
Учебники / Computer-Aided Otorhinolaryngology-Head and Neck Surgery Citardi 2002
.pdf402 |
Kane et al. |
no doubt that bone may be easily segmented from CT data through simple thresholding operation. Nevertheless, soft tissue can also be modeled using these techniques, and independently segmented soft tissue (e.g., tumor) and bony objects can be created and fit together into a unified model (Figure 22.1). Base data collected from multiple modalities (e.g., CT and MRI) can also be fused, allowing the construction of more complex models. ‘‘Mirroring’’ can also be used to reproduce a reversed symmetric model, which is sometimes useful in cases where a unilateral defect is being reconstructed to match the contralateral undamaged appearance.
The procedure for the construction of these models varies and can follow one of several paradigms. Often the companies that produce these models sell them to the surgeon. In this approach, the surgeon specifies the characteristics of the desired model and then sends the base scan data to the company. The data may be sent electronically. Alternatively, digital storage media (such as optical disks that store the CT data) may be shipped to the manufacturer (either electronically or by physical media transfer). The model manufacturer does all segmentation and data manipulation. It is important for the surgeon to understand that the anatomical fidelity of the final model is sensitively dependent upon the expertise of the individual performing the data manipulation and segmentation. In the situation where the manufacturer performs a complex segmentation at a site distant from the surgeon, it behooves the surgeon who ordered the model to confirm that the segmentation was done in a way that matches the surgeon’s understanding of the underlying anatomy and pathology and that the segmentation is consistent with the goals for the model’s use.
It also may be possible for the surgeon to perform the segmentation and then export the segmented data for model creation. Nonetheless, many companies still prefer to do the segmentation, since the data manipulation may be cumbersome and dependent upon proprietary algorithms and data formats. Objects segmented from a volumetric data set must be converted into the expected input format of the prototyping machine. The specific steps of this process vary and may make it inefficient or impossible for the surgeon to perform the segmentation.
Some companies sell the rapid prototyping equipment directly to the medical institution. In this case the surgeon has greater control of the segmentation and model production. However, the costs of such systems are still beyond the means of craniofacial surgeons in most settings.
Regardless of the location of model production, the per model cost of production of these models currently is almost prohibitively high. However, costs may decrease as other manufacturers enter the marketplace and produce competition for price and services. When used in the clinical setting, medical insurers usually will not pay the full price of prototype production, but sometimes third-

Computer-Aided Craniofacial Surgery |
403 |
FIGURE 22.1 Rapid prototyping technologies for creation of 3D solid models. (a) Stereolithographic model of a skull with a shaded tumor (Courtesy ProtoMED CT Bone Models). (b) Fused deposition model (Courtesy of Stratasys, Inc.).
party payers will partially subsidize the cost of model manufacture. Unfortunately, economic considerations have probably significantly inhibited the clinical use of such models.
Several different rapid prototyping technologies are capable of producing high-quality anatomical models:
404 |
Kane et al. |
Stereolithography: Stereolithography builds the model through the sequential addition of multiple layers that are added by a computer-guided ultraviolet laser, which polymerizes a photosensitive resin made of acrylic or epoxy [6]. New layers are formed at the liquid/surface interface of the resin bath. After a layer is completed and hardened by the laser at the surface, the model is then submerged in the bath of liquid resin on a precisely controlled movable platform. The process then repeats, and the next layer is then solidified at the surface. The resin is translucent when dry. The process may be time-consuming, taking a full day to produce a model. Postprocessing is also required to remove the supports that were placed to prevent sagging of overhanging structures. Stereolithography has been the most commonly used technology in the production of medical models. This type of model is most often custom-ordered from a private company by the surgeon.
Fused Deposition Modeling: Fusion deposition modeling generates physical models by depositing extruded molten plastic in layers, which then solidify as they cool. Equipment for the production of these models can fit on a desktop and may be sold to institutions that may control the production of models locally. The costs of such equipment could only be justified if the institution requires a sufficiently high volume of models. This technique also produces supports, which are generated by the machine to prevent sagging of overhanging structures during the modelbuilding process and need to be removed prior to model completion.
Selective Laser Sintering: Selective laser sintering uses a flat sheet of powder, which is heated to close to its melting point. A carbon dioxide laser beam scans over the powder and heats the grains so that they melt on the outside and stick together (sinter). The base plate then moves down slightly, and the next layer of powder is spread across the surface by a rotating roller. The object is supported as it is made by the tightly packed unsintered powder, so it does not need extra supporting structures. At the end of the build process, the entire cake of powder, sintered and nonsintered, is allowed to cool down and lifted out of the machine. Then the loose powder is shaken off and the sintered object is freed. The finished objects have a matte, powdery surface. These models can be made in wax, sand or steel powder as well as nylon.
22.3.2Assessment of Anatomical Deformities and Planning of Surgical Interventions
Surgical simulation necessitates a surgical plan. The appropriateness, accuracy, and safety of the surgical plan depend on comprehension of the relevant anatomy. Reformatted CT data, displayed both as two-dimensional slice and three-dimen-
Computer-Aided Craniofacial Surgery |
405 |
sional volume-rendered images, provide the simulator with the information necessary for comprehension of that anatomy.
Segmentation of bone is done by thresholding. Simultaneous display of specific soft tissues, such as ocular globes, is accomplished by editing the soft tissue on two-dimensional slices or by using other segmentation techniques such as region growing. The edited soft tissue is then saved as an independent object or subvolume that can be displayed with or without the associated osseous structures. Following segmentation, which is the most time-consuming task in the surgical planning process, the resulting composite volume data set can be freely manipulated in six degrees of freedom and their surfaces deformed and reintegrated into a new whole. Of particular concern are quantitative and qualitative definitions of the specific anatomical relationships. As a result, the surgeon, during surgical planning, should focus on the brain and the orbits, the nasal cavity, the orbits and the maxilla (including the maxillary dentition and the infraorbital nerve foramina), the maxilla and the mandible.
While a variety of software packages are capable of performing at least a portion of the imaging tasks requisite for this type of surgical simulation, we have heavily relied upon the ANALYZE software package (Mayo Biomedical Imaging Resource, Rochester, MN) for these tasks. The software’s modules support all image processing in a flexible, but cohesive application environment. Furthermore, this software now runs well on a standard personal computer using the Windows operating system (Microsoft Corporation, Bellevue, WA).
Once the anomalous anatomy has been defined, a surgical plan can be synthesized. Initially, the plan is based on the dysmorphology, which will be surgically altered; then the plan is individualized for the patient specific pathology, the age of the patient, and the extent of correction desired by the patient and/or responsible adult(s). Generation of a surgical plan implies definition of a desired end result. If the patient truly has a unilateral deformity (such as a localized osseous or soft tissue hyperor hypoplasia), the unaffected contralateral side can be used as a template for the reconstruction of the affected side. Usually, the side that is contralateral to the major pathology has secondary deformation owing to skewed growth. Unicoronal synostosis (discussed later in this chapter) exemplifies this problem. Often, the actual pathology is bilateral.
Traditional craniofacial reconstructive surgical planning has been based upon data from a variety of sources: standardized life-sized lateral and AP skull x-rays (cephalometry), direct surface measurements (anthropometry), and replicas of the dentition (dental study models or casts). Such planning began with orthognathic surgery, where direct assessment of the occlusion (dental models) and indirect assessment of the jaws (cephalometry) usually yielded a surgically reliable plan. Reconstruction for grossly asymmetric jaw deformities and for upper face and calvarial deformities independent of the dentition was much more difficult to plan from conventional 2D radiographic images and dental models.
406 |
Kane et al. |
Nonetheless, an extensive and published normative database has been derived from cephalometry and dental study models. Although the cephalometric data are two-dimensional, they have been adapted for three-dimensional use [7,8]. The efficacy of such adaptation remains debatable. Data for select skull measurements have been derived from two-dimensional axial CT scan images without digital registration to anatomical fiducial points of individual subjects. Such data would be more reliable if they had been obtained within the three-dimensional CT digital data itself [9].
Regarding the orbital region, anthropometric data are available for standard surface measurements of the upper face [10], including the ocular globes [11], and conventional radiographic data are available for the interorbital space [12]. The discordant change in both intraorbital (the globe, extraocular muscles, and periorbital fat) and periorbital (eyelids and medial and lateral canthi) soft tissues compared with change in subjacent bone compromises the utility of such data since accurate soft-tissue outcome predictions in the orbital region are not currently possible. In short, true three-dimensional normative craniofacial data that are age, gender, and race specific are not available at this time. We have acquired and use a small set of normative data from infants and children with normal craniofacial structures who required CT scan for evaluation of neurological or traumatic disorders. A similar approach has been presented by the Australian group [13].
Three principles guide the planning of craniofacial interventions:
Calvaria. The calvaria are operated upon to release premature osseous fusion (craniosynostosis), to release intracranial constraint, to normalize dysmorphic shape, and to repair osseous defects. The extent of the calvarectomy required for both surgical safety (i.e., surgical exposure) and reconstruction varies depending upon the specific pathology. Nonetheless, attention is primarily focused on normalization of the frontal region; frontal deformation directly affects appearance because the region is not hidden by hair. Reconstruction of the superior orbital rim or rims is often a component of frontal reconstruction.
Globe-Orbit Relationship. Orbital region dysmorphology may consist of malrelationships between the orbits in the axial (horizontal), coronal (cephalad-caudad), or sagittal (anterior-posterior) planes; malrelationships between the ocular globe and the orbit with proptosis/exorbitism (too much ventral projection of the globe) or enophthalmos (posterior displacement of the globe); deformation of the orbit; abnormal size of the globe; or combinations thereof. Correction of such dysmorphology requires an anatomically exact understanding of the relationship between the ocular globe and its orbit. That relationship is a composite of the size of the orbit, the shape of the orbit, the position of the orbit relative
Computer-Aided Craniofacial Surgery |
407 |
to the rest of the skull, the size of the globe, and the volume of the extraocular orbital contents. The size and shape of the orbit and its position relative to adjacent bones can be altered purposefully with surgery. Such alterations are part of the surgical plan. The volume of the extraocular orbital contents often is inadvertently altered during periorbital surgery; this alteration cannot be planned predictably.
Maxillary-Mandibular and Dental Occlusal Relationships. Orthognathic surgery may be indicated for improvement of a compromised upper airway, protection of the globes (through enlargement of the inferior orbits), improved mastication, and correction of speech distortions. Cephalometric data and dental study models are time-proven effective planning tools for jaw surgery in dentoskeletally mature patients with essentially symmetrical malocclusions. Such patients usually do not require the sophisticated three-dimensional surgical planning discussed in this article. Planning of correction of major jaw asymmetries in mature patients and upper or lower jaw operations in young patients, however, is facilitated with three-dimensional computer-aided planning.
22.3.3 Philosophy for Surgical Simulation
Ideally, craniofacial surgical simulation should begin with the desired appearance and function and end with the hard and soft tissue operative plan to achieve that result. This process can be conceptualized as the following steps:
1.Preoperative skin surface three-dimensional image of the patient with documentation of functional limitations
2.Simulated desired skin surface three-dimensional image
3.Simulation of functional correction
4.Simulated hard and soft tissue three-dimensional imaging of the head coincident with the desired skin surface image and function
5.Blueprint of hard and soft tissue changes necessary to convert the real preoperative configuration to that simulated in step 4
This ideal is yet to be realized.
Nonetheless, the technology needed for the implementation of this ideal sequence is beginning to emerge [14,15]. The process begins with generation of polygonal surface meshes representing both the osseous and facial skin surfaces. The osseous surface is segmented from CT data, while the facial surface is extracted from a laser scan of the face. These two meshes are then registered anatomically to each other. The elastic and mechanical properties of the skin are modeled by mathematically treating the soft tissue as behaving like springs. These springs represent several physical properties of skin, including turgor, and gravity. The spring model is used to estimate the soft tissue deformation that would
408 |
Kane et al. |
be caused by the specified bone repositioning (Figure 22.2). The output of this method [14] is a photorealistic three-dimensional representation of the external appearance of the patient. This representation allows dynamic views of the face (e.g., prediction of soft tissue deformation with jaw motion).
The actual process of surgical simulation we routinely use today for complex craniofacial operations, whether done conventionally or with computer assistance, consists of the acquisition preoperative osseous images (plus dental models if relevant) and then planning/simulation for osseous normalization [16]. It is hoped that normalization of the bony skeleton will produce normalization of overall appearance and function; that is, the soft tissues will assume the desired position/appearance after correction of the targeted bony abnormalities/anomalies. Regretfully, this is not always the case. As we discuss skeletally based surgical planning and simulation subsequently, it is important to remember that appearance and function are the goals of surgery rather than the achievement of specific skeletal measurements. It is hoped that increasing sophistication of com- puter-assisted surgery will facilitate the attainment of these goals.
22.3.4Techniques for Computer-Aided Surgical Planning
Having transferred the CT data to the graphics workstation in a suitable format, the simulator produces a series of three-dimensional osseous surface volume– rendered images of the skull in standard projections. The planned osteotomies and ostectomies are drawn on these images in anatomically correct locations. Each bone segment to be altered is defined as a separate object by ‘‘cutting’’ the proposed sites, as if using a saw. Bone segments, such as an orbit, can be moved in all six degrees of freedom: anteroposterior, right-left, cephalad-caudad, and the rotations about each of their axes. ‘‘Bending’’ of a bone segment, a common technique for contouring of the calvaria and supraorbital bandeau in infants, can be achieved by creating multiple objects out of the bone segment. These component segments are moved or warped or both to reshape their contour, according to the plan. Bone grafts are simulated by creation of de novo objects.
If the operation includes alteration of the globe-orbit relationship, the globes and optic nerves are visualized as independent objects and then embedded into the three-dimensional osseous surface images. When globe position is expected to remain constant with respect to the majority of the skull (as in the correction of the orbital deformity of unicoronal synostosis), the mobilized bone segments are moved until the desired globe-orbit relationship is achieved. If the purpose of the operation is to reposition the globe(s) (as in hypertelorism correction), either a new composite object consisting of the globe and its orbit is created to be moved as a single unit during the simulation or the globe and its orbit can be moved independently to achieve a best-fit appearance.
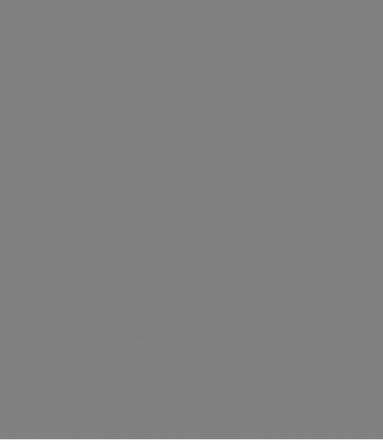
Computer-Aided Craniofacial Surgery |
409 |
FIGURE 22.2 Simulation of soft tissue deformation in craniofacial surgery. (a) Preoperative appearance. (b) Simulated postoperative appearance. (c) Postoperative appearance.
(d) Preoperative bone structure. (e) Simulated postoperative bone structure. (f, g) Soft tissue and bony appearance before simulated jaw motion (h, i) Soft tissue and bony appearance with simulated jaw motion. (Courtesy of Matthias Teschner, Telecommunications Laboratory, University of Erlangen-Nuremberg, Erlangen, Germany.)
The completed simulation is examined for correspondence with the desired outcome. If the coincidence is not satisfactory, the plan is altered, the simulation repeated, and the result rechecked. This process is reiterated until a satisfactory simulation is achieved. At that time, the movement of each defined object is recorded in voxels of the translation in the x,y,z coordinates and as degree of

410 |
Kane et al. |
rotation around the x-, y-, and z-axes, relative to the object’s original position. Voxels are then converted into metric measurements for intraoperative use. The spatial relationship between a displaced bone segment and unaltered reference points also can be obtained. Such reference surgical landmarks help determine the designated position while relocating a bony segment during the operation. A number of experimental and commercial intraoperative navigation systems have been used to make relocation of osseous segments more exact (see below). Nonetheless, intraoperative execution of the simulated ‘‘blueprint’’ remains a surgical challenge. Dental occlusion serves as the guide for maxilla-mandible movement, as was the case before computer-assisted surgery.
22.3.5Computer-Aided Surgical Simulation Case Reports
22.3.5.1 Case 1: Nonsyndromic Bicoronal Syntosis
A 2-month-old female baby was evaluated for brachycephaly without acral anomalies. Her family history did not include craniofacial deformities. Preoperative three-dimensional CT reformations documented bilaterally fused coronal sutures, a broad flat forehead, nasion recession with an obtuse nasofrontal angle, recessed supraorbital rimes, vertical elongation of the orbits, and bilateral superior proptosis. The surgical plan included bilateral coronal extended suturectomy, bilateral frontal bone recontouring, and supraorbital bar recontouring and advancement. The orbital aspect of the plan was quantified by assessing the position of the superior-inferior orbital rim tangent relative to the corneal plane and was found to be 10 mm dorsal to this plane (normal 2 mm).
Surgical simulation (Figure 22.3) began with bifrontal osteotomy including
FIGURE 22.3 This case summary illustrates simulation and verification of correction of the fronto-orbital deformities of nonsyndromic bicoronal synostosis in a 2-month-old girl. (Top row) Preoperative reformations. Note frontal and supraorbital recession with proptosis. (Second row) Surgical simulation. The frontal bones (blue) and the supraorbital bandeau (yellow) have been advanced and recontoured as independent objects based upon desired frontonasal contour and superior orbital rim-globe relation (see CD-ROM for color image). (Third row) Actual perioperative result (CT scan 4 days postoperatively). These images differ from the simulation in frontonasal contour and the presence of calvarial grafts at the pterions. (Bottom row) Three-dimensional longitudinal orbital projection to show the orbit-globe relationship in preoperative scan (left) for planning the supraorbital bandeau movement, simulated image (middle) demonstrating normalization of the relationship, and the actual perioperative result (right). Note the normalization of the superiorinferior orbital rim tangent to corneal plane distance: a change of 10 mm in both the simulation and the actual operation.
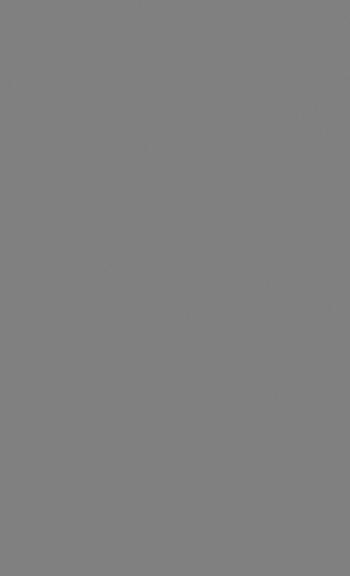
Computer-Aided Craniofacial Surgery |
411 |