
Учебники / Computer-Aided Otorhinolaryngology-Head and Neck Surgery Citardi 2002
.pdfHead and Neck Virtual Endoscopy |
341 |
was very labor intensive, it demonstrated the potential not only to visualize the surface anatomy of the lumen, but also to also view the submucosal structures, evaluate tumor extent, and visually display tumor interaction with surrounding structures.
19.3.3.1 Anatomical Delineation
We recently reported our experience using VE for evaluation of head and neck neoplasms in a series of 21 patients with head and neck tumors who underwent VE as part of their diagnostic evaluation [59]. VE flight paths were constructed from spiral CT images obtained during tumor staging workup, and images were compared to findings on axial CT images, flexible, and rigid endoscopic findings. VE images were constructed to provide luminal contour detail only. Overall, normal anatomy was clearly seen at all locations in the majority of cases. There was, however, variability in the quality of images at different sites. VE was able to display tracheal and subglottic anatomy not well visualized by standard mirror or flexible fiberoptic examination. The semirigid structure of the trachea allowed for excellent visualization of the tracheal rings, the cricoid cartilage contour, and the mucosal contour of the undersurface of the true vocal cords. Figure 19.4 displays superior (Figure 19.4A) and subglottic (Figure 19.4B) views of the true vocal folds; the subglottic view clearly provides a unique visualization of the undersurface of the true vocal cords. Nasopharyngeal anatomy was also clearly delineated with VE. Fine anatomical details such as the fossa of Rosenmu¨ller, torus tubarius, the posterior choanae, the posterior aspects of the inferior turbinates, and the nasopharyngeal walls were clearly delineated in the majority of the patients (Figure 19.5). Both the trachea and nasopharynx have in common a relatively rigid framework that is generally patent and does not tend to collapse in the normal state. Laryngeal anatomy was also generally well visualized by VE. The true vocal folds, false folds, arytenoids, aryepiglottic folds, and epiglottis were all well seen in the majority of cases. The laryngeal ventricles were not well seen in most cases in our study, most likely because of the selected flight path, field of view, and angulation of the virtual camera. It is likely that variation of the flight path would allow better delineation of this area. For example, Yumoto et al. produced sagittal cutaway views of the medial laryngeal surfaces, which displayed the ventricles clearly [72]. Image quality at the tongue base, vallecula, and pyriform sinuses was more variable. Several anatomical factors contributed to this variability. Posterior displacement of the tongue base, apposition of the tip of the epiglottis on the posterior pharyngeal wall, and collapse of the soft palate and/or lateral pharyngeal walls all contributed to obliteration of the lumen, rendering contour delineation imprecise and unreliable in many patients. Apposition of mucosal surfaces in many studies limited resolution of the normal anatomy. This resulted from inability to discern surface contour when two surfaces are touching each other. Figure 19.6 shows the apparent melding of apposed
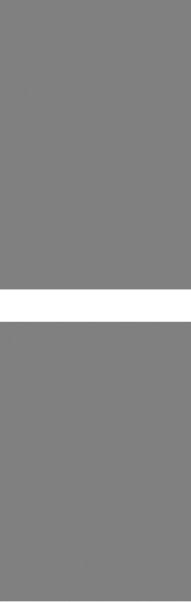
342 |
Armstrong and Nguyen |
(A)
(B)
FIGURE 19.4 Laryngeal anatomy: (A) superior view; (B) subglottic view of normal larynx.
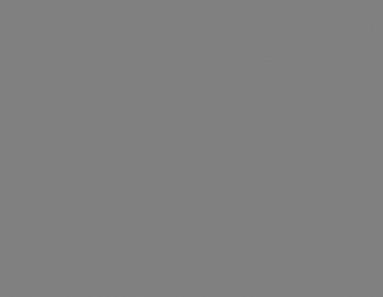
Head and Neck Virtual Endoscopy |
343 |
FIGURE 19.5 In this view of nasopharynx from below, note detailed depiction of fossa of Rosenmu¨ller, torus tubarius, vomer, and posterior choanae. Due to the rigid structure of the nasopharynx, high-quality images of the surface features can be reliably obtained.
tissues at the epiglottis (Figure 19.6A) and uvula (Figure 19.6B). With the exception of superior ability to visualize the subglottis from an inferior perspective, limitations of VE were similar to those obtained by standard visual examination techniques in the conscious patient. In the hypopharynx, distension of the pharyngeal walls is necessary to visualize the surface anatomy, either by performing a valsalva maneuver in the awake patient, which is a standard part of our flexible endoscopic examination routine, or manual distension of the tissues during operative endoscopic procedures under general anesthesia. Performance of a valsalva maneuver during image acquisition for VE may improve image quality, but it will likely require an additional sequence of scans, since breath holding will forcefully close the glottis, degrading the image quality of the true and false vocal folds.
19.3.3.2 Tumor Assessment
The ability to delineate tumor extent using VE also varied by anatomical location, and the same anatomical constraints that limited visualization of normal anatomy using VE also affected visualization of tumors. Subglottic tumor extension was

344 |
Armstrong and Nguyen |
(A)
(B)
FIGURE 19.6 Apposition of mucosal tissues in airway. Apposition of the epiglottis (A) and uvula (B) demonstrate how abutment of tissues results in a loss of distinction between the two independent surfaces at the interface and artifactual distortion of the anatomical appearance.
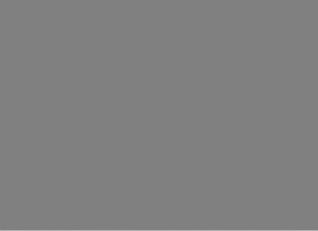
Head and Neck Virtual Endoscopy |
345 |
clearly identified as blunting or mass effect at the anterior commissure, and laryngeal tumors were generally well visualized, with the exception of small mucosal T-1 glottic tumors that could not be distinguished from normal mucosa and were missed by both axial CT and CT VE. Visualization of supraglottic tumors was also generally accurate. Figure 19.7 shows a VE image of a T-2 epiglottic SCCA viewed from above. Visualization of the laryngeal surface of the epiglottis, which can also be difficult with rigid direct laryngoscopy, was also suboptimal with VE in our study. The laryngeal surface of the epiglottis lies in a plane nearly parallel to the visual axis of standard endoscopic examinations, making visualization of the surface difficult. However, visualization may be improved with different viewing angles designed to specifically image the laryngeal surface of the epiglottis. Tumors of the hypopharynx and oropharynx were less well visualized, and pyriform sinus tumors were especially problematic, just as they are with other noninvasive diagnostic techniques that do not distend the pyriform sinus. Although in some patients the images were able to show the hypopharyngeal anatomy, tumor visualization and assessment proved difficult. Tumors of the posterior pharyngeal wall could be visualized, but if there was apposition of redundant mucosal folds, visualization was limited. In the hypopharynx, gravity, apposition of mucosal surfaces, and pooled secretions decrease resolution and account for the loss of contour definition. Mucosal contour changes were visualized by VE, but subtle mucosal irregularities could not be appreciated, limiting the sensitivity
FIGURE 19.7 T-2 squamous cell carcinoma of the superior portion of the laryngeal surface of the epiglottis. Tumor is shown extending along the superior margin and along the laryngeal surface of the epiglottis.
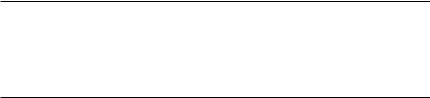
346 |
Armstrong and Nguyen |
TABLE 19.2 Factors Impairing VE Image Quality
Flat mucosal or submucosal lesions
Apposition of two mucosal surfaces (pyriform sinus, tongue base, epiglottis, soft palate)
Bulky tumors apposing multiple mucosal surfaces Pooling of secretions
Motion (swallowing, phonation, breathing, arterial pulsation)
of the technique, especially for smaller tumors. Factors leading to impaired visualization of normal anatomy and tumors are summarized in Table 19.2.
19.3.3.3 Evaluation of Airway Obstruction
Burke et al. published their experience using CT VE to evaluate 21 patients with airway obstruction resulting from a variety of causes including laryngotracheomalacia, tracheal masses, glottic webs, innominate artery compression, and vocal fold immobility [73]. They found that VE was as accurate as optical endoscopy for evaluation of fixed stenoses. Correct diagnosis was made in 95% of fixed obstructions, and VE accurately depicted the extent of the lesion seen by optical endoscopy in all but one case. VE was better able to assess the length of tracheal tumors than optical endoscopy. However, the technique was not accurate for evaluation of dynamic obstructions from tracheomalacia, vocal fold immobility, and innominate artery compression of the trachea. This initial study revealed areas for further study and improvement. Their group proposed evaluation of gated examinations timed for respiration and/or heartbeat and infusion of contrast to aid evaluation of dynamic obstructions.
VE of the upper airways shows significant promise for evaluation of the trachea and subglottic larynx and is useful for evaluation of fixed airway obstructions. In addition, the technique may provide information that can aid in staging of persons unfit for operative endoscopy under general anesthesia. Current techniques allow resolution that can image contour deformities, but no color information or indication of vascularity is provided, and the resolution is not precise enough to demonstrate subtle mucosal irregularities that are characteristic of smaller tumors. Nevertheless, this technology is in its infancy, and improvements in resolution and image processing may greatly improve the resolution capabilities of VE.
19.4ADVANTAGES AND LIMITATIONS OF VIRTUAL ENDOSCOPY
VE has a number of inherent advantages (Table 19.3). First and foremost, VE is a noninvasive technique and has been successfully applied to depict anatomy

Head and Neck Virtual Endoscopy |
347 |
TABLE 19.3 Advantages and Limitations of VE
Advantages
Noninvasive imaging technique
Ability to use CT, MRI, or ultrasound data
3D representation of complex anatomy with perspective
Ability to view lesions from any angle without lens angle distortion Visualization past tight stenoses
Visualization of luminal irregularities
Objective noninvasive documentation of response to therapy Limitations
Limited resolution of mucosal defects No color information
No texture information
No tissue obtained for diagnosis
Anatomical visualization variable by location Limited ability to evaluate tumor invasion Time requirement to construct flight path Radiation exposure (CT)
Cost
and pathological changes at a number of sites in both the head and neck region and the entire body. VE imaging provides a realistic depiction of complex radiographic anatomy in a manner that is more familiar to clinicians [16,20,74], and virtual reconstructions when seen side by side with corresponding axial images facilitates the clinician’s 3D interpretation and understanding of both normal anatomy and pathological changes. VE is a very flexible technique that is not subject to the same limitations as visual endoscopic techniques. There is no lensangle distortion, and the virtual camera can be maneuvered in any desired direction, magnification, and field of view. This allows visualization of anatomy from unique perspectives and the ability to bypass anatomical obstructions that cannot be traversed using standard optical endoscopy techniques.
Clinically, a number of areas in the head and neck region show great potential for utilization of VE. Assessment of airway obstruction is one area where VE has been demonstrated to be a helpful adjunctive study. VE is an excellent tool for evaluation of luminal incursions, making it useful for evaluation and follow-up for lesions of the subglottic larynx and trachea. It is especially helpful in situations where there is significant narrowing that precludes safe passage of an endoscope through the obstruction and for evaluation of patients medically unfit for general anesthesia. The airways have been studied much more extensively than other areas of the head and neck, but early studies in the sinuses and the temporal bone are encouraging and have stimulated further research to better define how and when VE can be applied at these sites.
348 |
Armstrong and Nguyen |
VE is a flexible and powerful technology that is widely applicable to a number of medical and nonmedical applications. Perspective image rendering requires high-resolution cross-sectional image data that is an accurate anatomical representative of the imaged structure. The ability to perform VE is independent of imaging modality, but the resolution, precision, and inherent contrast differences between tissues are important inherent properties of the imaging modality that determine the quality of the VE study. There is no limitation on the scale of applicability of these techniques. Perspective rendering techniques have applications in flight simulation, terrain mapping, and manufacturing on a large scale and microscopic applications on a very small scale. For example, 3D reconstruction techniques have been applied to image subcellular anatomy using data obtained from confocal microscopic examinations [65,75]. Along with the flexibility to adapt VE to different imaging modalities, VE image data is inherently portable and can be transferred to other computers for further study and manipulation. The portability of the data can facilitate additional applications of VE. The image data are also objective, and comparison of subsequent VE studies is easy to perform. Optical endoscopic examinations do not share this advantage unless the study is recorded onto videotape or digital media. The presence of a permanent record of the examination makes VE potentially very useful for posttherapy follow-up of clinical response or detection of recurrence or relapse following treatment. It may also be able to obviate the need for repeated surgical endoscopy in certain cases. Medical student and physician education and preoperative surgical planning are two logical applications suggested in other studies [54,74,76].
Although VE has several important advantages over operative endoscopy and can accurately delineate many lesions, there are a number of disadvantages and limitations to this imaging modality (Table 19.3). Perhaps most important is that, although VE provides excellent contour definition, fine mucosal irregularities, vascularity, and color are not depicted [63]. These characteristics are often critical for detection and delineation of the extent of tumors, thus subtle mucosal or submucosal lesions cannot be detected by VE. Contrast resolution is inherently limited by the type of data obtained. For CT, only data reflecting x-ray absorption are recognized—not the vibrant colorful appearance of the tissues seen visually. Although collimations as thin as 1 mm with pitch ratio of 1 can be attained with current spiral CT scanners, the resolution is still not refined enough to delineate subtle mucosal details visible to the human eye. A second major drawback of VE is lack of tissue for diagnosis, which is a critical requirement in the evaluation of potential malignant neoplasms. Successful imaging is dependent upon at least some lumen patency, and when there is collapse of soft tissues or abutment of tumor against the opposite mucosal wall, image quality decreases markedly, just as occurs with flexible endoscopic examinations.
The cost of constructing and interpreting VE images is a barrier to acceptance of this diagnostic modality. We found generation of VE sequences for our
Head and Neck Virtual Endoscopy |
349 |
initial examinations took more than one hour with supervision by a neuroradiologist, but with experience this time rapidly decreased to approximately 20 minutes by a skilled CT technologist. This finding is similar to that reported by Rodenwadt et al. [63]. VE also requires specialized hardware and software, which represent a significant capital expenditure. Efforts are underway by a number of groups to develop automated and semiautomated methods to speed up the creation of VE studies [60,70,77]. Automation of image segmentation and flight path generation are areas of focus to improve the speed to complete the examinations, and in the near future many of the functions currently performed by the physician or technologist at the imaging workstation may be automated.
19.5FUTURE CHALLENGES
A number of challenges need to be met before widespread incorporation of VE into clinical practice can occur. There has been extensive evaluation of VE throughout the body, and a number of preliminary studies in the head and neck region have been completed. The indications for VE and an understanding of the strengths and limitations of VE are rapidly being acquired, but additional study is necessary to further define the utility of current applications for VE as well as to discover new applications for this imaging tool. Areas where VE is likely to play a greater role in the future are clinical education and integration with other technologies. VE use will expand as technology improves, costs decrease, and the new applications of VE are discovered.
19.5.1Improvement of VE Study Quality and Speed
Several technical hurdles limit the utility of VE, and attention is focused on these problems. The quality of the raw image data will reflect the quality of the final VE study. CT, MRI, and ultrasound rely on different physical properties to display tissue contrast and have different contrast and spatial resolutions. A current limitation of VE studies is they do not have the resolution necessary to show the fine mucosal details that provide texture clues visible to the naked eye. Increased spatial resolution may improve this somewhat, but a significant enhancement of resolution will be necessary. MRI has the advantage of acquiring images without ionizing radiation, but the usefulness of MRI for generation of VE is limited by the increased time to acquire images and lower tissue resolution. MRI has better soft tissue contrast resolution than CT, which allows more precise segmentation of anatomical structures and potentially improved discrimination of soft tissues and tumor margins. Use of CT and MRI data together may provide better contrast and resolution of deep tissues than either study alone. Initial experience fusing CT and MRI data is promising, but significant refinement is required to allow
350 |
Armstrong and Nguyen |
seamless merging of image data [60]. For MRI to assume a more prominent role in VE, advances in MRI technology to allow more rapid imaging and processing will be necessary. There is considerable interest in fast scanning techniques such as fast spiral MRI, echo planar imaging, and fast low-angle shot MRI, which decrease scanning time to 50 msec or less [78,79]. Although fast scanning MRI techniques are currently receiving great attention in myocardial imaging and other applications where rapid acquisition is critical [78,79], the contrast and spatial resolution as well as signal to noise ratios currently available do not provide adequate image quality to produce usable VE studies at this time.
Data segmentation is a critical step before image rendering occurs, and also one of the most difficult. Segmentation is simply classification of the image data to allow discrimination of different features. CT attenuation and MRI signal intensity values are generally used in the segmentation process. Unfortunately, these values alone often do not allow precise discrimination of vital anatomical structures or tumor margins, and manual or semiautomated segmentation of anatomical structures is performed to specifically select desired anatomical features. It is a tedious task to manually outline individual anatomical structures, and current semiautomated segmentation programs rely on the skill of the operator to properly select the anatomic regions of interest. Automation and improved accuracy of the data segmentation process will greatly simplify generation of VE studies and decrease cost [23].
Along with automation of segmentation processes, automation of flight path determination and VE frame acquisition along the flight path will greatly speed the process and decrease study costs. Manual flight path generation and image acquisition has a learning curve that must be mastered before studies can be efficiently produced, but the process involves repetitive manual selection of position, view direction, view angle, and lighting. Algorithms to partially automate the process have been developed, but further improvements are necessary before they can be completely automated to dependably generate useful flight paths [68– 70]. By removing the repetitive steps in the imaging process and increasing automation, protocols could eventually be developed to allow the technicians currently performing CT and MRI scans to efficiently perform VE studies. This is a necessary prerequisite for VE to be cost-effective.
The next logical advancement of VE after development of automated data segmentation and flight path generation is the development of true virtual reality simulations that will radiographically support real-time navigation throughout the body (analogous to the concept of miniaturization and travel throughout the body depicted in the 1966 movie The Fantastic Voyage). It is not difficult to conceive that an operator will be able to access a computer workstation, set the camera angle, and manipulate a joystick to navigate throughout the imaged data set in real time. As described earlier, this will require advances in software as well as