
Учебники / Gene Therapy of Cochlear Deafness - Present Concepts and Future Aspects Ryan 2009
.pdfresults were repeated using the human homolog of math1 (hath1) and an adenovector as the delivery vehicle. In that study hair cells were shown to be regenerated in adult mammalian vestibular neuroepithelium in vitro [24]. This demonstrated that both different atonal homologs and different delivery methods are effective at restoring hair cells. Interestingly math1 knockout animals can also be rescued through the delivery of the drosophila atonal gene, demonstrating the degree of conservation in sequence and function of this gene family [25]. Most recently, generation of new and functional auditory hair cells in the guinea pig cochlea was demonstrated using math1 delivered by an adenovector [26, 27]. Transfection of atoh1 in several model systems has been shown to be capable of generating hair cells, suggesting that atonal is a good candidate for further therapeutic development of inner ear disorders where reestablishment of sensory cells can elicit a clinical benefit. Examination of auditory hair cells that result from atonal therapy in vivo have identified some abnormal morphologic features, with cells having the characteristics of outer hair cells and supporting cells. This may suggest that genes that are active earlier in the development of the sensory neuroepithelium function may be useful for restoring the inner ear. Manipulation of cell cycle control has also been demonstrated to result in restoration of auditory hair cells [28]. Inhibition of hes1 and hes5 has also been demonstrated to generate supernumerary hair cells [29, 30]. Manipulation of several different development pathways can therefore be used to induce hair cell production. A therapeutic approach that allows the transformation of supporting cells into hair cells may be one effective way of restoring vestibular function.
Delivery of Genes to the Inner Ear
A key element in the development of vestibular hair cell regeneration is developing a method for delivering therapeutic genes to the damaged tissue. Adenovectors are increasingly being used in gene therapy research for the inner ear [for review see, 31]. Adenovectors are nonenveloped viruses containing a linear double-stranded DNA genome. They measure 90 nm in diameter and have a molecular weight of 2,500 kDa. There are over 40 serotype strains of adenovirus, most of which cause benign respiratory tract infections in humans. However, subgroup C serotypes 2 and 5 are predominantly used as vectors. The life cycle does not normally involve integration into the host genome, rather these serotypes replicate as episomal elements in the nucleus of the host cell and consequently there is no risk of insertional mutagenesis.
The wild-type adenovirus genome is approximately 35 kb of which up to 30 kb can be replaced with foreign DNA. There are four early transcriptional units (E1, E2, E3 and E4), which have regulatory functions, and a late transcript which codes for structural proteins. Progenitor vectors have either the E1 or E3 gene inactivated,
54 |
Baker · Brough · Staecker |
with the missing gene being supplied in trans either by a helper virus, plasmid or integrated into a helper cell genome. Second-generation vectors additionally use an E2a temperature-sensitive mutant or an E4 deletion. Adenoviral vectors are very efficient at transducing target cells in vitro and in vivo, and can be produced at high titers (>1011/ml). This is an important factor in inner ear gene therapy where the volume of delivered molecular agent can be limited by the volume of the inner ear. Delivery of excessive volume results in trauma and loss of function [32]. Therefore, when using a vector approach for gene delivery, utilization of a vector that can be concentrated is advantageous. We have evaluated a number of different delivery approaches including delivery into the scala tympani via the round window membrane and delivery via the posterior semicircular canal. We have also developed an approach that models human stapedotomy, an approach that may be used for human gene delivery. Using a postauricular approach, the middle ear of adult C57Bl6 mice was exposed. The stapedial artery was followed and the stapes and oval window identified. Using an argon laser, a fenestration into the vestibule was created at the edge of the footplate, thereby avoiding injury to the stapedial artery (fig. 1a). After fenestrating the footplate, hyaluronic acid gel (Sepragel™, Gyrus, Inc.) was used to cover the fenestration to prevent egress of perilymph. Using a microinjector, 1 × 107 PU of E1/E3/E4-deleted adenovector that expressed green fluorescent protein (GFP) was injected into the vestibule. As seen in figure 1, expression of GFP can be seen throughout the inner ear including outer hair cells, and in the vestibular neuroepithelium.
Evaluation of Gene Delivery to Damaged Macular Neuroepithelium
In order to validate the efficacy of adenovector-mediated gene delivery to damaged vestibular neuroepithelium, we treated mouse macular organ cultures with neomycin 10–3 m for 48 h. These explants were then challenged with increasing doses of an E1/E3/E4-deleted adenovector that expressed GFP driven by a human cytomegalovirus (hCMV) promoter. GFP expression in these cultures was similar to explants that were not treated with aminoglycosides and then challenged with a similar dose of adenovector. As seen in figure 2, delivery of increasing doses of vector to damaged macular organ culture result in increasing numbers of cells expressing GFP. Delivery of 5 × 106 PU of vector to macular organs resulted in GFP expression in a larger percentage of the damaged macular epithelium, suggesting that adenovector can be concentrated to a high enough degree to saturate the targeted epithelium.
To obtain more quantitative data, these experiments were repeated with a vector that expressed luciferase. Determination of the level of luciferase activity in the explants allowed an exact measure (per μg of protein) of total transfection efficiency per experimental condition. As seen in figure 3, there was no significant long-term
Vestibular System Gene Therapy |
55 |
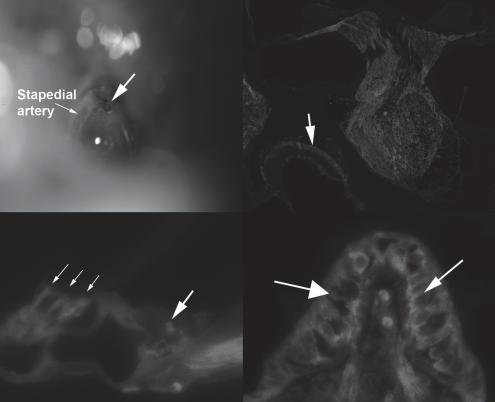
a |
b |
c |
d |
Fig. 1. To evaluate the efficacy of adenovector-mediated gene delivery in the inner ear, we developed a mouse model of a delivery approach that could potentially be used in humans. After exposing the middle ear, the mouse stapes footplate was fenestrated using an argon laser (a). Injection of an advanced generation adenovector that expressed gfp via this approach resulted in broad distribution of gfp expression throughout the inner ear (b) including expression in the macular organs (arrow). Analysis of gfp distribution demonstrates expression of gfp in outer hair cells (c; small arrows) and within neurites innervating the inner hair cell (c; large arrow). Within the vestibular system expression of gfp is seen both in vestibular hair cells and supporting cells (d).
difference in transfection per dose of vector between non-aminoglycoside and amin- oglycoside-treated cultures. At early time points neomycin-treated cultures appeared to express a higher level of luciferase, suggesting that damaged neuroepithelium is very amenable to transfection with adenovectors.
Delivery of Atoh1 to the Aminoglycoside-Treated Macular Organ Cultures
We evaluated the effect of adenovector-mediated delivery of atoh1 by transfecting neomycin-treated macular organ explants with an adenovector that expressed
56 |
Baker · Brough · Staecker |
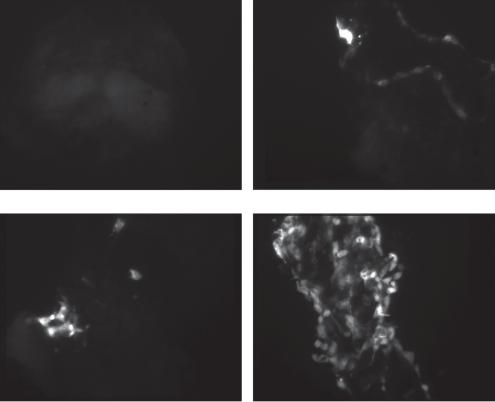
0 PU vector/culture |
1 × 105 PU vector/culture |
1 × 106 PU vector/culture |
5 × 106 PU vector/culture |
Fig. 2. Adult mouse macular organ cultures were pretreated with 0.1 mM neomycin for 48 h and then treated with 1 × 105 to 5 × 106 PU of gfp-expressing adenovector and maintained in vitro. Explants were examined under an inverted fluorescent microscope. Exposing the damaged explants to increasing doses of vector results in increasing numbers of gfp-positive cells.
both atoh1 and gfp (Ad.gfp.atoh1.11d). Two, 5 and 10 days after vector delivery, explants were fixed and serially sectioned. The sections were immunostained with anti-myosin VII. Figure 4a demonstrates that there are no myosin VII-positive cells present, suggesting that neomycin treatment has adequately ablated the hair cell population. The damaged neuroepithelium also broadly expressed GFP, demonstrating effective vector delivery. Myosin VII-positive cells were first seen 10 days after delivery of vector (fig. 4c). Brdu labeling in conjunction with neomycin and atoh1 treatment did not demonstrate the presence of Brdu-labeled nuclei in the restored neuroepithelium (fig. 5), suggesting that the hair cells seen after atoh1 delivery are the product of transdifferentiation rather than a mitotic event. Cultures treated only with neomycin did not show any spontaneous recovery of hair cells [33].
Vestibular System Gene Therapy |
57 |
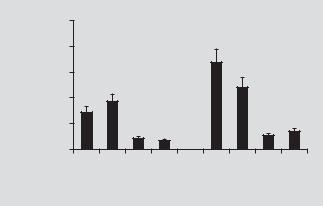
|
250,000 |
|
protein |
200,000 |
|
150,000 |
||
|
||
RLU/µg |
100,000 |
|
50,000 |
||
|
||
|
0 |
Day |
Day |
Day |
Day |
Day |
Day |
Day |
Day |
3 |
8 |
15 |
26 |
3 |
8 |
15 |
26 |
|
Control |
|
|
Neomycin |
|
Fig. 3. Macular organ cultures were treated with luciferase-expressing adenovector or pretreated with neomycin followed by luciferase-expressing adenovector. Three, 8, 15 and 26 days after treatment, luciferase levels per total protein (RLU/μg protein) was determined. During the first week of luciferase expression, the neomycin-treated cultures expressed higher levels of luciferase than control cultures suggesting that damaged vestibular neuroepithelium is efficient. Both control and neo- mycin-treated explants continue to express low amounts of luciferase at 26 days after delivery.
In Vivo Studies to Evaluate the Delivery of Atoh1 into the Inner Ear
We next tested atoh1 overexpression in vivo using a mouse model of vestibular aminoglycoside damage. Unilateral vestibular damage was induced by injecting 2 μl of neomycin (10–3 m) into the inner ear via the round window. This was found to reliably destroy all vestibular hair cells within 48 h. Two days after inducing unilateral vestibular dysfunction mice received an intracochlear injection of Ad.11D.atoh1 via the round window membrane. This delivery method was used due to the ease of surgical access in the mouse and previous documentation of adenovector delivered transgene in the vestibular neuroepithelium [34]. Transmission electron microscopy demonstrated recovery of stereocilia in the atoh1-treated animals, with animals treated only with neomycin showing only microvilli at the apical surface of the neuroepithelium (fig. 6). The effect of atoh1 delivery in neomycin-treated animals was also evaluated using myosin VII immunostaining. Animals were treated with neomycin as described above. Two days after neomycin treatment, 1 × 108 PU Ad.11D.atoh1 was injected into the posterior semicircular canal. Eight weeks after vector delivery the temporal bones were removed and processed for immunohistochemistry. Controls consisted of age-matched untreated controls and animals that had undergone only neomycin treatment and had recovered for 8 weeks. Figure 7 demonstrates that there is good recovery of myosin VII-positive cells (fig. 7c) compared to animals treated only with neomycin (fig. 7b), suggesting that this is an effect of atoh1 rather than spontaneous recovery of the damaged neuroepithelium. To evaluated balance function a second set of animals were swim tested 2 months after aminoglycoside ablation followed by
58 |
Baker · Brough · Staecker |
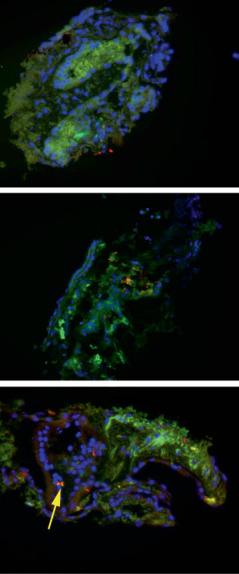
Fig. 4. To track the development of hair cells after math1 delivery, macular organs were treated with neomycin and subsequently transfected with a vector expressing math1 and GFP. Two, 5 and 10 days after vector delivery the maculae were fixed, sectioned and immunostained with antimyosin VII and a rhodaminelabeled secondary antibody. GFP expression is green; the position of nuclei is demonstrated by DAPI stain, and hair cells appear red. GFP production can be noted by day 2 after vector delivery (a), whereas myosin VII-positive cells (arrow) are not seen until 14 days after vector delivery (c). The lack of myosin VII staining seen at earlier time points suggests that the myosin VIIpositive cells are newly produced, rather than the recovery of a damaged hair cell.
a
b
c
no treatment or treatment with Ad.11D.atoh1. Age-matched control animals showed a baseline swim test time of 10 ± 1.4 s. Animals treated only with aminoglycoside showed average swim test times of 22 ± 4.2 s (p < 0.01), suggesting impaired balance compared to controls. Animals treated with aminoglycoside followed by Ad.11D. atoh1, showed swim times of 12 ± 3 s (p = 0.1). This suggests that there was a recovery of balance in the vector-treated animals [33]. Delivery of atoh1 to damaged vestibular epithelium therefore appears to induce the functional recovery of vestibular hair cells.
Vestibular System Gene Therapy |
59 |
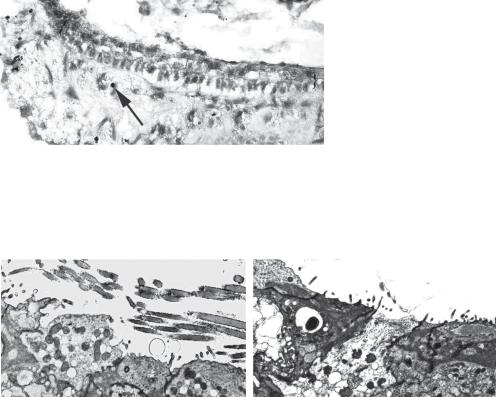
Fig. 5. Brdu labeling of macular organ cultures that have been treated with neomycin and atoh1 demonstrated that there were no Brdu-labeled nuclei within the neuroepithelium (shown between brackets, {}). Several Brdu-labeled nuclei are seen below the neuroepithelium (arrow).
a |
b |
Fig. 6. Transmission electron micrograph of a utricle from an atoh1-treated animal (a) shows the presence of stereocilia, whereas animals treated only with neomycin (b) demonstrate only microvilli in the neuroepithelium. This suggests that in vivo, there is no significant spontaneous recovery of stereocilia-bearing cells.
Conclusion
The last 10 years have seen significant improvements in the efficacy and payload capacity of a variety of vectors. Adenovectors have been widely used and are currently in human clinical trials for gene delivery to the eye, thereby demonstrating that these vectors are safe to use for gene delivery to a neuroepithelium. We have demonstrated that atoh1 delivery is capable of inducing recovery of the vestibular system in a mouse aminoglycoside hair cell loss model. Further development of an adenovector that allows delivery of atoh1 to damaged vestibular epithelium may have the potential of treating a variety of complex disorders such as aminoglycoside vestibulotoxicity. Improving targeting of vectors and utilization of tissue-specific promoters would improve specificity and the efficacy of adenovectors for use as molecular therapeutics for human vestibular hair cell loss.
60 |
Baker · Brough · Staecker |
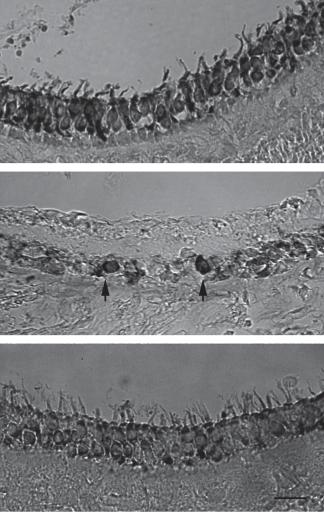
|
a |
Fig. 7. Adult mice were |
|
treated with an intraco- |
|
chlear injection of neomy- |
|
cin into the scala tympani. |
|
After 48 h, an adenovector |
|
that expressed atoh1 was |
|
injected into the posterior |
|
semicircular canal. The ani- |
|
mals were allowed to |
|
recover for 8 weeks. Age- |
b |
matched untreated controls |
|
demonstrated normal dis- |
|
tribution of the hair cell |
|
specific myosin VII (a) in the |
|
macular organs. Animals |
|
injected only with neomy- |
|
cin did not demonstrate |
|
residual myosin VII-positive |
|
cells (b; arrow). Animals |
|
treated with neomycin fol- |
|
lowed by delivery of atoh1 |
|
demonstrated recovery of |
c |
myosin VII-positive cells (c). |
Acknowledgments
This research was supported by NIH grant NIDCD R41 DC 07772 01.
References
1 Proctor L, Perlman H, Lindsay J, Matz G: Acute vestibular paralysis in herpes zoster oticus. Ann Otol Rhinol Laryngol 1979;88:303–310.
2 Tsuji K, Velazquez-Villasenor L, Rauch SD, Glynn RJ, Wall C III, Merchant SN: Temporal bone studies of the human peripheral vestibular system. Ménière’s disease. Ann Otol Rhinol Laryngol Suppl 2000;181: 26–31.
3 Tsuji K, Velazquez-Villasenor L, Rauch SD, Glynn RJ, Wall C III, Merchant SN: Temporal bone studies of the human peripheral vestibular system. Aminoglycoside ototoxicity. Ann Otol Rhinol Laryngol Suppl 2000;181:20–25.
4 Gillespie MB, Minor LB: Prognosis in bilateral vestibular hypofunction. Laryngoscope 1999;109:35– 41.
Vestibular System Gene Therapy |
61 |
5 Dayal VS, Chait GE, Fenton SS: Gentamicin vestibulotoxicity. Long term disability Ann Otol Rhinol Laryngol 1979;88:36–39.
6 Herdman SJ, Blatt P, Schubert MC, Tusa RJ: Falls in patients with vestibular deficits. Am J Otol 2000;21:- 847–851.
7 Rauch SD: Vestibular histopathology of the human temporal bone. What can we learn? Ann NY Acad Sci 2001;942:25–33.
8 Wall C III, Merfeld DM, Rauch SD, Black FO: Vestibular prostheses: the engineering and biomedical issues. J Vestib Res 2002;12:95–113.
9 Kentala E, Vivas J, Wall C III: Reduction of postural sway by use of a vibrotactile balance prosthesis prototype in subjects with vestibular deficits. Ann Otol Rhinol Laryngol 2003;112:404–409.
10 Tyler M, Danilov Y, Bach YR: Closing an open-loop control system: vestibular substitution through the tongue. J Integr Neurosci 2003;2:159–164.
11 Forge A, Li L, Corwin JT, Nevill G: Ultrastructural evidence for hair cell regeneration in the mammalian inner ear. Science 1993;259:1616–1619.
12 Warchol ME, Lambert PR, Goldstein BJ, Forge A, Corwin JT: Regenerative proliferation in inner ear sensory epithelia from adult guinea pigs and humans. Science 1993;259:1619–1622.
13 Tanyeri H, Lopez I, Honrubia V: Histological evidence for hair cell regeneration after ototoxic cell destruction with local application of gentamicin in the chinchilla crista ampullaris. Hear Res 1995;89:194–202.
14 Kelley MW, Talreja DR, Corwin JT: Replacement of hair cells after laser microbeam irradiation in cultured organs of Corti from embryonic and neonatal mice. J Neurosci 1995;15:3013–3026.
15 Lambert PR: Inner ear hair cell regeneration in a mammal: identification of a triggering factor. Laryngoscope 1994;104:701–718.
16 Oesterle EC, Rubel EW: Hair cell generation in vestibular sensory receptor epithelia. Ann NY Acad Sci 1996;781:34–46.
17 Kopke RD, Jackson RL, Li G, Rasmussen MD, Hoffer ME, Frenz DA, Costello M, Schultheiss P, Van De Water TR: Growth factor treatment enhances vestibular hair cell renewal and results in improved vestibular function. Proc Natl Acad Sci USA 2001;98:5886–5891.
18 Zine A, Aubert A, Qiu J, Therianos S, Guillemot F, Kageyama R, de Ribaupierre F: Hes1 and Hes5 activities are required for the normal development of the hair cells in the mammalian inner ear. J Neurosci 2001;21:4712–4720.
19 Shailam R, Lanford PJ, Dolinsky CM, Norton CR, Gridley T, Kelley MW: Expression of proneural and neurogenic genes in the embryonic mammalian vestibular system. J Neurocytol 1999;28:809–819.
62
20 Ben-Arie N, McCall AE, Berkman S, Eichele G, Bellen HJ, Zoghbi HY: Evolutionary conservation of sequence and expression of the bHLH protein Atonal suggests a conserved role in neurogenesis. Hum Mol Genet 2006;5:1207–1216.
21 Ben-Arie N, Hassan BA, Bermingham NA, Malicki DM, Armstrong D, Matzuk M, Bellen HJ, Zoghbi HY: Functional conservation of atonal and Math1 in the CNS and PNS. Development 2000;127:1039– 1048.
22 Bermingham NA, Hassan BA, Price SD, Vollrath MA, Ben Arie N, Eatock RA, Bellen HJ, Lysakowski A, Zoghbi HY: Math1:an essential gene for the generationofinnerearhaircells.Science1999;284:1837– 1841.
23 Zheng JL, Gao WQ: Overexpression of Math1 induces robust production of extra hair cells in postnatal rat inner ears. Nat Neurosci 2000;3:580– 586.
24 Shou J, Zheng JL, Gao WQ: Robust generation of new hair cells in the mature mammalian inner ear by adenoviral expression of Hath1. Mol Cell Neurosci 2003;23:169–179.
25 Wang VY, Hassan BA, Bellen HJ, Zoghbi HY: Drosophila atonal fully rescues the phenotype of Math1 null mice: new functions evolve in new cellular contexts. Curr Biol 2002;12:1611–1616.
26 Kawamoto K, Ishimoto S, Minoda R, Brough DE, Raphael Y: Math1 gene transfer generates new cochlear hair cells in mature guinea pigs in vivo. J Neurosci 2003;23:4395–4400.
27 Izumikawa M, Minoda R, Kawamoto K, Abrashkin KA, Swiderski DL, Dolan DF, Brough DE, Raphael Y: Auditory hair cell replacement and hearing improvement by Atoh1 gene therapy in deaf mammals. Nat Med 2005;11:271–276.
28 Lowenheim H, Furness DN, Kil J, Zinn C, Gultig K, Fero ML, Frost D, Gummer AW, Roberts JM, Rubel EW, Hackney CM, Zenner HP: Gene disruption of p27(Kip1) allows cell proliferation in the postnatal and adult organ of Corti. Proc Natl Acad Sci USA 1999;96:4084–4088.
29 Zine A, Aubert A, Qiu J, Therianos S, Guillemot F, Kageyama R, de Ribaupierre F: Hes1 and Hes5 activities are required for the normal development of the hair cells in the mammalian inner ear. J Neurosci 2001;21:4712–4720.
30 Zine A, de Ribaupierre F: Notch/Notch ligands and Math1 expression patterns in the organ of Corti of wild-type and Hes1 and Hes5 mutant mice. Hear Res 2002;170:22–31.
31 Maiorana CR, Staecker H: Advances in inner ear gene therapy: exploring cochlear protection and regeneration. Curr Opin Otolaryngol Head Neck Surg 2005;13:308–312.
Baker · Brough · Staecker
32 Praetorius M, Baker K, Weich CM, Plinkert PK, Staecker H: Hearing preservation after inner ear gene therapy: the effect of vector and surgical approach. ORL J Otorhinolaryngol Relat Spec 2003; 65:211–214.
33 Staecker H, Praetorius M, Baker K, Brough DE: Vestibular hair cell regeneration and restoration of balance function induced by math1 gene transfer. Otol Neurotol 2007;28:223–231.
34 Staecker H, Li D, O’Malley BW Jr, Van De Water TR: Gene expression in the mammalian cochlea: a study of multiple vector systems. Acta Otolaryngol 2001;121:157–163.
Hinrich Staecker, MD, PhD
Department of Otolaryngology, University of Kansas School of Medicine, MS 3010 3901 Rainbow Blvd.
Kansas City, KS 66160 (USA)
Fax +1 913 588 6708, E-Mail hstaecker@kumc.edu
Vestibular System Gene Therapy |
63 |