
Diss / (Springer Series in Information Sciences 25) S. Haykin, J. Litva, T. J. Shepherd (auth.), Professor Simon Haykin, Dr. John Litva, Dr. Terence J. Shepherd (eds.)-Radar Array Processing-Springer-Verlag
.pdf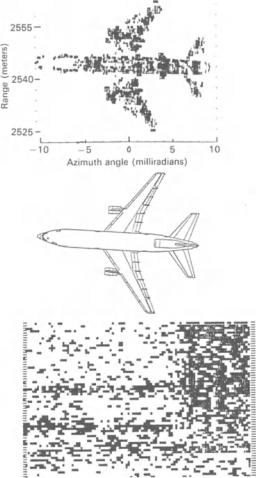
304 B.D. Steinberg
Signal
I
I R, Ri
L |
Beamformer |
|
Array output
Fig. 7.7. Array model and signal processor configuration for self-synchronization. (After [7.6])
The spatial correlation algorithm was developed to handle these cases [7.6]. Figure 7.7 sketches a periodic array of N elements steered to 80 the angie from broadside. The phase-shift fjJn in the nth channel is nkduo where k = 21t/Ais the wavenumber, d is the element spacing and Uo = sin 80 , There is a phase error ofjJn in the nth channel, resulting both from element position error and electrical mistuning. The left channel is considered the reference. Its phase error is arbitrarily assigned the value of O. In each channel except the reference channel is a phase-error correcting weight. The sum is the array output.
Information for determining the correct values of the weights is obtained from the products of signals in adjacent channels. The first product is eoet; the second product is el e! ; and so on. Each product is integrated, or averaged over J range bins:
~ |
|
1 |
J |
.et . |
(7.1) |
Ro |
. |
1 = - |
L eo |
||
|
J |
j= 1 |
,J ,J |
|
is an estimate of the correlation coefficient of the radiation field between the zeroth and first antenna elements modified by the phase error OfjJ. Its expectation is
|
(7.2) |
Therefore, |
|
Ro.l :::: Ro, 1 exp( -jofjJd |
|
R1,2 :::: Rl,2 exP[j(OfjJl - OfjJ2)J |
|
Rn•n +l :::: Rn.n+ l exp[j(ofjJn - ofjJn+dJ· |
(7.3) |
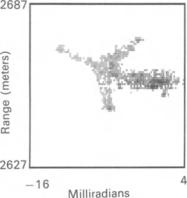
7. The Radio Camera |
307 |
7.6 Number of Elements
Array thinning is essential in the radio camera. The resolution of its array is determined by its length, and not the number of elements. The latter determines its SNR gain, and its sidelobe or grating lobe properties. The SNR gain of a receiving array is directly proportional to N. The effect upon sidelobe level and the existence of grating lobes is more complicated, and is our primary concern. Grating lobes are formed when thinning is periodic, i.e., the inter-element space d remains constant, but is considerably larger than the "filled" spacing A/2. The radiation pattern
(7.5)
consists of the patternfo(u) of the filled array repeated at intervals of Ajd. There are two conditions under which (7.5) is an acceptable pattern for
imaging purposes. The first exists when the transmitter beamwidth is smaller than the lobe spacing A./d. This occurs when the transmitting antenna is larger than d, in which case, only a single lobe of(7.5) is illuminated by the transmitter. Because no scatterers outside the central lobe are excited, no lobe ambiguity results, nor does undesired back-scattered energy from the central lobe enter the system. This procedure was used in taking data for the image of Fig. 7.3. The transmitting antenna was a 1.2 m diameter dish; the receiving array sampled the radiation field every 25 cm, which was 8 wavelengths.
The second condition under which grating lobes are acceptable is when the target is smaller than A/d, and is free of a clutter background. In this case, the transmitter may illuminate a sector containing several ofthe receiving lobes, but because no scatterers are outside the central lobe, no ambiguity results. The data for Fig. 7.5 were obtained in this manner. The transmitting antenna, again, was a 1.2 m dish; the receiving samples were spaced at about 0.75 m, or 24 wavelengths.
Periodic thinning and grating lobe suppression, as previously described, preserves satisfactory sidelobe characteristics in the neighborhood of the main lobe. When circumstances do not permit periodic thinning, an aperiodic procedure such as random thinning must be employed. Aperiodic thinning destroys grating lobes, but not the energy in the grating lobes. The result is undesirably high sidelobes. The average sidelobe power level is N- 1, and the peak sidelobe is typically about 10 dB higher [7.1]. When random thinning is employed, the system must be designed to tolerate the high sidelobe level.
One favorable aspect of random thinning is that the resolution and sidelobe properties of the radiation pattern are almost totally decoupled. The angular resolution remains A./L, where L is the length over which the elements are randomly deployed. The average sidelobe level is N - 1 independent of the length, while the peak sidelobe grows very slowly with length; the relationship is logarithmic. Thus, once the penalty for using a random array is paid, and N is
308 B.D. Steinberg
determined by the tolerable sidelobe level, the aperture size can be made almost arbitrarily large. In essence, the higher the resolution, the higher the thinning factor. N is determined by the tolerable sidelobe level which, in tum, is governed by the required dynamic range in the image. For an imaging problem in which a 20 dB dynamic range is desired, about 1000 elements are required.
7.7 Number of Bits per Sample
Savings can also be affected by reducing b, the number of bits per sample. Figure 7.11 is a repeat of Fig. 7.3, but with a different data quantization procedure. For Fig. 7.3, the I and Q samples were each quantized to 8 bits. For Fig. 7.11, they were each quantized to 1 bit, which is a data reduction of 8: 1. Furthermore, the Fourier kernel of the image processor was similarly quantized to 2 bits, thereby eliminating the need for all complex multiplication in the signal processor. Comparison of the images show them to be nearly identical, and equally good. Their resolutions are the same, and the pixel intensities and locations are almost identical. The reader is referred to [7.9] for the theory of data compression in microwave imaging, and examples of several combinations of quantization of amplitude and phase.
7.8 Data Truncation
Array thinning and bit compression offer about three orders of magnitude reduction in data handling for the example given in the introduction. While it is a large reduction, it is still insufficient, for the reduced NBb product still exceeds
,.. |
|
|
.,. c |
|
|
\' |
|
-4490 |
|
". ,. |
I. ~. !~. |
|
\.. |
|
|
||
|
|
• |
,,·tll~ |
... |
,.:~ |
|
-4450 |
|
|
|
.••~I |
J~, |
|
• |
" |
|
|
|
|
., |
|
|
|
-4400 |
||
|
|
|
\" |
"~ , |
·t· " |
.' ,4350 |
||
I |
I |
|
, |
,. ","w: |
||||
|
I |
|
|
I |
|
40 |
||
0 |
10 |
|
20 |
|
|
30 |
|
Azimuth (radians)
Fig. 7.11. Example of bit compression and simplification of the radio camera signal processor. Image of the housing development of Fig. 7.3. Input data to the phased array are hard limited and quantized to two bits of phase. The kernel of the signal processor is also quantized to two bits of phase. (From [7.9])
7. The Radio Camera |
309 |
1011 bps. The input data to the high-resolution signal processor must be truncated to reduce the average rate to an acceptable level. In discussing the problem, [7.9] recommends a low or conventional resolution screening process to discover those targets or volume elements in space for which high-resolution imagery is desirable. Only those targets are processed by the high-resolution signal processor. The system consists of a scanning radar operating in conventional mode. Input data are also passed to a temporary buffer store with highresolution capability and limited capacity. The input data reside in store only long enough for the main receiver to decide whether or not a high-resolution image is called for. Most of the data are discarded. The undiscarded data are read out at a modest rate acceptable to the signal processor. By these three procedures, the total average data rate may be kept within bounds.
7.9 Conclusions
The radio camera is shown to produce very high-resolution images of targets and scenes from microwave radar data. The huge aperture that is necessary to achieve the high-resolution is self-calibrated by a process called adaptive beamforming (ABF). ABF deduces the required corrections for the phase errors in the array, directly from the back-scattered radiation field.
One ABF procedure utilizes the known spherical wavefront of the reradiation from a point reflector. It is called the dominant scatterer algorithm. The second procedure operates upon the spatial correlation function of the reradiation field from a quasi-homogeneous distribution of scatterers. It is called the spatial correlation algorithm.
Data rate reduction is essential for practical utilization of the radio camera techniques. Array thinning, bit compression, and data truncation are discussed for this purpose.
References
7.1B.D. Steinberg: Principles ofAperture and Array System Design: Including Random and Adaptive Arrays (Wiley, New York 1976)
7.2B.D. Steinberg: Microwave Imaging with Large Antenna Arrays: Radio Camera Principles and Techniques (Wiley, New York 1983)
7.3A.V. Oppenheim, J.S. Lim: The Importance of Phase in Signals. Proc. IEEE 69, 529-541 (1981)
7.4B.D. Steinberg, J. Tsao, D. Carlson, T. Seeleman: Imaging experiments. Valley Forge Research Center Quarterly Progress Report No. 45, University of Pennsylvania (1984) pp. 1-13
7.5B.D. Steinberg: Microwave imaging of aircraft. Proc. IEEE 76, 1578-1592 (1988)
7.6E.H. Attia, B.D. Steinberg: Self-Cohering Large Antenna Arrays Using the Spatial Correlation Properties of Radar Clutter. IEEE Trans. AP-37, 30-38 (1989)
7.7E.H. Attia: Phase synchronizing large antenna arrays using the spatial correlation properties of radar clutter. Ph.D. Dissertation, University of Pennsylvania (1984)
310 B.D. Steinberg
7.8B. Kang, B.D. Steinberg: Self-calibration of phased arrays using the modified Muller-Buffing- ton theorem and the spatial correlation algorithm, Valley Forge Research Center Quarterly Progress Report No. 54, University of Pennsylvania (1988) pp. 32-45
7.9B.D. Steinberg: A theory of the effect of hard limiting and other distortions upon the quality of microwave images. IEEE Trans. ASSP-3S, 1462-1472 (1987)
7.10B.D. Steinberg, H.M.S.: Microwave Imaging Techniques (Wiley, New York 1991)