
Учебники / Computer-Aided Otorhinolaryngology-Head and Neck Surgery Citardi 2002
.pdf422 |
Rosen and Simpson |
rately simulate the properties of skin and underlying tissue. He perhaps envisioned a time when modeling techniques and applications would ubiquitously assist physicians in planning and simulating their more complex procedures. Though many hurdles have yet to be overcome, we are closer today than ever before to realizing Limberg’s vision in soft tissue surgery. The development of complex modeling, data fusion, and virtual reality systems will empower today’s and tomorrow’s physicians.
In this chapter we will explore the present state of computer-aided surgery (CAS) applications in soft tissue surgery and project potential future developments. Section 23.2 will address the specific challenges of CAS in soft-tissue surgery. Section 23.3 will explore the benefits of CAS in this field and will detail state-of-the-art applications and techniques. Patient-specific models have been used in our institution, Dartmouth Hitchcock Medical Center, for a variety of applications. We have chosen three different etiologies and facial areas for examples in this chapter. We have used these patient-specific models to help plan surgeries for defects created by congenital anomalies, tumors, and trauma. In Section 23.4 we will present our vision for CAS in the future by detailing key forthcoming technologies.
23.2SURGICAL CHALLENGES AND CAS SOLUTIONS
Shortly after the introduction of the computer, physicians recognized the applications of modeling human tissues. Up until the past decade, while computational power may have been adequate for complex modeling, the mathematical models describing the motion and properties of human tissue had not yet been developed. Additionally, the equations defining skin and soft tissue were too large for all but the most powerful of machines. Recently, both of these impediments are dwindling, and today we are closer than ever before to creating simulations that accurately behave like human skin, muscle, and bone. The field of surgical simulation is a vast one with applications in medical training, preoperative planning, telesurgery, and many other areas. The ultimate progression of surgical simulation technology will incorporate enhanced visualization and proprioception of a completely immersed virtual reality display. Wearing a pair of electronic gloves and a specialized helmet called a head-mounted display (HMD), the surgeon of tomorrow will be able to see and feel the reconstructed nose described above. He will decide among multiple options by performing the reconstruction in a virtual operating environment and studying the results of tissue change. Figure
23.1presents this technology as it would be applied to a nasal reconstruction. Improvements in decision making and simulation technologies in medicine
have moved together throughout the development of the most modern CAS techniques. The computer has long been used as an expert system to aid the physician
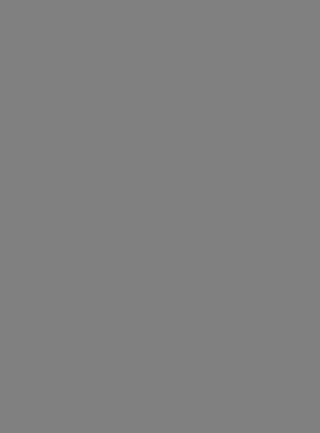
Computer-Aided Soft Tissue Surgery |
423 |
FIGURE 23.1 The history of planning for nasal reconstruction begins with a leaf template, progresses through clay modeling and computer-based simulation, and ultimately ends with virtual reality. (From Ref. 32.)
in decision making. An expert system is an algorithm or flowchart that summarizes an expert’s approach to a certain problem. The computer then takes the form of an interactive textbook by providing multiple approaches to specific problems. The computer will present predetermined case histories, and the outcomes to these cases can be compared. The user is able to select from a menu of possible actions and images. Based on the entered information the system will present a prediction of the outcome on the chosen procedure. Importantly, the expert system can be almost infinite in its knowledge databases through the use of networking technologies. Outcomes of all cases with the same initial conditions can
424 |
Rosen and Simpson |
be stored in its databases. The rhinoplasty simulator developed by Constantian et al. is an elegant example of an expert system [2]. Movement of tissue in this program affects a down-line of responses on associated tissues. The system presents known case histories and images that apply and suggest a number of possible outcomes. From this information, the surgeon is more equipped to make the best decision for the patient.
Expert systems are currently used for a variety of different applications, including rhinoplasties, mid-face advancements, and mandibular osteotomies, among others [3]. The computer herein displays two-dimensional (2D) images that have been digitally retouched or painted by the surgeon or operator. This graphical system has no incorporated data on the physical properties of the tissue. Such systems rely on the ability of the surgeon to predict the outcome of his planned procedure through photo retouching. One can recognize the insufficiencies of systems that fail to incorporate models of tissue change.
A major accomplishment in the field of CAS was the development of reformatted CT/MR data. By this method, CT/MR imaging scans are digitized and reformatted by a computer using graphic rendering techniques to construct threedimensional (3D) models. What is significant here is the fact that the models are patient-specific; the total model incorporates combined data. First-generation models of this sort were rigid and limited. Though good for procedures like bone reconstruction, these models lacked wide utility [2]. What soft tissue modeling needed was the ability to position tissue in one area and observe the response from another area. To accomplish this goal, the computer requires a model of the physical properties of tissues involved. To analyze relative mechanical responses to certain surgical procedures, the patient model must be designed to accurately account for patient tissue at each location of the body.
Current medical imaging technologies, including computed tomography (CT), magnetic resonance imaging (MRI), and positron emission tomography (PET), are all encoded volumetrically such that the spatial reference frame of the scan is independent of the patient. However, these imaging technologies fail to incorporate information as to how each element of tissue connects and interacts with other elements of tissue; herein lies the shortcoming of these scans. A partial solution to this problem was developed by Pieper and Chen, who designed a simulation system for soft tissue plastic surgery [4–10]. Recognizing the need of the surgeon to approximate responses to change and also properties of skin, muscle, and bone, they created CAPS, a software package for computer-aided plastic surgery. This system is based upon the formation of a finite element mesh (FEM) in which each segment of the face is divided into regions called elements. These complex geometric elements are linked such that their movements together approximate that of the original tissue. Each element is defined by the boundaries that it shares with other elements representing tissues.
Computer-Aided Soft Tissue Surgery |
425 |
CAPS uses a 3D model of the human face with FEM overlaid soft tissue to estimate the results of tissue ablation and rearrangement. First, a video scan of the face is performed that records skin color and cylindrical coordinates. The program converts the cylindrical coordinates to rectangular coordinates, which allows the surgeon to manipulate the image from any external point. A FEM is then constructed and the surface is color mapped accordingly. A skin stiffness matrix is referenced to integrate the strain and distortion this reconstruction produces on the skin soft tissue. An algorithm for the displacement data then constructs the CAPS predicted outcome. The surgeon views the 3D model on a computer screen and is able to cut, excise, create flaps, and rearrange tissues as the procedure requires. The computer incorporates the changes to the model and using the FEM shows the results on the tissue overall. The surgeon is able to visualize how excision of the lesion affects all areas of the face. The user designates simple approximation or a double Z-plasty rhomboid-to-W closure [5–11].
CAPS and other systems like it have great utility in facial plastic and reconstructive surgery. In addition to planning and simulating multiple types of tissue rearrangements, several other goals are achieved. Notably, the surgeon can choose among several types of flaps and incision plans to least affect the patient’s appearance and expressions. The patient is able to see how the surgery may change his appearance, which may reduce miscommunication and patient anxiety. Future CAPS systems may incorporate algorithms for tissue aging to yield more true results several years postoperatively. Though these algorithms have a long way to go before adequacy, CAPS is one of the best examples of soft-tissue patient-specific modeling systems available. Eventually, we envision this type of system to be used preoperatively and postoperatively for surgical planning and analysis.
Several state-of-the-art augmented reality (AR) systems allow for the joining of real and virtual data in the operating room. In AR, virtual patient information is fused with real patient data via a process called ‘‘data fusion.’’ There are numerous potential applications of AR systems in otolaryngology. In the clinical setting (such as the operating room), the surgeon employing AR must wear special glasses or a HMD so that the CT and MRI data appear superimposed on the area of interest. This type of system has been used to visualize a fetus in a woman’s abdomen prior to operation. Novice surgeons may have difficulty visualizing organs in 3D, and this technology adds dimensionality and a frame of reference to different types of visualization [12].
A plastic surgeon may choose to superimpose a nasal reconstruction procedure plan directly onto the patient’s skin. The computer software incorporates general principles of tissue change, including elasticity of different tissues, and then it can project how movement of a particular tissue area on the face will affect other tissue areas. In this way, the computer may offer guidance to the
426 |
Rosen and Simpson |
physician in his surgical plan. The ability of the computer to compute mathematical relationships of tissue movement will enhance the surgeon’s abilities. An AR system for neurosurgeons allows for CT or MRI imaging of a brain tumor to be transformed into a 3D image and superimposed on the patient’s head to help plan the skin incision and bone flap approach. The surgeon may then use the program in the operating room as a reference map to help assess the surgical margins.
In 1993, Performance Machines, an integrated approach for the AR in the operating room, was introduced. The Performance Machines system superimposed the patient-specific imaging data on the living patient in real time in the operating room. The registration of patient and dataset were done through an optical tracking system. When using this device, the surgeon must wear a HMD virtual reality helmet, or a display may be mounted upon a boom within the view of the surgeon. A combination of real patient images, mathematical models, and patient-specific imaging data, as well as procedure-specific cues and flags, would be projected within the surgeon’s perspective. During a tumor resection, Performance Machines gives the surgeon the ability to see through a tumor. This would allow the surgeon during a tumor resection to look through the tumor to see critical structures behind the tumor, such as a carotid artery, optic nerve, etc., and to avoid them. The patient-specific model is dynamic so that if the soft tissue moved, then the tumor within the soft tissue can be readjusted to predict its new position. Functional outcomes, including the motion of the jaw, may be predicted during the actual procedure.
Several medical robotic systems that are currently available are likely to gain additional applications in many new areas. Robotics are useful for calibration and the elimination of tremor. Surgical robotics also may perform tasks that are beyond the capabilities of human hands. Plastic and reconstructive surgeons will use robotic technologies to enhance and broaden their skills in numerous surgical procedures. ORTHODOC is a hip replacement surgery robotic system that helps the surgeon plan the operation in three dimensions [13]. The computer system takes a special CT of the hip after implanting three bone screws as reference points. ORTHODOC then gives instructions to a robot that forms the cavity using the screws for guidance. This system drills consistently with more precision that any human hand can. The surgeon may stop the robot at any point and revert to customary procedure as the operative field is in full view. Traditionally, the size and type of implants for hip replacement have been determined by overlaying templates on x-ray scans [14]. ORTHODOC and robotic systems like it reduce the margin of error in determining orthopedic implant sizing and types. The concept behind ORTHODOC may be applied to other surgical challenges. The reconstructive surgeon is frequently confronted by cases involving complex traumatic fractures of the craniomaxillofacial skeleton. For surgical reduction of these fractures, the surgeon must not only determine how to reconstruct a particular bone structure, but also choose among available fragments and donor sites. Robotic
Computer-Aided Soft Tissue Surgery |
427 |
systems will enhance the ability of plastic surgeons to reattach bone fragments, and in more delicate procedures robots themselves may perform procedures semiautonomously or even autonomously. Undeniably, robots will enhance the abilities of surgeons by performing actions outside the abilities of human hands.
Otorhinolaryngology–head and neck surgery is unique in that it encompasses a wide range of medical fields, including trauma, complex reconstructive surgery, elective surgery, complex tissue rearrangement, and other fields. Soft tissue surgery is an area of medicine that is especially enhanced by the ability of the surgeon to plan his cases. Reconstructive plastic surgeons are often faced with highly complex cases. The remedies for these cases require the collaborative efforts of surgeons from multiple specialties for the determination of the best course of treatment for the patient. It is crucially important that surgeons have the capacity to plan for these cases. Ideally, surgical planning would include surgical simulation. The various CAS technologies described in this section represent typical examples of CAS-based solutions for critical surgical challenges.
The goal of this technology is the prediction of surgical outcomes. As mathematical models improve, they should be better able to predict the response of skin, soft tissue, muscle, and joints to various procedures. Virtual reality simulators can now predict the mechanical outcomes of some musculoskeletal procedures. Similar computer-based information systems for other procedures involving the head and neck region would also be desirable.
23.3CAS APPLICATIONS
At Dartmouth Hitchcock Medical Center, we have used patient-specific models for a variety of applications. We have chosen three unique cases, which have distinct etiologies for the facial deformity. In addition, each case focuses upon a specific facial area. We shall discuss how state-of-the-art modeling and simulation technologies in congenital anomalies, tumors, and trauma have helped us to plan for complex surgical cases. For each case, we will discuss how CAS enhanced traditional preoperative planning. These cases illustrate that CAS can help surgeons achieve specific goals in soft tissue surgery with more precision and confidence than previously possible.
23.3.1 Congenital Anomalies
In the case of congenital anomalies, we have used patient-specific modeling for microtia, cleft lip repair, and other more rare anomalies (such as craniosynostosis). In these cases, it was possible to predict the outcomes of surgery using complex mathematical models. The patient-specific models allow the surgeon to compare several different lip repairs in a given patient before the actual surgical procedure commences.
428 |
Rosen and Simpson |
Microtia, which is a particularly interesting congenital anomaly, demonstrates computer-based modeling technologies in a direct clinical application. The technique developed over the years has been to use rib cartilage to create a framework that is placed under the skin in the first stage. The framework and skin are then elevated at the second stage. The lobule and concha reconstruction are either done at additional stages or combined with the first two.
Traditionally, a cast of the opposite ear has served as a planning guide for the reconstruction. Unfortunately, this technique is often inadequate for the reconstruction of a mirror image of the normal ear. Recently, a computer-based system has been used to scan the normal ear; this data may then serve to create a model of the intact, normal ear. Then the computer software may segment the cartilage independently from the skin in this model and subsequently create a true mirror image. This gives us a far more exact model of the ear we wish to create. The data set comprising the 3D model may guide the manufacture of a physical model of the missing ear and a physical model of the cartilage framework of that ear (Figure 23.2). In the past, surgeons would have carved a balsa wood model of the missing framework and then used this wood model to guide the construction of a rib cartilage framework. Obviously, these models— whether carved balsa wood or machine-milled material—serve the same purpose, that is, to approximate the creation of the cartilaginous framework from rib cartilage. Of course, the machine-milled models are more precise than other alternatives.
23.3.2 Tumors
Computer-aided soft tissue surgery using patient-specific models also has applications in the reconstruction of defects that result from the surgical excision of benign and malignant neoplasms as well as trauma. In this case, the CAS-derived approach was used to form the reconstruction of bone and soft tissue defects caused by the removal of a recurrent dermatofibrosarcoma protuberans.
In this case the tumor involved the left side of the forehead, the eyebrow, and the glabella area. The model helped to predict the size of the defect after the resection of the tumor, and during the subsequent reconstruction it was possible to compare the tumor side with contralateral, normal side (Figure 23.3). Since this was a recurrent lesion, the ipsilateral side was distorted by both the recurrent neoplasm and the first surgical excision. The contralateral side formed a template for the reconstruction. The computer simply created a mirror image of the unaffected side.
With the aid of the patient-specific anatomically mapped model, one may predict the size of the defect and plan the best donor site for a free tissue transfer. Often these procedures must be staged. In this example, the initial reconstruction

Computer-Aided Soft Tissue Surgery |
429 |
(A)
(B)
FIGURE 23.2 Computer-based imaging technology may be used to reconstruct an external auricle for the correction of microtia. (A) A patient-specific 3D model of the normal ear is shown. (B) The mirror image of the normal ear has been created by the computer from the imaging data from the normal side. (Copyright 2000 Medical Media Systems, Inc. with permission.)

430 |
Rosen and Simpson |
(A)
(B)
FIGURE 23.3 Preoperative modeling of tumor boundaries as well as adjacent tissues may guide intraoperative tumor resection and reconstruction. (A) The skull model depicts the underlying bony anatomy. (B) The imaging data was reformatted by the computer to present the tumor, resection margins, and the patient’s overlying soft tissue. (Copyright 2000 Medical Media Systems, Inc. with permission.)
Computer-Aided Soft Tissue Surgery |
431 |
used a lateral arm flap; later an eyebrow reconstruction, which involved tissue transfer from the contralateral brow was performed.
23.3.3 Trauma
The deformities that result from head and neck trauma are among the most challenging. In this example, a patient sustained a facial gunshot wound, which caused extensive destruction of the tissues of the left cheek, skin, muscle, body of mandible, and mucosal lining, as well as secondary ankylosis of the ipsilateral temporo-mandibular. A 3D model was created at the CAS workstation for surgical planning (Figure 23.4).
The reconstruction of this type of defect requires a composite flap encompassing bone, muscle, soft tissue bulk, and skin. A vascularized free tissue transfer of iliac crest bone with adjacent muscle and soft tissue can make possible the appropriate combination of tissue types for successful reconstruction. A computer-generated, patient-specific model can help the preoperative planning of this reconstruction.
This complex reconstruction may be termed a multiplex flap, since multiple tissue types share a single blood supply through a single vascular connection (see Ref. 15). In this case, the multiplex flap provides intraoral lining, bony reconstruction, soft tissue bulk, and facial motor function. The flap also serves to release the temporomandibular ankylosis. The bony part of the reconstruction can even support the placement of osteo-integrated dental appliances, whose introduction requires a second delayed procedure. From a soft-tissue perspective, the flap’s soft tissue must replace the entire ipsilateral cheek cosmetic unit. A computergenerated mirror image based upon the contralateral side can provide information about what is missing on the traumatized side.
23.4FUTURE DEVELOPMENTS
The changes in medicine afforded by computer technologies over the next decade will be nothing short of revolutionary. Herein, we will present a vision for the future of CAS. The applications of virtual reality and its associated components in medicine are numerous and not specific to head and neck surgery. We will describe how these technologies will enhance the surgeon’s ability with respect to the cases previously discussed. Presently, computational power is great enough to handle the tasks of accurately modeling change in human skin, muscle, and bone. Virtual reality (VR) encompasses a number of surgical research topics, including computer graphics, imaging, visualization, simulation, datafusion, and telemedicine. All of these areas are essential for our proposed vision.
Imagine a patient who requires a complex operation for the removal of a cancerous mass in the left cheek. The surgeon faces more than several critical