
Учебники / Computer-Aided Otorhinolaryngology-Head and Neck Surgery Citardi 2002
.pdfHead and Neck Virtual Endoscopy |
331 |
using axial CT, MRI, and, recently, ultrasound data [23–25]. VE is widely applicable throughout the body, and a number of organ systems have been studied, including the esophagus [26,27], stomach [28,29], small bowel [30–32], colon [22,33–35], genitourinary tract [36–38], tracheobronchial tree [21,39], vascular structures [40–43], nervous system [44–48], the temporal bone [49–52], paranasal sinuses [23,53–58], and upper respiratory tract [59–63]. A summary of the anatomical regions under investigation is displayed in Table 19.1.
19.2VIRTUAL ENDOSCOPY TECHNIQUES
It is important to understand the essential distinction between 3D image reconstructions produced with software that does not incorporate the concept of perspective into the image reconstructions, and perspective-rendering methods used to produce VE studies. Perspective is an artistic term that describes techniques used to represent the spatial relationships of objects, as they would appear to the eye when viewed on a 2D surface. A simple illustration of perspective representation is shown in the two cubes in Figure 19.1. The cube on the left (Figure 19.1A) is drawn without incorporation of perspective to produce a representation of an object that appears three-dimensional, but relative sizes are not taken into account. The size of the posterior face of the cube is the same as the front face surface. The cube on the right (Figure 19.1B) depicts a decrease in the size of the apparent posterior surface of the cube, which seems to be viewed from a further distance than the front surface. This second illustration is more representative of what the human visual system would normally perceive. The 3D reconstruction techniques that do not incorporate perspective use an orthogonal or parallel ray-casting technique to display the 3D image onto a 2D plane; this approach assumes that the object is viewed from a remote distance in space [64,65]. When viewed from long distances, the light rays reflected from an object are nearly parallel, and use of parallel ray-casting techniques to display the image does not produce significant distortion (Figure 19.2A). Using parallel ray projections to produce 3D reconstructions is mathematically and computationally much simpler, but the view generated is not conducive to close-up viewing. Attempts to obtain a magnified view results in a larger view of a smaller portion of the structure. This situation is analogous to using the zoom feature on a copy machine. The image is larger and appears closer, but the field of view is extremely narrow because of limitations imposed by the reconstruction algorithm.
Perspective rendering techniques attempt to simulate the properties of the human eye by taking into account divergence of light rays between the eye and different portions of a given object, differences in the size of objects viewed from different distances, the field of view of the human eye or camera lens, and the effects of lighting, shading, and reflections. Figure 19.2 graphically illustrates differences between parallel ray reconstruction (Figure 19.2A) and perspective
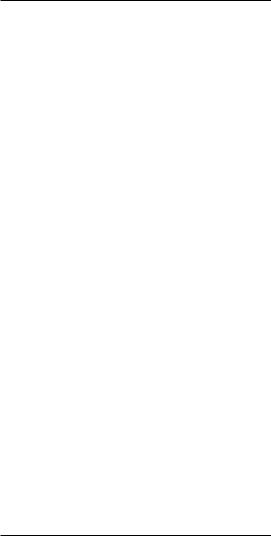
332 |
Armstrong and Nguyen |
TABLE 19.1 Virtual Endoscopy: Anatomical
Regions of Clinical Investigation
Otolaryngology Trachea Larynx Pharynx
Otological structures middle ear cochlea vestibular system
Nasal cavity and paranasal sinuses Pulmonology
Bronchoscopy
Vascular Surgery Carotid artery Aorta
Renal artery Gastroenterology Esophagus
Stomach Small bowel Biliary tract Colon
Urology
Renal calyces Ureter Bladder
Evaluation of bladder reconstructions Neurosurgery
Spinal canal Intracranial vasculature
Cerebral ventricular system Aneurysm mapping
Gynecology
Uterus Fallopian tubes
Orthopedic surgery Major joint spaces
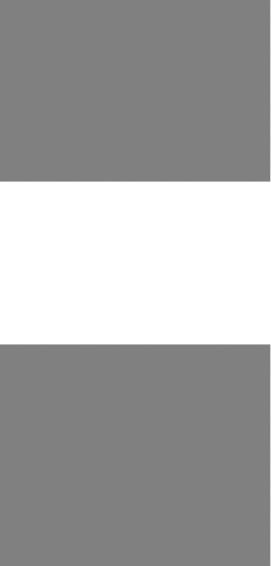
Head and Neck Virtual Endoscopy |
333 |
FIGURE 19.1 Depiction of cube without and with incorporation of perspective. (A) Cube is constructed to depict the appearance without incorporation of perspective. The front and back (shaded) surfaces are of equal size. (B) Cube incorporates the concept of perspective. The more distant posterior surface is depicted as smaller to account for the increased distance from the viewpoint.
FIGURE 19.2 Parallel ray vs. perspective rendering of 3D structures. (A) Parallel rays projected onto a planar surface to simulate nonperspective rendering. There is a linear transposition of data from the 3D cube onto the 2D plane. (B) Divergence of light rays from the eye or camera lens on the left to the surface of the cube. The relative position of sampled points on the object will vary depending on their relative position in space and distance from the viewpoint.
334 |
Armstrong and Nguyen |
rendering (Figure 19.2B). In parallel ray reconstruction, the parallel light rays pass through the object and map onto the planar surface with one-to-one correspondence, irrespective of distance from the viewpoint. In contrast, in perspective reconstruction, the relative position of the points mapped along the diverging rays projected from the viewpoint mapped onto a 2D plane varies by distance from the viewpoint, resulting in changes in the apparent size based on distance from the viewpoint. By mimicking the optical properties of the human visual system in a fashion similar to the ways in which an artist creates a painting with cues to relative size and distance, the perspective rendering software can create a realistic appearing simulation of an endoscopic visualization from cross-sectional data.
Four major steps are required to create a VE study. Cross-sectional data are first acquired by either spiral CT or MRI and transferred to a 3D workstation. These data are then processed to construct a 3D model of the imaged structure for the third step. Next, individual perspective rendered images are created. In this step, a series of rendered images along a selected pathway are generated and linked together in the final step to produce a simulated flight path. A number of technical variables at each of the above steps need to be understood, as they will affect the ultimate quality of the studies.
19.2.1 Data Acquisition
The majority of VE studies currently utilize CT data, and the majority of this discussion will concentrate on processing CT data. Advances in CT technology, especially the development of spiral (helical) CT scanners, has enabled rapid acquisition of high-resolution CT scans. Because spiral CT data are rapidly acquired in a continuous fashion, there is no interval gap or misalignment of successive slices from patient motion. Interval gap and image misalignment can be problematic in conventional CT scanners. Parameters affecting the quality of spiral CT studies include collimation width of the x-ray beam, table translation speed, image reconstruction interval, and the length of the anatomic region covered by the examination, which will be discussed briefly below.
The collimation width is a principal determinant of the resolution in the longitudinal axis. In general narrow collimation widths of 1–2 mm are used in examinations of the head and neck. Translation speed indicates how rapidly the body is moved through the scanner, while the gantry containing the x-ray tubes and detectors rotate at a fixed rate. Translation is generally expressed in terms of pitch factor, defined as:
Pitch factor [table speed (mm/sec) collimation (mm)]gantry rotation period (sec)
Head and Neck Virtual Endoscopy |
335 |
The distance to be covered by the study must also be taken into account with spiral CT studies. X-ray tube heating limits the available time to obtain a scan without interruption to allow x-ray tube cooling. When collimation width and pitch are small, a shorter distance is covered in a given scanning period. Ideally, a very narrow collimation width and small pitch would be selected to provide the highest possible resolution, but this limits the distance that can be scanned without interruption to allow x-ray tube cooling.
Since scanning is performed in a continuous fashion, the data are manipulated using interpolation algorithms to reconstruct a series of axial slices. The number and spacing between slices is specified by the reconstruction interval. Selection of an optimal reconstruction interval is important because it is also an important determinant of the longitudinal resolution of the final VE images [67]. Appropriate selection and balancing of collimation width, pitch, and reconstruction interval are all necessary to obtain a high-quality VE imaging study. Optimization of these variables has largely been a heuristic exercise, although several researchers have attempted to use phantoms to determine optimal scanning values [17,32,63]. In our practice, for VE evaluations of head and neck neoplasms or airway compromise, spiral axial CT imaging is typically performed from the skull base to the thoracic inlet using iodinated intravenous contrast with the patient breathing in a relaxed manner (breath holding physiologically distorts the glottic and supraglottic anatomy). We have found the following parameters to provide high-quality data for endoscopic renderings:
Collimation thickness 2 mm
Pitch factor 1.5–2.0
Reconstruction interval 1–2 mm
19.2.2 Data Processing for 3D Reconstruction
The axial image data are transferred to a dedicated computer workstation and processed using image rendering software. Prior to constructing VE images, a 3D model of the data is constructed to first generate a 3D data set that can be analyzed by the rendering software. The processing methods to generate the 3D model differ based on the software used, and several manufacturers have developed and made commercially available their own proprietary software (Voxel View /Vitrea , Vital Images Inc., Fairfield, IA; Voyager , Marconi Medical Systems, Cleveland, OH; 3D Navigator, GE Medical Systems, Milwaukee, WI). Two general methods are used to prepare image data for perspective rendering: shaded surface display (SSD) and the more advanced volume reconstruction techniques used in volume rendering software.
SSD techniques rely on identification and selection of surface feature data to construct a 3D model of the lumen surface. This is accomplished by a process
336 |
Armstrong and Nguyen |
of segmentation that requires selecting voxels of desired intensities for inclusion into the data set. A 3D surface model containing the voxels located on the surface of the imaged object is reconstructed, and extraneous data are eliminated. SSD has the advantage of requiring less computational power to process the image data, but the rendered images have increased artifacts such as floating pixels and surface discontinuities. Because data are eliminated, information about submucosal tissues is not available.
In contrast to SSD, volume rendering software systems assign a color and opacity to each voxel in the entire data set based on the intensity of the image data. For CT scans, intensity is the attenuation expressed in Hounsfield units, while for MRI scans, intensity is the signal intensity. If desired, manual or automated segmentation of the data can also be performed prior to image rendering to highlight desired structures for incorporation into the VE image (see Ref. 49). Volume rendering has the advantage of retaining all available data, which can be selected and ultimately utilized by altering opacity, color, and lighting effects in the rendering program. Although volume rendering requires considerably more computational power, no information is lost during the reconstructions, and this method of image processing is becoming more popular as computer hardware costs decrease.
19.2.3 Perspective Rendering
The next step in generation of a VE study is to visualize the digital 3D reconstruction in a manner similar to that of the human eye through the use of perspective rendering software. Although all objects imaged will be finally displayed on a 2D surface (either hard copy, films, or monitor screen), the perspective effect created by the graphics software gives the object the impression of three-dimen- sional depth of field.
The perspective rendering software programs provide a number of tools to emulate visualization of the imaged object. These tools mimic human visual perception of real objects. Software tools allow selection of viewpoint and view direction, view angle (which alters perceived magnification), and lighting effects (intensity and direction of light). Color and opacity can also be altered as described earlier. Skillful manipulation of these imaging tools allows the operator to produce single frame radiographic images that visualize the anatomical structures from almost any direction, distance, magnification or lighting angle. Figure 19.3 shows the first frame of a VE study, looking superiorly into the trachea. The subglottic larynx is visible in the distance.
19.2.4 Animation of VE Images
After generation of the first viewpoint is performed, the flight path for the study is selected either manually or semiautomatically using programmed algorithms

Head and Neck Virtual Endoscopy |
337 |
FIGURE 19.3 Virtual endoscopic still frame depicting the trachea and subglottic larynx with cut-away of the thoracic cavity. This image was constructed using perspective volume rendering technique, incorporating all image data as demonstrated in the cut-away view through the thorax. Although submucosal details are not visible through the lumen in this projection, alteration of opacity could be performed to visualize the relational anatomy of the great vessels, thyroid gland, and other structures adjacent to the tracheal lumen.
[68–70]. At selected points along the flight path, the operator acquires images using the selected viewing parameters. The flight path consists of a sequential collection of perspective rendered images incrementally acquired at specified points along the flight path. Flight paths can be viewed as an animated movie that simulates flying through the patient; this approach emulates the experience of an endoscopic examination. The operator can control playback rate and view the study in forward or reverse direction along the predetermined path. Each key frame of the movie is a representation of a viewpoint, view direction, and view angle, and the quality of the study is dependent on the appropriate selection of each of these image components along the entire flight path.
The flight paths generated by VE provide a simulated endoscopic examination. The current technology is not real-time and is not interactive. In order to provide a true virtual reality experience, it is necessary to produce real-time image rendering and display at 30 frames per second (standard frame rate for viewing video) and update the images interactively in response to operator changes in position and view direction within 100 msec (preferably within 10 msec) to provide the sense of immediate response to operator instructions. Current computational power on commercially available workstations does not come close to
338 |
Armstrong and Nguyen |
realizing these requirements, but given the rapid pace of increased computational power, this will be possible in the foreseeable future.
19.3CLINICAL APPLICATIONS OF VIRTUAL ENDOSCOPY
Since the first clinical reports of VE in the colon and bronchi [21,22,39], VE techniques have been developed for a wide variety of organ systems, including the upper and lower digestive tracts, the biliary tree, the urogenital system, the central nervous system, vascular structures, and major joint spaces (Table 19.1). Although VE has been applied to a number of areas of the body, the techniques are still relatively primitive, and clinical indications for use are still being assessed, while VE techniques and methodology are undergoing rapid evolution. By no means has the full potential of VE been explored. Evaluation of VE in every major organ system and the continued rapid pace of innovation and evolution of the technology indicate that VE will rapidly evolve and will advance beyond the realm of research and become a standard tool in clinical practice. Just how, when, and where VE will play its role remains to be defined.
In the head and neck region pilot studies have been completed at a number of sites. These early studies, which will be described below, have demonstrated the ability of VE to visualize anatomical structures and pathological lesions in a manner similar to that of optical endoscopic examinations. These early studies have provided valuable information necessary to further refine and define the applicability of VE in clinical diagnosis, surgical planning and treatment, and integration with other technologies and teaching applications.
19.3.1 Temporal Bone and Middle Ear
The middle ear and mastoid cavity do not lend themselves to direct (noninvasive) examination beyond limited visualization of the middle ear contents through an intact and (sometimes) semitransparent tympanic membrane. The use of rigid telescope endoscopy to evaluate the middle ear has become popular in recent years [4]. However, the technique requires perforation or reflection of the tympanic membrane. Virtual otoscopy can noninvasively provide information about the middle ear and has the advantage of visualization of structures from any desired perspective. The middle ear is normally aerated, making it a potentially good site to study using VE, but the small size of the middle ear space and structures within are technical impediments to adequate virtual representation.
Frankenthaler et al. [49] recently demonstrated successful mapping of the temporal bone using VE. Their group was able to successfully visualize middle ear and mastoid structures using perspective volume rendering techniques. Their methodology required both manual and automatic segmentation of images to iso-
Head and Neck Virtual Endoscopy |
339 |
late and outline the important anatomical structures of interest (ossicles, facial nerve, internal carotid artery, etc.), but it added significantly to the time required to produce images. Although the manual segmentation process was very labor intensive, it provided valuable data. In the temporal bone, simple visualization of the surface morphology of the mastoid cavity and middle ear spaces is relatively easy because of large differences in attenuation between air and soft tissues. However, much more clinically relevant information can be provided by clearly representing the subsurface anatomy of the critical temporal bone structures, including the facial nerve, cochlea, membranous labyrinth, carotid artery, and jugular bulb as described earlier. The ability to visualize the deep, bone-encased structures and to display anatomical relationships with the same view obtained at surgery could provide the surgeon with preoperative diagnostic information and contribute to an enhanced understanding of the anatomical and pathological details that will be encountered at surgery.
VE of the middle and inner ear has been studied very little so far, and its eventual utility remains to be seen. A number of potential otological applications may become available with advances in image acquisition and processing techniques. Detailed evaluation of the cochlea and semicircular canals for persons with vertigo, imaging of the cochlea in cochlear implant candidates, evaluation of congenital atresias or ossicular dislocations, and evaluation and postoperative follow-up of cholesteatomas are all potential future applications. A recent study that provides a dramatic illustration of the potential of VE in otology is provided by Diamantopoulos et al. [71]. MR VE examination of a normal subject was able to display the cochlea, oval window, vestibule, common crus, and the semicircular canals using perspective volume rendering techniques. The ability to accurately image and visualize the anatomy of the cochleovestibular structures with high resolution provides a clear illustration of the untapped potential of VE for diagnosis, teaching, and research applications.
19.3.2 Nasal Cavity and Paranasal Sinuses
The nasal cavity is more accessible to direct visualization than the middle ear, and optical endoscopy of the sinuses is a standard diagnostic modality for the otolaryngologist. However, current endoscopic techniques only allow visualization of the orifices of the maxillary, sphenoid and frontal sinuses, and unless unusually patent or surgically enlarged, direct visualization into the sinuses is not possible. Axial and/or coronal CT studies are used to evaluate sinus pathology and visualize the bony and soft tissue anatomy adjacent to the paranasal sinuses. The images serve both as a preoperative diagnostic tool and an anatomical roadmap during surgery to prevent inadvertent injury to critical structures adjacent to the sinuses during endoscopic surgical procedures. Accurate correlation of the anatomy seen through the telescope at surgery with CT data requires the surgeon
340 |
Armstrong and Nguyen |
to mentally reconstruct the cross-sectional data to precisely determine proximity to vital structures; this considerable skill may only be acquired with experience.
As with other anatomical sites where there is a need to provide accurate 3D reconstructions of complex anatomical structures; VE of the nasal cavity and paranasal sinuses could be a very useful clinical tool. Potential applications of VE in the nasal cavity and sinuses include providing supplemental data to augment standard CT and MR studies, assist presurgical planning, use as an adjunctive tool for intraoperative navigation, and for postoperative monitoring. Gilani et al. (54) have demonstrated high-resolution depictions of the nasal cavity and paranasal sinuses, the ability to view anatomy with unique perspectives, and ability to ‘‘look into’’ the paranasal sinuses with VE [54]. In addition to simply creating an endoscopic visualization of the sinus anatomy, Hopper et al. [56] were able to perform ‘‘virtual tissue removal’’ by first constructing VE reconstructions of the nasal cavity and subsequently electronically removing the middle turbinate and uncinate process. They demonstrated that electronic tissue removal mimicked the findings demonstrated at actual surgery and allowed complete visualization of the infundibulum and the planned surgical site. The importance of their findings should not be discounted. Their work demonstrated the use of VE not only as a novel method to realistically visualize anatomical structures, but also as a tool for planning and rehearsal of surgical procedures. Further refinement could allow surgeons to preoperatively perform complete rehearsals of planned operations at a computer workstation. This ability has potential teaching applications for students as well as for skilled surgeons prior to performing advanced procedures.
19.3.3 Larynx and Pharynx
Several studies have demonstrated the feasibility of VE to evaluate pharyngeal and laryngeal anatomy and pathology. Yumoto et al. provided clear demonstration of the potential of VE to visualize normal anatomy and pathological changes in a series of two normal cases and 10 diseased subjects [72]. They demonstrated that their reconstructed images provided supplemental diagnostic information in cases of vocal cord paralysis but did not provide additional information to axial CT images in the cases of laryngeal cancer. Recently, Fried et al. reported on three cases of VE using CT and MRI data to produce virtual reconstructions [60]. In one of these cases, they demonstrated the ability to fuse the CT and MRI image data into one combined image and utilize this merged data to produce VE images. Using the same techniques reported for temporal bone imaging [49], segmentation of important structures was performed to provide a reconstruction of not only surface anatomy, but also structures surrounding the larynx. These images were stacked to form a 3D structure and subsequently imported into a VE software program to construct endoscopic renderings. Although the process