether transfer of phospholipids and cholesterol onto apoAI occur simultaneously [118–121] or sequentially [122–125] remains to be fully elucidated.
ABCA1 is broadly expressed in many tissues, and liver and macrophages are key cell types involved in sterol homeostasis [126, 127]. Liver ABCA1 is the major regulator of plasma HDL levels, as selective inactivation of hepatic ABCA1 reduces plasma HDL by approximately 80% [128], whereas specific overexpression of ABCA1 in the liver increases plasma HDL levels [129, 130]. Within the liver, ABCA1 is most abundant in parenchymal and Kupffer cells compared to endothelial cells [131], and hepatocyte ABCA1 has been shown to play a critical role in the lipidation of newly synthesized apoAI [132]. In contrast to the liver, macrophage ABCA1 plays a minimal role in determination of plasma HDL-C levels but critically regulates atherosclerotic lesion development, as described below.
Chapter 6. ABC Transporters and Apolipoprotein E |
99 |
ABCG1
ABCG1 is the founding member of the ABCG subclass of ABC transporters [54]. ABCG1 is a half-size transporter with one ATPase-binding cassette [54–56, 133–136], and therefore requires either homodimerization or heterodimerization to provide functional transporter activity. Recent reports suggest that ABCG1 can function as a homodimer, and there are conflicting reports whether ABCG1 may also form functional heterodimers with ABCG4. In one study, HEK 293 cells transiently transfected with either ABCG1 or ABCG4 demonstrated that separately, each promoted cholesterol efflux to HDL but not to lipid-free apoAI [137]. However, coexpression of ABCG1 and ABCG4 did not potentiate lipid efflux compared to cells individually expressing either transporter, suggesting that ABCG1–ABCG4 heterodimers, if formed, may not display an enhanced ability to efflux lipid. In contrast, another report recently suggested that ATPase activity increased in insect cells expressing either ABCG1 or ABCG4, and that ATPase activity was augmented by coexpression of both genes [138]. This study concluded that ABCG1 and ABCG4 may function as homodimers or heterodimers, at least with respect to ATPase activity [138].
The human ABCG1 gene contains 23 known exons and spans approximately 98 kb [134–136, 139]. The murine ABCG1 gene contains regions of similarity to the human gene from exons 5–23. Thus far, human ABCG1 transcripts have been studied primarily in macrophages, where at least 11 different mRNAs have been described that result from complex patterns of alternative transcription initiation, alternative splicing, and translation initiation [134–136, 139]. At least seven different ABCG1 protein isoforms with amino termini comprised of various combinations of exons 1–10 spliced to exons 11–23 have been predicted in macrophages alone [134–136, 139]. The presence of multiple alternative amino termini for ABCG1 suggests that exons 1–10 may encode regulatory domains and that exons 11–23, which encode the nucleotide binding and transmembrane domains, may constitute the catalytic portion of ABCG1. Multiple ABCG1 transcripts are responsive to LXR–RXR stimulation [135, 136]. Two LXR responsive elements conserved between human and murine ABCG1 are located within intron 7 and have been shown to regulate transcription of ABCG1 [135]. In addition, an RXR response element located approximately 1.6 kb upstream of exon 1 has been postulated, but not proven, to regulate ABCG1 transcription [136]. Recently, the murine ABCG1 gene has also been shown to encode multiple transcripts [140].
ABCG1 mRNA is most abundant in brain and macrophage-rich tissues such as spleen and lung, as well as in placenta, intermediate in heart and muscle, and low in liver [140, 141]. Mice deficient in ABCG1 have recently been shown to accumulate lipids in tissues when challenged with a high fat diet [142], suggesting that ABCG1 does participate in the regulation of body sterol homeostasis.
100 Kathryn E. Naus and Cheryl L. Wellington
ABCG1 facilitates cholesterol efflux to HDL2 and HDL3 but not to lipidfree apoA1 in transiently transfected HEK 293 cells [137, 140]. Furthermore, antisense inhibition of ABCG1 expression reduces cholesterol efflux in a dose-dependent manner [137, 143]. These observations link ABCG1 to pathways relevant for atherosclerosis and RCT. Macrophage ABCG1 mRNA is highly induced by lipid loading as well as by LXR–RXR agonists [137, 144], and efflux of cholesterol from lipid-laden macrophages suppresses ABCG1 expression [143]. ABCG1 is also upregulated in macrophages isolated from individuals with Tangier disease [145], suggesting the possibility of a compensatory mechanism to enhance macrophage ABCG1 function in the absence of ABCA1. Recently, ABCG1 in human monocyte-derived macrophages was shown to be extraordinarily sensitive to cholesterol loading or exposure to LXR–RXR agonists, and was more closely associated with cholesterol efflux than ABCA1 levels [146]. These observations suggest that ABCG1 may also mediate lipid homeostasis and lipid efflux in macrophages, although this remains to be determined experimentally.
Macrophage-Specific Influences on Atherosclerosis
Because macrophages are derived from hematopoietic stem cells, bone marrow transplantation (BMT) is a popular approach for the study of macrophage-specific effects on cholesterol efflux and atherosclerosis (for review see Ref. [147]). After irradiation, donation of marrow to a recipient mouse leads to the repopulation of the recipient animal with macrophages of donor origin. A second method to obtain macrophage-specific expression in vivo is with the visna virus long terminal repeat (LTR). A construct that contains the visna virus LTR will allow directed expression of a gene of interest to macrophages [148].
The two most commonly used animal models of atherosclerosis are apoE−/− and LDLR−/− mice. Both of these models develop atherosclerotic lesions and have elevated plasma cholesterol; apoE−/− mice develop atherosclerosis spontaneously, whereas LDLR−/− mice require feeding with an atherosclerotic or Western-type diet (for reviews see Refs. [149, 150]). Feeding a Western-type diet to apoE−/− mice is often used to induce a more severe atherosclerotic pathology at a younger age. In addition, gender differences are apparent in both models, with LDLR−/− males having significantly greater lesion areas than females. Similar trends are observed in the apoE−/− model [151].
Macrophage apoE and Atherosclerosis
ApoE plays a crucial role in atherosclerosis [152, 153]. ApoE is synthesized predominantly by the liver, but is also made in several peripheral tissues including macrophages [152, 153]. Although a major function of apoE is to
Chapter 6. ABC Transporters and Apolipoprotein E |
101 |
facilitate the hepatic uptake of triglyceride-rich lipoprotein particles, macrophage-derived apoE has potent antiatherogenic activities that appear to act independently of its effects on plasma lipoproteins. In vitro lipid loading of macrophages stimulates synthesis and secretion of phospholipidbound apoE, which in turn facilitates cholesterol efflux [146, 154–159]. The local source of apoE not only supplies phospholipids as fatty acid donors for esterification of cholesterol, but transfer of apoE to HDL expands its surface and increases its capacity to accept cholesterol. Both human monocytederived macrophages [160, 161] and mouse peritoneal macrophages [159] are known to have the capacity to use endogenously secreted apoE as a cholesterol acceptor in the absence of other exogenous factors. Intriguingly, macrophage apoE-mediated efflux is dependent on culture volume; large culture volumes dilute the secreted apoE and result in low level efflux mediated only by apoE in the immediate juxtacellular space [159]. In contrast, at low culture volumes where apoE levels are saturating, cholesterol efflux is robust and is mediated by extracellular as well as juxtacellular apoE [159]. These observations suggest that endogenous apoE has both autocrine and paracrine effects that may become particularly important in the context of the vessel wall [159].
In addition to promoting local cholesterol efflux, macrophage-derived apoE has several other antiatherogenic properties including modulation of the inflammatory response within the vessel wall (reviewed in Refs. [162–164]). ApoE inhibits the expression of vascular cell adhesion molecule-1 on endothelial cells through its interaction with cell surface apoE receptor 2, which results in stimulation of nitric oxide synthase in endothelial cells [165, 166]. ApoE also inhibits lipid oxidation, T cell activation and proliferation, the migration and proliferation of smooth muscle cells, and platelet aggregation [167–174]. Thus, reductions in apoE levels or functional properties can affect lesion development through a number of mechanisms.
Many BMT and viral experiments done to determine the role of macrophage apoE in plasma lipid metabolism and atherosclerosis involved reconstitution of apoE−/− or wild-type (WT) mice with bone marrow from donors with varying levels of apoE (Table 6.1) (reviewed in Ref. [175]). Many groups have shown that reconstitution of WT bone marrow in hypercholesterolemic apoE−/− recipients resulted in a decrease in plasma total cholesterol, even when challenged with an atherogenic diet [176–182], demonstrating that apoE secreted by the WT macrophages can influence lipid metabolism. Furthermore, this decrease in cholesterol was dependent on the gene dosage, as apoE+/− donor marrow gave an intermediate level of plasma total cholesterol [178, 182]. Consistent with an antiatherogenic role of macrophage apoE, mean lesion area was reduced in mice that received WT compared to apoE−/− bone marrow [176, 177, 180–183]. Additionally, Zhu et al. [179] found that macrophage cholesterol efflux was increased in animals that received WT marrow. These antiatherogenic changes were obtained despite the fact that plasma apoE levels were in all cases <10% of the WT murine levels, indicating the
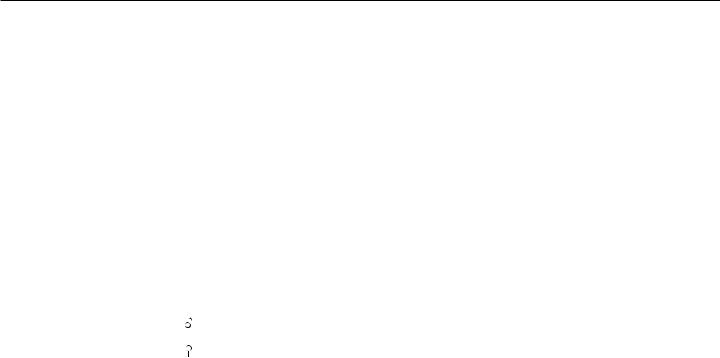
TABLE 6.1. Effect of macrophage apoE on atherosclerosis.
|
|
Plasma apoE |
|
mΦ Cholesterol |
|
|
Group |
Donor |
Recipient (% of WT)* |
Diet |
efflux |
Total cholesterol |
Mean lesion area |
|
|
|
|
|
|
|
Bellosta et al. [176] |
hTgE+/0 |
|
hTgE+/+ |
Boisvert et al. [177] |
apoE+/+ |
|
apoE−/− |
Linton et al. [178] |
apoE+/+ |
|
apoE+/− |
|
apoE−/− |
Fazio et al. [183] |
apoE+/+ |
|
apoE−/− |
Van Eck et al. [182] |
apoE+/+ |
|
apoE+/− |
|
apoE−/− |
Zhu et al. [179] |
apoE+/+ |
|
hTgE+/0 |
|
apoE−/− |
apoE−/−
apoE−/−
apoE−/−
WT ()
apoE−/−
apoE−/−
(?)
hTgE+/0 (3–5%) |
C |
NM |
hTgE+/+ (8%) |
|
|
NM |
C |
NM |
|
A/W |
|
NM |
C |
NM |
|
A/W |
|
hTgE+/0 (↓ 31%) |
hTgE+/0 (↓ 3×) |
hTgE+/+ (↓ 60%) |
|
apoE+/+ (↓50%) |
|
apoE−/− (no change) |
|
apoE−/− ↑ 4× above |
apoE+/+ (↓ not |
apoE+/+ |
quantified) |
apoE+/+ (↓ 74%) |
|
apoE+/− (↓ 51%) |
|
apoE−/− (no change) |
|
apoE−/− ↑ 3× above |
apoE+/+ (↓ 52×) |
apoE+/+ |
|
No change |
C |
NM |
No change |
apoE+/+ (↓ 10×) |
|
A/W |
|
No change |
|
apoE+/+ (3.5%) |
C |
NM |
apoE+/+ (↓ 91%) |
|
apoE+/− (1.9%) |
|
|
apoE+/− (↓ 82%) |
|
|
|
|
apoE−/− (↓ 13%) |
|
|
A/W |
|
apoE−/− ↑ 1.4× above |
apoE+/+ (↓ not |
|
|
|
apoE+/+ |
quantified) |
hTgE+/0 (7%) |
C |
apoE+/+ (↑ 48–74%) |
apoE+/+ (↓ 82%) |
NM |
|
|
hTgE+/0 (↑ 37–49%) |
hTgE+/0 (no change) |
|
Boisvert et al. [184] |
apoE+/+ |
WT ( |
) |
No change |
C |
NM |
No change |
apoE+/+ (↑ 2×) |
|
apoE−/− |
|
|
|
A/W |
apoE+/+ (↑ 42%) |
No change |
|
Van Eck et al. [185] |
apoE+/+ |
WT ( |
) |
No change |
C |
No change |
|
|
|
apoE−/− |
|
|
|
A/W |
|
apoE−/− FC ↑ 2× above |
apoE+/+ (↓ 4×) |
|
|
|
|
|
|
|
apoE+/+ |
|
Van Eck et al. [181] apoE+/+ |
apoE−/− |
h-apoE2 (14%) |
C |
No change |
apoE+/+ (↓ 87%) |
apoE+/+ (↓ 23×) |
h-apoE2 |
|
h-apoE3-Leiden |
|
|
h-apoE2, h-apoE3-Leiden |
h-apoE2, h-apoE3- |
h-apoE3- |
|
(19%) of apoE+/+ |
|
|
(transient ↓) |
Leiden (no change) |
Leiden |
|
|
|
|
|
|
|
|
|
|
|
|
|
All comparisons made relative to baseline recipient measurements unless stated otherwise. NM, not measured; C, chow diet; A/W, atherosclerotic/Westerntype diet; FC, free cholesterol; h-apoE, human apoE.
*% of WT murine apoE levels.
104 Kathryn E. Naus and Cheryl L. Wellington
atherogenic importance of locally produced macrophage apoE even in the absence of plasma apoE [176, 179, 182].
Conversely, transplantation of apoE−/− bone marrow into atherosusceptible C57B/6 WT recipients creates a model with deletion of apoE solely in macrophages. These mice display no appreciable change in plasma cholesterol levels [183–185], indicating that the selective deficiency of macrophage apoE is not sufficient to affect plasma lipid metabolism when apoE is synthesized normally elsewhere. However, following several weeks on an atherogenic diet, lesion area was increased in WT mice that received apoE−/− bone marrow. Since these differences in lesion areas are seen without changes in plasma cholesterol levels, the protective effects of apoE clearly extend beyond its ability to lower cholesterol [179].
Taken together, these studies strongly support an antiatherogenic role for macrophage apoE. This conclusion is not without a caveat, however, as locally secreted apoE would be predicted to encourage cholesterol influx into macrophages via lipoprotein uptake by apoE receptors [186], and Boisvert et al. [184] found that WT mice reconstituted with apoE-producing macrophages had increased lesions compared to those with apoE−/− macrophages. This unexpected result may be due to differences in gender, diet, or feeding schedules compared to other similarly designed BMT experiments. However, the majority of BMT experiments strongly support an atheroprotective role for macrophage apoE, wherein even low levels of this apolipoprotein are capable of altering the atherosclerotic fate of the animals.
Macrophage ABCA1 and Atherosclerosis
Using BMT experiments, much insight has been gained about the importance of ABCA1 in the macrophage (Table 6.2). Like humans, mice with a complete disruption of ABCA1 have decreased levels of plasma total cholesterol and HDL [102, 103]. However, selective deficiency of macrophage ABCA1 does not decrease plasma lipid or apoAI levels, and conversely, reconstitution of ABCA1−/− animals with WT bone marrow does not restore plasma HDL levels, although very small increases in plasma HDL and apoAI levels have been observed [187]. These studies show that macrophage ABCA1 has only a minimal impact on the circulating pool of mature plasma HDL.
Breeding ABCA1−/− mice to either the apoE−/− or LDLR−/− background was found to reduce plasma lipids in both models on both a chow and high fat high cholesterol diet, as expected due to the role of ABCA1 in HDL regulation [188]. Unexpectedly, however, aortic lesions in ABCA1−/−/apoE−/− mice did not advance beyond fatty streaks on either a chow or an atherogenic diet despite the presence of severe xanthomas and foam cells in the skin [188]. Although more complex lesions were observed in the LDLR−/− compared to the apoE−/− model, lesion size did not differ between LDLR−/− mice that contained or lacked total ABCA1 [188]. These findings suggested that the less atherogenic plasma lipid profile observed in the complete absence of ABCA1

TABLE 6.2. Effect of macrophage ABCA1 on atherosclerosis.
|
|
Recipient |
Plasma apoE |
Plasma apoAI |
|
mΦ Cholesterol |
Total |
|
Group |
Donor |
(sex) |
(% of WT)* |
(% of WT)* |
Diet |
efflux |
cholesterol |
Mean lesion area |
Haghpassand |
ABCA1+/+ |
WT ( ) |
NM |
No change |
C |
ABCA1−/− |
ABCA1+/+ (↑19%) |
NM |
et al. [187] |
ABCA1−/− |
|
|
|
|
(↓ 7%) |
ABCA1−/− (↑ 24%) |
|
|
|
|
|
|
A/W |
|
HDL-C: |
|
|
|
|
|
|
|
|
ABCA1+/+ (↑2×) |
|
|
|
|
|
|
|
|
ABCA1−/− (no change) |
|
|
ABCA1+/+ |
ABCA1−/− |
NM |
ABCA1−/− |
C |
ABCA1−/− |
ABCA1+/+ (↑ 20%) |
NM |
|
ABCA1−/− |
|
|
(↓ 50%) |
|
(↓11%) |
ABCA1−/− (↑ 45%) |
|
|
|
|
|
|
A/W |
|
No change in HDL-C |
|
Aiello et al. |
apoEABCA 1−/− apoE−/− |
NM |
NM |
C |
NM |
No change in |
apoEABC A1−/− |
|
[188] |
apoE−/− |
|
|
|
|
|
HDL-C |
↑ 3.3× above apoE−/− |
Van Eck et al. |
ABCA1 +/+ |
LDLR−/− |
ABCA1−/− |
ABCA1−/− |
C |
ABCA1−/− |
ABCA1−/− (↓ 14%; |
|
[189] |
ABCA1−/− |
|
(↓ 56%) |
(↓ 24%) |
|
(↓ 4%) |
TG ↑ 74%) |
|
|
|
|
|
|
A/W |
|
ABCA1−/− ↓ 1.4× below |
ABCA1−/− (↑ 1.6×) |
|
|
|
|
|
|
|
ABCA1+/+ |
|
|
|
|
|
|
|
|
|
|
All comparisons made relative to baseline recipient measurements or animals reconstituted with WT marrow (unless stated otherwise). NM, not measured; C, chow diet; A/W, atherosclerotic/Western-type diet; TG, triglycerides.
106 Kathryn E. Naus and Cheryl L. Wellington
may have counterbalanced the proposed proatherogenic effect of macrophage ABCA1 deficiency, resulting in no net change in lesion size. To specifically test the influence of macrophage ABCA1 on atherosclerosis, Van Eck et al. reconstituted apoE−/− mice with apoE−/−/ABCA1−/− bone marrow, and observed no change in plasma lipids but a significant increase in lesion area [188]. Similarly, reconstitution of LDLR−/− mice with ABCA1−/− bone marrow had no effect on plasma HDL levels, but resulted in an increased lesion area as well as the presence of more advanced lesions [189]. These studies clearly demonstrated that selective deficiency of macrophage ABCA1 leads to greater development of atherosclerotic lesions, but this effect is independent of circulating HDL.
Thus far, there are conflicting reports on whether overexpression of ABCA1 throughout the body protects from atherosclerosis. Overexpression of ABCA1 under the control of the apoE promoter in atherosusceptible C57Bl/6 mice resulted in decreased atherosclerosis when challenged with a proatherogenic diet [190]. However, these mice unexpectedly developed increased lesions when crossed onto the apoE−/− model [190]. In contrast, Singaraja et al. [69] demonstrated that ABCA1 BAC transgenic mice, which express excess ABCA1 under endogenous regulatory signals, exhibit dramatically reduced lesion size when crossed onto the apoE−/− background. These observations demonstrate that appropriate physiological regulation of ABCA1 is crucial for its antiatherogenic effects in vivo. Whether selective overexpression of appropriately regulated ABCA1 in macrophages is sufficient to protect from atherosclerotic lesion development remains to be determined.
Conclusions
Macrophages play crucial roles in the development of atherosclerosis. One vital aspect of macrophage physiology in atherosclerosis is the efflux of cholesterol accumulated during the uptake of oxidized lipoproteins. Failure of macrophages to rid themselves of accumulated cholesterol is a critical step in lesion progression. Within the context of the vessel wall, local synthesis and lipidation of apoE may also enhance the efficiency of cholesterol efflux from macrophages, and thus retard foam cell formation. In this review, we have highlighted aspects of ABCA1, ABCG1, and apoE function within macrophages and their roles in cholesterol efflux and atherosclerosis. However, many questions remain. For example, ABCA1 is required for efficient secretion of apoE from macrophages [191], and Van Eck et al. [189] have shown that plasma apoE and apoAI levels are reduced by the lack of macrophage ABCA1, providing further evidence that macrophage ABCA1 can affect the metabolism of apoE in vivo. Because macrophage-secreted apoE may have an important role in facilitating cholesterol efflux within the vessel wall, it is possible that ABCA1 may also affect the ability of endogenous apoE to promote RCT under conditions of minimal exposure to plasma
Chapter 6. ABC Transporters and Apolipoprotein E |
107 |
HDL or apoAI [157, 158, 160, 161, 192]. Although the experiments of Singaraja et al. [69] clearly demonstrated that apoE is not required for ABCA1 to promote cholesterol efflux and reduce lesion progression in vivo, these findings do not rule out the possibility that a greater reduction in lesion size or progression may be observed if ABCA1 is overexpressed in the presence of macrophage apoE, particularly if overexpression of ABCA1 is itself restricted to macrophages. Additionally, although Huang et al. [193, 194] have shown that cholesterol efflux in apoE-expressing J774 cells does not depend on ABCA1 but rather is modulated by SRBI expression, ABCA1 and ABCG1 do affect apoE secretion and spontaneous apoE-dependent lipid efflux in human monocyte-derived macrophages [146, 191]. These studies highlight the importance of further investigation into the interplay of the efflux pathways mediated by ABCA1, ABCG1, apoE, and SRBI in relevant model systems. Studies using recently described ABCG1-deficient mice should soon reveal whether or not ABCG1 participates in atherosclerotic lesion development. Because ABCG1 has been reported to be upregulated in ABCA1-deficient macrophages [145], it will also be important to determine the extent to which compensatory gene expression pathways may contribute to macrophage cholesterol efflux and atherosclerosis in vivo. Through these and other investigations, a deeper understanding of how the functions of all of these molecules are integrated within the macrophage may lead to novel methods to preserve optimal macrophage function within the arterial wall and reduce the burden of atherothrombotic disease.
Acknowledgments: We are grateful to the colleagues who have provided critical feedback on this review, including Veronica Hirsch-Reinshagen, Luis Maia, Marcia MacDonald, and Liam Brunham. CLW is supported by a New Investigator Award from the Canadian Institutes of Health Research (CIHR) and operating grants from CIHR and the Heart and Stroke Foundation of Canada.
References
1.Murray CJL, Lopez AD: Alternative projections of mortality and disability by cause 1990–2020: Global Burden of Disease Study. Lancet 349: 1498–1504, 1997.
2.Murray CJL, Lopez AD: Global mortality, disability, and the contribution of risk factors: Global Burden of Disease Study. Lancet 349: 1436–1442, 1997.
3.Gordon T, Kannel WB, Castelli WP, Dawber TR: Lipoproteins, cardiovascular disease and death. Arch Intern Med 141: 1128–1131, 1981.
4.Gordon DJ, Rifkind BM: High density lipoprotein—the clinical implications of recent studies. N Engl J Med 321: 1311–1316, 1989.
5.Hodis HN, Mack WJ: Triglyceride-rich lipoproteins and progression of atherosclerosis. Eur Heart J 19: A40–A44, 1998.