
Учебники / Gene Therapy of Cochlear Deafness - Present Concepts and Future Aspects Ryan 2009
.pdfshown to deliver the reporter genes β-gal and GFP to cells in the spiral ligament, spiral limbus, Reissner’s membrane, and spiral ganglia in the mouse [61], as well as outer hair cells and supporting cells in the organ of Corti in the guinea pig [23], they will have to overcome problems of limited transgene expression time, cell targeting, and inefficient DNA transfer to play a major role in inner ear gene transfer.
Applications of Gene Transfer
Gene therapy has been investigated in four fundamental areas of inner ear dysfunction: (1) introduction of protective factors to enhance SGC survival after hair cell loss; (2) introduction of protective factors to enhance hair cell survival in the face of ototoxic insults, both chemical and noise-induced; (3) hereditary hearing loss with the goal of using gene therapy to transform cells from one phenotype to another or replace/silence a dominant negative mutation that causes hearing loss, and (4) functional hair cell regeneration.
Spiral Ganglion Cell Survival
Hair cell loss results in loss of trophic factors such as neurotrophin-3 (NT-3) and brain-derived neurotrophic factor (BDNF) that maintain the survival and functional integrity of their corresponding SGCs [62, 63]. Since SGC survival is critical for cochlear implant performance, interventions directed at enhancing SGC performance may provide valuable adjuvant therapy for patients with severe-profound hearing loss. Direct infusion of NT-3 and BDNF into the cochlea significantly enhances SGC survival [64], but continuous infusion via an osmotic mini-pump [11] is impractical and offers only a short-term solution.
Gene transfer of a vector-neurotrophin complex offers a potential means of promoting long-term SGC survival after hair cell loss. A replication-defective HSV-1 vector carrying the human BDNF gene drove expression of human BDNF mRNA in murine fibroblasts and elicited neuritic process outgrowth in explanted spiral ganglia cells [35]. In vivo studies have supported both an HSV-BDNF vector [17] and an AAV-BDNF vector [16] to promote the survival of SGCs after an aminoglycosidedeafening regimen. A third viral vector, adenovirus-BDNF, enhanced SGC survival following a deafening regimen in guinea pigs [65].
Glial cell line-derived eurotrophic factor (GDNF) is yet another paracrine factor shown to be important in the survival of inner ear auditory neurons [7, 66]. Both direct application of GDNF in cultured dissociated cochlea treated with cisplatin or neomycin, and in vivo infusion of GDNF (both into the cochlea as well as through the tympanic membrane into the middle ear) before an ototoxic deafening regimen promoted SGC survival in the guinea pig [66]. Intracochlear GDNF infusion also protected SGCs from degeneration after noise trauma [7]. Gene therapy with an aden- ovirus-GDNF vector (Ad-GDNF) enhanced SGC survival in the guinea pig deafened
74 |
Kesser · Lalwani |
by an aminoglycoside/diuretic regimen [18], and Ad-GDNF gene therapy plus electrical stimulation preserved SGCs significantly better than either treatment alone [67]. These preliminary studies lay the groundwork for possible adjunctive therapy for patients with severe-profound hearing loss who benefit from cochlear implantation.
Hair Cell Protection
The same trophic factors that enhance SGC survival have also been shown to protect hair cells, including NT-3 [68], BDNF [68, 69], and GDNF [70, 71]. Gene therapy, using an adenoviral vector carrying the human GDNF gene, can protect vestibular hair cells and rescue cochlear hair cells and hearing function from gentamicininduced ototoxicity [5, 14].
Iron-chelating agents have shown promise as protective factors in aminoglycosideinduced hearing loss [72, 73]. While a vector-iron chelating agent complex has not yet been studied, antioxidant gene therapy using adenoviral vectors to overexpress the enzymes catalase and Mn superoxide dismutase afforded protection of hair cells and hearing when delivered 5 days before a deafening regimen of systemic kanamycin and ethacrynic acid [13].
Iron chelators and reactive oxygen species have also been postulated to play a role in noise-induced hearing loss. Iron chelators (deferoxamine, mannitol), free radical scavengers (mannitol, glutathione, ebselen), as well as neurotrophic factors have been shown to attenuate cochlear damage caused by acoustic trauma [8, 9, 68, 71, 74, 75]. As viral vectors are engineered to contain genes that express free radical scavengers, another strategy for hair cell protection will most likely be validated.
Hair Cell Regeneration
Many of the genes and factors that govern cell fate determination in the sensory epithelium of the inner ear have been elucidated. Math1 (mouse atonal homolog 1), a basic helix-loop-helix transcription factor, is essential for the generation of inner ear hair cells [76]. Using a plasmid vector in vitro, Zheng and Gao [77] induced overexpression of Math1 and observed ectopic hair cell production. These same researchers used an adenoviral vector with the human atonal homolog 1 (Hath1) gene to generate a large number of hair cells in the sensory epithelium of cultured rat utricular maculae [78]. New hair cells were also observed with adenovirus-mediated overexpression of Hath1 following aminoglycoside injury [78].
Gene transfer with an adenoviral vector containing the Math1 insert has been shown to infect supporting cells and generate new hair cells following a deafening regimen in an adult guinea pig model [1]; the newly generated hair cells appear to be functional, as Ad-Math1-infected guinea pigs demonstrated increased hair cell counts and lower thresholds on ABRs relative to untreated, deafened controls [2]. An adenoviral vector with the Math1 insert also drove expression of new hair cells in the macular organs of the aminoglycoside-treated mouse both in vitro and in vivo resulting in recovery of balance function [79].
Inner Ear Gene Therapy and Stem Cell Transplantation |
75 |

Table 2. A sample of potential genetic targets for gene transfer
Gene |
Gene product |
Function |
Location |
Mouse model |
Syndromic |
Nonsyndro- |
Reference |
symbol |
|
|
|
|
locus |
mic locus |
|
|
|
|
|
|
|
|
|
MYO7A |
Myosin VIIa |
Intracellular |
11q12.3 |
Shaker-1 |
USH1B |
DFNA11, B2 |
Self et al. [125], |
|
|
motor |
|
|
|
|
1998 |
|
|
|
|
|
|
|
Weilet al. [80], |
|
|
|
|
|
|
|
1995 |
|
|
|
|
|
|
|
|
USH1C |
Harmonin |
Scaffold |
11p15.1 |
Deaf circler |
USH1C |
DFNB18 |
Verpy |
|
|
protein |
|
|
|
|
et al. [88], |
|
|
|
|
|
|
|
2000 |
|
|
|
|
|
|
|
|
CDH23 |
Cadherin 23 |
Intercellular |
10q21-q22 |
Waltzer |
USH1D |
DFNB12 |
Di Palma |
|
|
adhesion |
|
|
|
|
et al. [84], |
|
|
|
|
|
|
|
2001 |
|
|
|
|
|
|
|
Bork et al. |
|
|
|
|
|
|
|
[82], 2001 |
|
|
|
|
|
|
|
|
PCDH15 |
Protocadherin |
Intercellular |
10q21 |
Ames waltzer |
USH1F |
DFNB23 |
Alagramam |
|
15 |
adhesion |
|
|
|
|
et al. [85], |
|
|
|
|
|
|
|
2001 |
|
|
|
|
|
|
|
Ahmed |
|
|
|
|
|
|
|
et al. [87], |
|
|
|
|
|
|
|
2003 |
|
|
|
|
|
|
|
|
SANS |
SANS |
Scaffold |
17q24-q25 |
Jackson circler |
USH1G |
|
Weil et al. [89], |
|
|
protein |
|
|
|
|
2003 |
|
|
|
|
|
|
|
|
MYO6 |
Myosin VI |
Intracellular |
6q13 |
Snell’s waltzer |
|
DFNA22, B37 |
Ahmed et al. |
|
|
motor |
|
|
|
|
[87], 2003 |
|
|
|
|
|
|
|
Melchionda |
|
|
|
|
|
|
|
et al. |
|
|
|
|
|
|
|
[124], 2001 |
|
|
|
|
|
|
|
|
KCNQ1 |
KCNQ1 |
Potassium |
11p15.5 |
|
JLNS1 |
|
Neyroud |
|
|
channel |
|
|
|
|
et al. [90], |
|
|
|
|
|
|
|
1997 |
|
|
|
|
|
|
|
|
KCNE1 |
KCNE1 |
Potassium |
21q22.1- |
|
JLNS2 |
|
Schulze-Bahr |
|
|
channel |
q22.2 |
|
|
|
et al. [91], |
|
|
|
|
|
|
|
1997 |
|
|
|
|
|
|
|
|
MYO15 |
Myosin 15 |
Intracellular |
17p11.2 |
Shaker-2 |
|
DFNB3 |
Probst et al. |
|
|
motor |
|
|
|
|
[127], 1998 |
|
|
|
|
|
|
|
Wang et al. |
|
|
|
|
|
|
|
[128], 1998 |
|
|
|
|
|
|
|
|
For a complete list, see the Hereditary Hearing Loss Homepage: http://webhost.ua.ac.be/hhh.
76 |
Kesser · Lalwani |
Genetic Hearing Loss
Targeted gene therapy/gene rescue has the potential to replace a defective gene with the wild-type allele. Homologous mouse models of human diseases offer the ideal substrates for experimental gene transfer. These mouse models have also provided invaluable insight into normal cochlear physiology.
Mouse models of Usher’s syndrome, an autosomal recessive condition characterized by sensorineural hearing loss, vestibular deficits, and progressive blindness secondary to retinitis pigmentosa, have revealed many of the proteins and genes, including myosin VIIa [80, 81] , cadherin 23 [82–84], protocadherin 15 [85–87], harmonin [88], and SANS [89], responsible for hair bundle and stereocilia development and organization. Each of these genes is a potential target for gene therapy (table 2).
Defects in potassium channel proteins KCNE1, KCNQ1 (Jervell and Lange-Nielsen syndrome) [90, 91], and KCNQ4 (DFNA2) [3] have also been implicated in hereditary hearing loss. Whereas defects in KCNQ1 alter endolymph secretion, KCNQ4, localized to the basolateral surface of outer hair cells [92] has been implicated in outer hair cell degeneration by abolishing an outward potassium channel current causing chronic depolarization [93]. An adenoviral vector containing the wild-type KCNQ4 gene insert has been shown to transfect cultured human vestibular tissue and drive expression of the wild-type potassium channel KCNQ4, proof of principle that adenoviral vectors can transfect human inner ear epithelium and drive expression of functionally relevant genes [47] (fig. 4).
Gene rescue in cases of mutant phenotypes and gene knockout in cases of dominant negative mutations represent potential applications of gene therapy in genetic hearing loss. Mutations in GJB2, a gene encoding connexin 26, another ion channel protein, cause DFNB1 [4], the most common genetic hearing loss. GJB6 (DFNB1) [94] also encodes a gap junction protein known as connexin 30. With the recent report that connexin 26 expressed from extra alleles delivered via a modified bacterial artificial chromosome in deaf Cx30–/– mice completely restored hearing sensitivity [95], both GJB2 and GJB6 represent important targets for gene therapeutic intervention.
Stem Cell Applications
Cell transplantation or cell replacement therapy with stem cells represents a second major strategy for restoring hearing following hair cell loss. Stem cells are self-renew- ing pluripotent cells that can give rise to any cell in the organism depending on the microenvironment in which the stem cells reside. Using the appropriate cytokines and growth factors, stem cells can be developed into highly specialized cells that may one day replace lost, damaged, or defective human cells, including dopaminergic cells for Parkinson’s disease [96] or pancreatic islet cells for diabetes [97]. For hair cell
Inner Ear Gene Therapy and Stem Cell Transplantation |
77 |
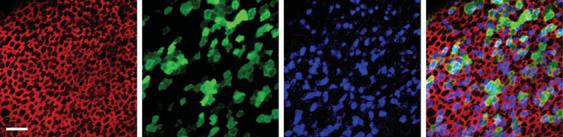
a |
|
b |
|
c |
|
d |
Fig. 4. Confocal images of a saccule harvested from a patient undergoing labyrinthectomy for Ménière’s disease. The explant culture was exposed to 2.3 × 107 viral particles/ml of Ad2-GFP.KCNQ4 for 24 h, fixed and stained with Alexa Fluor 546 phalloidin (red) and KCNQ4 (blue) and GFP (green). The scale bar represents 20 μm and applies to all images. The same field and focal plane are shown throughout. a An image of the apical surface of the epithelium showing the actin that rings each cell. b GFP-positive supporting cells and hair cells are evident in this field. c The KCNQ4 antibody labeled both endogenous hair cell KCNQ4 and exogenous virally transfected KCNQ4 in hair cells and supporting cells. d Merge of panels a–c shows colocalization of GFP and KCNQ4 in 78.6% of the cells. Reprinted from Kesser et al. [47].
loss in the inner ear, a renewable source of progenitor cells that can differentiate into functioning hair cells with the appropriate connections to their corresponding SGCs may restore sensorineural hearing loss. Inner ear hair cells can be generated from three possible stem cell sources: embryonic stem cells, adult inner ear stem cells, and neural stem cells.
Embryonic or Neonatal Stem Cells
In a stepwise fashion using epidermal growth factor and insulin-like growth factor 1 followed by basic fibroblast growth factor in vitro, Li et al. [98] were able to induce three separate lines of mouse embryonic stem cells into inner ear ‘enriched progenitor cells’. Following the withdrawal of these growth factors, the progenitor cells further differentiated into mature cell types expressing hair cell markers myosin 7a, espin, parvalbumin 3, and α9-acetylcholine receptor along with markers for Math1, Brn3.1, and Jagged-1, indicative of maturing sensory epithelia; these cells adopted a hair cell phenotype when grafted into the otic vesicle of chicken embryos [98]. Further refinements to their culture techniques, as well as proof of principle that human embryonic stem cells can differentiate into cells expressing similar inner ear markers have more recently been made [99].
The newborn mouse cochlea may also be a source of inner ear stem cells as it contains highly proliferative progenitor cells that are capable of forming spheres, colonies of monoclonal cells generated from a single stem cell. These sphere-form- ing cells express genes that are indicative of inner ear progenitor cells and have the capacity to differentiate to form cells that express hair cell markers (myosin 7a and espin) in culture [100] and express functional ion channels similar to those seen
78 |
Kesser · Lalwani |
in early hair cells [101]. These same researchers found that the ability for sphere formation in the mouse cochlea decreases about 100-fold during the 2nd and 3rd postnatal weeks, coincident with the onset of hearing; in contrast, expression of progenitor cell markers in the vestibular system remained constant during this period [101].
Adult Stem Cells
The adult mouse inner ear may be another source of inner ear progenitor cells; cells from the adult mouse utricular epithelium have been shown to form spheres that express marker genes of the developing inner ear and have the capacity for selfrenewal. These inner ear stem cells were found to be pluripotent and can give rise to cell types of ectodermal, endodermal, and mesodermal lineages [102].
Stem cells from a different organ system, the central nervous system, may provide inner ear progenitors for both hair cell regeneration [103] as well as replacement of spiral ganglion neurons [103–106]. Not only do neural stem cells survive transplantation into the native and injured mammalian inner ear [103–105, 107], but these engrafted cells may have functional implications by sending neuritic processes to the organ of Corti to replace injured SGCs [106] or by providing trophic support to residual SGCs after hair cell loss [104], as GDNF and BDNF expression have been observed in transplant-derived cells [108]. Neural stem cells were also protective in a cochlear ischemia-reperfusion model [109].
Preliminary work with bone marrow stromal cells or mesenchymal cells also shows these cells to be potential candidate stem cells to replace hair cells [110]. When Math1 is overexpressed in culture with these cells, or if mouse mesenchymal stem cell-derived cells were cocultured with embryonic chick inner ear cells, expression of the hair cell marker proteins myosin 7a, espin, and Brn3c, p27Kip, and jagged2 was induced [111].
Stem Cell Transplantation
In vivo techniques for introducing stem cells into the inner ear and minimizing surgical trauma range from round window injection [112] to lateral semicircular canal injection [113], cochlear lateral wall injection [103] (which did result in hearing loss) [113], injection at the posterior semicircular canal [114], at the internal auditory meatus [115], and at the modiolus [107]. Grafted cells tended to congregate at sites of injury [98]. Given the varied access points to the inner ear, the injection site and method for clinical trials will be most likely tailored to the individual patient, the hearing loss, and goal of the stem cell transplantation.
While these preliminary studies are promising for the future of stem cell therapy in the inner ear, several important questions remain unanswered. Are the hair cells generated by stem cell transplantation functional? If so, will they be able to form the appropriate neural pathways? Does hair cell morphology matter – can a single stem cell induce the differentiation of the appropriate size and shape hair cell
Inner Ear Gene Therapy and Stem Cell Transplantation |
79 |
along the entire length of the cochlea? What are the immunologic implications of grafting stem cells – will the recipient immune response destroy the engrafted cells? The cochlea and brain are immunologically privileged sites; thus far no significant immune response has been noted in in vivo stem cell studies [103, 105, 107] including xenografts of mouse embryonic stem cells transplanted into guinea pig cochleae [104]. Can stem cells in the inner ear induce neoplasm formation? While sphereforming cells harvested from the postnatal mouse organ of Corti can give rise to cells that express functional ion channels suggestive of nascent hair cells [101], it remains to be seen whether these inner ear progenitor cells can induce hair cells that have functional consequences, including lowering ABR thresholds after a deafening regimen, in much the same way adenoviral gene therapy with Math1 has been shown [2].
Future Work
Challenges for the future of gene therapy include refining vectors to improve transduction efficiency and cell targeting, refining methods of gene delivery to minimize trauma to the inner ear while ensuring widespread transfection of the whole cochlea or whole inner ear [116], and discovering new genes such as Myo15a that can be employed to restore function [117].
Cell-specific transgene expression using different viral serotypes and specific promoters will improve transduction efficiency and cell targeting [50, 118–120]. Use of non-viral vectors for delivery of therapeutic molecules into the cochlea using transplanted cells (cell-gene therapy) offers another approach to introducing genetic material to change cell behavior in the inner ear [121]. Gene-silencing techniques using small interfering RNAs have also shown promise in autosomal dominant nonsyndromic hearing loss. A potent GJB2-targeting short interfering RNA has been identified that post-transcriptionally silences the expression of the R75W allele variant of GJB2 in cultured mammalian cells; prevention of hearing loss in GJB2 autosomal dominant deafness was achieved by using this short interfering RNA in the mouse model [122].
With the discovery that human vestibular epithelia can be harvested, cultured, and transfected with an engineered, replication-deficient adenovirus, human hair cells can now be accessed for experimental manipulation [47]. The development of this in vitro model system to study gene transfer and, potentially, stem cell transplantation in human inner ear tissue opens the door for exciting research in the human inner ear. This platform can be used to test viral vectors and their transgenes, neurotrophic factors, cell-signaling proteins, transcription factors, stem cell candidates, and other pharmacologic agents designed to restore function to the inner ear.
80 |
Kesser · Lalwani |
References
1Kawamoto K, Ishimoto S, Minoda R, Brough DE, Raphael Y: Math1 gene transfer generates new cochlear hair cells in mature guinea pigs in vivo. J
Neurosci 2003;:23:4395–4400.
2 Izumikawa M, Minoda R, Kawamoto K, Abrashkin KA, Swiderski DL, Dolan DF, Brough DE, Raphael Y: Auditory hair cell replacement and hearing improvement by Atoh1 gene therapy in deaf mammals. Nat Med 2005;11:271–276.
3 Kubisch C, Schroeder BC, Friedrich T, Lütjohann B, El-Amraoui A, Marlin S, Petit C, Jentsch TJ: KCNQ4, a novel potassium channel expressed in sensory outer hair cells, is mutated in dominant deafness. Cell 1999;96:437–446.
4 Kelsell DP, Dunlop J, Stevens HP, Lench NJ, Liang JN, Parry G, Mueller RF, Leigh IM: Connexin 26 mutations in hereditary non-syndromic sensorineural deafness. Nature 1997;387:80–83.
5 Kawamoto K, Yagi M, Stover T, Kanzaki S, Raphael Y: Hearing and hair cells are protected by adenoviral gene therapy with TGF-beta1 and GDNF. Mol Ther 2003;7:484–492.
6 Yagi M, Magal E, Sheng Z, Ang KA, Raphael Y: Hair cell protection from aminoglycoside ototoxicity by adenovirus-mediated overexpression of glial cell line-derived neurotrophic factor. Hum Gene Ther 1999;10:813–823.
7 Ylikoski J, Pirvola U, Virkkala J, Suvanto P, Liang XQ, Magal E, Altschuler R, Miller JM, Saarma M: Guinea pig auditory neurons are protected by glial cell line-derived growth factor from degeneration after noise trauma. Hear Res 1998;124:17–26.
8 Yamasoba T, Schacht J, Shoji F, Miller JM: Attenuation of cochlear damage from noise trauma by an iron chelator, a free radical scavenger and glial cell line-derived neurotrophic factor in vivo. Brain Res 1999;815:317–325.
9 Yamasoba T, Pourbakht A, Sakamoto T, Suzuki M: Ebselen prevents noise-induced excitotoxicity and temporary threshold shift. Neurosci Lett 2005;380: 234–238.
10 Yamasoba T, Nuttall AL, Harris C, Raphael Y, Miller JM: Role of glutathione in protection against noiseinduced hearing loss. Brain Res 1998;784:82–90.
11 Staecker H, Kopke R, Malgrange B, Lefebvre P, Van de Water TR: NT-3 and/or BDNF therapy prevents loss of auditory neurons following loss of hair cells. Neuroreport 1996;7:889–894.
12 Holt JR: Viral-mediated gene transfer to study the molecular physiology of the Mammalian inner ear. Audiol Neurootol 2002;7:157–160.
13 Kawamoto K, Sha SH, Minoda R, Izumikawa M, Kuriyama H, Schacht J, Raphael Y: Antioxidant gene therapy can protect hearing and hair cells from ototoxicity. Mol Ther 2004;9:173–181.
14 Suzuki M, Yagi M, Brown JN, Miller AL, Miller JM, Raphael Y: Effect of transgenic GDNF expression on gentamicin-induced cochlear and vestibular toxicity. Gene Ther 2000;7:1046–1054.
15 Cooper LB, Chan DK, Roediger FC, Shaffer BR, Fraser JF, Musatov S, Selesnick SH, Kaplitt MG: AAV-mediated delivery of the caspase inhibitor XIAP protects against cisplatin ototoxicity. Otol Neurotol 2006;27:484–490.
16 Lalwani AK, Han JJ, Castelein CM, Carvalho GJ, Mhatre AN: In vitro and in vivo assessment of the ability of adeno-associated virus-brain-derived neurotrophic factor to enhance spiral ganglion cell survival following ototoxic insult. Laryngoscope 2002; 112:1325–1334.
17 Staecker H, Gabaizadeh R, Federoff H, Van De Water TR: Brain-derived neurotrophic factor gene therapy prevents spiral ganglion degeneration after hair cell loss. Otolaryngology Head Neck Surg 1998; 119:7–13.
18 Yagi M, Kanzaki S, Kawamoto K, Shin B, Shah PP, Magal E, Sheng J, Raphael Y: Spiral ganglion neurons are protected from degeneration by GDNF gene therapy. J Assoc Res Otolaryngol 2000;1:315– 325.
19 Lalwani AK, Walsh BJ, Reilly PG, Muzyczka N, Mhatre AN: Development of in vivo gene therapy for hearing disorders: introduction of adeno-associ- ated virus into the cochlea of the guinea pig. Gene Ther 1996;3:588–592.
20 Kho ST, Pettis RM, Mhatre AN, Lalwani AK: Safety of adeno-associated virus as cochlear gene transfer vector: analysis of distant spread beyond injected cochleae. Mol Ther 2000;2:368–373.
21 Stover T, Yagi M, Raphael Y: Transduction of the contralateral ear after adenovirus-mediated cochlear gene transfer. Gene Ther 2000;7:377–383.
22 Jero J, Mhatre AN, Tseng CJ, Stern RE, Coling DE, Goldstein JA, Hong K, Zheng WW, Hoque AT, Lalwani AK: Cochlear gene delivery through an intact round window membrane in mouse. Hum Gene Ther 2001;12:539–548.
23 Wareing M, Mhatre AN, Pettis R, Han JJ, Haut T, Pfister MH, Hong K, Zheng WW, Lalwani AK: Cationic liposome mediated transgene expression in the guinea pig cochlea. Hear Res 1999;128:61– 69.
Inner Ear Gene Therapy and Stem Cell Transplantation |
81 |

24 Derby ML, Sena-Esteves M, Breakefield XO, Corey DP: Gene transfer into the mammalian inner ear using HSV-1 and vaccinia virus vectors. Hear Res 1999;134:1–8.
25 Dazert S, Aletsee C, Brors D, Gravel C, Sendtner M, Ryan AF: In vivo adenoviral transduction of the neonatal rat cochlea and middle ear. Hear Res 2001; 151:30–40.
26 Praetorius M, Baker K, Weich CM, Plinkert PK, Staecker H: Hearing preservation after inner ear gene therapy: the effect of vector and surgical approach. ORL J Otorhinolaryngol Relat Spec 2003; 65:211–214.
27 Carvalho GJ, Lalwani AK: The effect of cochleostomy and intracochlear infusion on auditory brain stem response threshold in the guinea pig. Am J Otol 1999;20:87–90.
28 Kawamoto K, Oh SH, Kanzaki S, Brown N, Raphael Y: The functional and structural outcome of inner ear gene transfer via the vestibular and cochlear fluids in mice. Mol Ther 2001;4:575–585.
29 Yamasoba T, Yagi M, Roessler BJ, Miller JM, Raphael Y: Inner ear transgene expression after adenoviral vector inoculation in the endolymphatic sac. Hum Gene Ther 1999;1:769–774.
30 Praetorius M, Knipper M, Schick B, Tan J, Limberger A, Carnicero E, Alonso MT, Schimmang T: A novel vestibular approach for gene transfer into the inner ear. Audiol Neurootol 2002;7:324–334.
31 Han JJ, Mhatre AN, Wareing M, Pettis R, Gao WQ, Zufferey RN, Trono D, Lalwani AK: Transgene expression in the guinea pig cochlea mediated by a lentivirus-derived gene transfer vector. Hum Gene Ther 1999;10:1867–1873.
32 Raphael Y, Frisancho JC, Roessler BJ: Adenoviralmediated gene transfer into guinea pig cochlear cells in vivo. Neurosci Lett 1996;207:137–141.
33 Dazert S, Battaglia A, Ryan AF: Transfection of neonatal rat cochlear cells in vitro with an adenovirus vector. Int J Dev Neurosci 1997;15:595–600.
34 Holt JR, Johns DC, Wang S, Chen ZY, Dunn RJ, Marban E, Corey DP: Functional expression of exogenous proteins in mammalian sensory hair cells infected with adenoviral vectors. J Neurophysiol 1999;81:1881–1888.
35 Geschwind MD, Hartnick CJ, Liu W, Amat J, Van De Water TR, Federoff HJ: Defective HSV-1 vector expressing BDNF in auditory ganglia elicits neurite outgrowth: model for treatment of neuron loss following cochlear degeneration. Hum Gene Ther 1996;7:173–182.
36 Di Pasquale G, Rzadzinska A, Schneider ME, Bossis I, Chiorini JA, Kachar B: A novel bovine virus efficiently transduces inner ear neuroepithelial cells. Mol Ther 2005;11:849–855.
82
37 He TC, Zhou S, da Costa LT, Yu J, Kinzler KW, Vogelstein B: A simplified system for generating recombinant adenoviruses. Proc Natl Acad Sci USA 1998;95:2509–2514.
38Luebke AE, Foster PK, Muller CD, Peel AL: Cochlear function and transgene expression in the guinea pig cochlea, using adenovirusand adeno-associated virus-directed gene transfer. Hum Gene Ther 2001;-
12:773–781.
39 Luebke AE, Steiger JD, Hodges BL, Amalfitano A: A modified adenovirus can transfect cochlear hair cells in vivo without compromising cochlear function. Gene Ther 2001;8:789–794.
40 Corey DP, Garcia-Anoveros J, Holt JR, Kwan KY, Lin SY, Vollrath MA, Amalfitano A, Cheung EL, Derfler BH, Duggan A, Géléoc GS, Gray PA, Hoffman MP, Rehm HL, Tamasauskas D, Zhang DS: TRPA1 is a candidate for the mechanosensitive transduction channel of vertebrate hair cells. Nature 2004;432:723–730.
41 Amalfitano A, Parks RJ: Separating fact from fiction: assessing the potential of modified adenovirus vectors for use in human gene therapy. Gene Ther 2002;2:111–133.
42Amalfitano A: Utilization of adenovirus vectors for multiple gene transfer applications. Methods 2004;- 33:173–178.
43 Davidson BL, Allen ED, Kozarsky KF, Wilson JM, Roessler BJ: A model system for in vivo gene transfer into the central nervous system using an adenoviral vector. Nat Genet 1993;3:219–223.
44 Ishimoto S, Kawamoto K, Kanzaki S, Raphael Y: Gene transfer into supporting cells of the organ of Corti. Hear Res 2002;173:187–197.
45 Yamasoba T, Suzuki M, Kondo K: Transgene expression in mature guinea pig cochlear cells in vitro. Neurosci Lett 2002;335:13–16.
46Han D, Yu Z, Fan E, Liu C, Liu S, Li Y, Liu Z: Morphology of auditory hair cells in guinea pig
cochlea after transgene expression. Hear Res 2004;- 190:25–30.
47 Kesser BW, Hashisaki GT, Fletcher K, Eppard H, Holt JR: An in vitro model system to study gene therapy in the human inner ear. Gene Ther 2007;14:- 1121–1131.
48 Lalwani A, Walsh B, Reilly P, Carvalho G, Zolotukhin S, Muzyczka N, Mhatre A: Long-term in vivo cochlear transgene expression mediated by recombinant adeno-associated virus. Gene Ther 1998;5: 277–281.
49 Lalwani AK, Walsh BJ, Carvalho GJ, Muzyczka N, Mhatre AN: Expression of adeno-associated virus integrated transgene within the mammalian vestibular organs. Am J Otol 1998;19:390–395.
Kesser · Lalwani

50 Stone IM, Lurie DI, Kelley MW, Poulsen DJ: Adenoassociated virus-mediated gene transfer to hair cells and support cells of the murine cochlea. Mol Ther 2005;11:843–848.
51 Kaplitt MG, Leone P, Samulski RJ, Xiao X, Pfaff DW, O’Malley KL, During MJ: Long-term gene expression and phenotypic correction using adeno-associ- ated virus vectors in the mammalian brain. Nat Genet 1994;8:148–154.
52Staecker H, Li D, O’Malley BW, Van de Water TR: Gene expression in the mammalian cochlea: a study
of multiple vector systems. Acta Otolaryngol 2001;- 121:157–163.
53 Lalwani AK, Mhatre AN: Cochlear gene therapy. Ear Hear 2003;24:342–348.
54Palu G, Parolin C, Takeuchi Y, Pizzato M: Progress with retroviral gene vectors. Rev Med Virol 2000;- 10:185–202.
55 Gunzburg WH, Fleuchaus A, Saller R, Salmons B: retroviral vector targeting for gene therapy. Cytokines Mol Ther 1996;2:177–184.
56 Gunzburg WH, Salmons B: Development of retroviral vectors as safe, targeted gene delivery systems. J Mol Med 1996;74:171–182.
57 Ailles LE, Naldini L: HIV-1-derived lentiviral vectors. Curr Top Microbiol Immunol 2002;261:31–52.
58 Naldini L, Blomer U, Gage FH, Trono D, Verma IM: Efficient transfer, integration, and sustained longterm expression of the transgene in adult rat brains injected with a lentiviral vector. Proc Natl Acad Sci USA 1996;93:11382–11388.
59 Blomer U, Naldini L, Kafri T, Trono D, Verma IM, Gage FH: Highly efficient and sustained gene transfer in adult neurons with a lentivirus vector. J Virol 1997;71:6641–6649.
60 Leventis R, Silvius JR: Interactions of mammalian cells with lipid dispersions containing novel metabolizable cationic amphiphiles. Biochim Biophys Acta 1990;1023:124–132.
61 Jero J, Tseng CJ, Mhatre AN, Lalwani AK: A surgical approach appropriate for targeted cochlear gene therapy in the mouse. Hear Res 2001;151:106–114.
62 Ernfors P, Van De Water T, Loring J, Jaenisch R: Complementary roles of BDNF and NT-3 in vestibular and auditory development. Neuron 1995;14:- 1153–1164.
63 Morrison RS, Kinoshita Y, Johnson MD, Ghatan S, Ho JT, Garden G: Neuronal survival and cell death signaling pathways. Adv Exp Med Biol 2002;513:41– 86.
64 Miller JM, Chi DH, O’Keeffe LJ, Kruszka P, Raphael Y, Altschuler RA: Neurotrophins can enhance spiral ganglion cell survival after inner hair cell loss. Int J Dev Neurosci 1997;15:631–643.
Inner Ear Gene Therapy and Stem Cell Transplantation
65 Nakaizumi T, Kawamoto K, Minoda R, Raphael Y: Adenovirus-mediated expression of brain-derived neurotrophic factor protects spiral ganglion neurons from ototoxic damage. Audiol Neurootol 2004; 9:135–143.
66 Kuang R, Hever G, Zajic G, Yan Q, Collins F, Louis JC, Keithley E, Magal E: Glial cell line-derived neurotrophic factor. Potential for otoprotection. Ann NY Acad Sci 1999;884:270–291.
67 Kanzaki S, Stover T, Kawamoto K, Prieskorn DM, Altschuler RA, Miller JM, Raphael Y: Glial cell linederived neurotrophic factor and chronic electrical stimulation prevent VIII cranial nerve degeneration following denervation. J Comp Neurol 2002;454:- 350–360.
68 Shoji F, Miller AL, Mitchell A, Yamasoba T, Altschuler RA, Miller JM: Differential protective effects of neurotrophins in the attenuation of noiseinduced hair cell loss. Hear Res 2000;146:134–142.
69 Lopez I, Honrubia V, Lee SC, Chung WH, Li G, Beykirch K, Micevych P: The protective effect of brain-derived neurotrophic factor after gentamicin ototoxicity. Am J Otol 1999;20:317–324.
70 Keithley EM, Ma CL, Ryan AF, Louis JC, Magal E: GDNF protects the cochlea against noise damage. Neuroreport 1998;9:2183–2187.
71 Shoji F, Yamasoba T, Magal E, Dolan DF, Altschuler RA, Miller JM: Glial cell line-derived neurotrophic factor has a dose dependent influence on noiseinduced hearing loss in the guinea pig cochlea. Hear Res 2000;142:41–55.
72Song BB, Schacht J: Variable efficacy of radical scavengers and iron chelators to attenuate gentamicin ototoxicity in guinea pig in vivo. Hear Res 1996;- 94:87–93.
73 Song BB, Anderson DJ, Schacht J: Protection from gentamicin ototoxicity by iron chelators in guinea pig in vivo. J Pharmacol Exp Ther 1997;282:369– 377.
74 Ohinata Y, Yamasoba T, Schacht J, Miller JM: Glutathione limits noise-induced hearing loss. Hear Res 2000;146:28–34.
75 Pourbakht A, Yamasoba T: Ebselen attenuates cochlear damage caused by acoustic trauma. Hear Res 2003;181:100–108.
76 Bermingham NA, Hassan BA, Price SD, Vollrath MA, Ben-Arie N, Eatock RA, Bellen HJ, Lysakowski A, Zoghbi HY: Math1: an essential gene for the generationofinnerearhaircells.Science1999;284:1837– 1841.
77 Zheng JL, Gao WQ: Overexpression of Math1 induces robust production of extra hair cells in postnatal rat inner ears. Nat Neurosci 2000;3:580– 586.
83