
Учебник по НТП
.pdf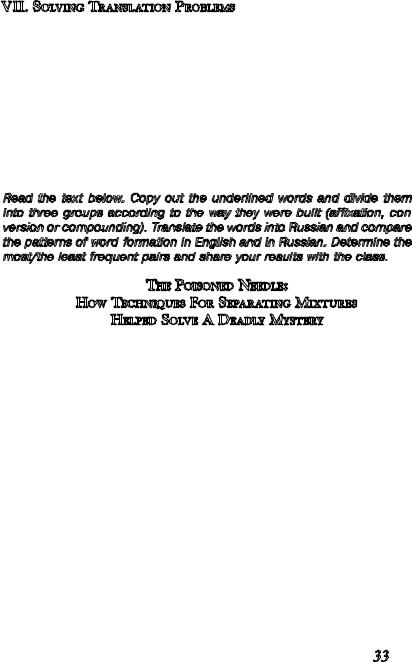
VII. Solving Translation Problems
The most widely used ways of word-building in English include affixation (work–worker), conversion (slow–to slow) and compounding (street+lamp = streetlamp). Close parallels between some English and Russian affixes (i.e. nation–нация, reddish–красноватый, etc.) hardly present any serious difficulties for translators. However, in other cases, especially those of compounding and conversion, finding EnglishRussian equivalents, whenever both the form and the meaning of a given word count, can become a tough problem. Thus, a key to successful translation might be found in development of skills in word analysis with the clear understanding of connections between the two languages.
Read the text below. Copy out the underlined words and divide them into three groups according to the way they were built (affixation, con- version or compounding). Translate the words into Russian and compare the patterns of word-formation in English and in Russian. Determine the most/the least frequent pairs and share your results with the class.
The Poisoned Needle:
How Techniques For Separating Mixtures
Helped Solve A Deadly Mystery
One morning in the summer of 1961, hundreds of crazed birds attacked the seaside town of Capitola, California. The birds crashed through glass windows and attacked people on the ground. Most of the birds were sooty shearwaters, a normally nonaggressive species that feeds on small fish and comes ashore only to breed. The incident fascinated Alfred Hitchcock, who frequently vacationed in nearby Santa Cruz. He included newspaper clippings about the Capitola attack in his studio proposal for
The Birds, which appeared in cinemas two years later.
In the winter of 1987, the agent that is now believed to be responsible for the Capitola incident struck on the opposite shore of the continent. Over a hundred people became extremely ill within hours after dining on cultured blue mussels in restaurants around Prince Edward Island in Canada. A tragic symptom of poisoning was the destruction of short term memory in about one quarter of the survivors. The mysterious syndrome was called "amnesic shellfish poisoning". This sort of neurological damage due to food poisoning had never been encountered before. A team of marine biologists and chemists was assembled by Canada's Department of Fisheries and Oceans (DFO) to work on the problem.
But an initial screening of the sample for known bacterial and viral pathogens revealed nothing. Tests for heavy metals, pesticides, and
33

PCBs also were negative. The mussel samples were extremely complex, containing thousands of different chemical compounds. How can one component be isolated from a such a complex mixture, without knowing anything about its physical or chemical properties? How do you find a needle in a haystack, when you've never seen a needle before? Suppose the haystack could be divided in half, and the half that tested negative for the needle could be discarded. Repeating this di- vide-and-discard process over and over again should eventually result in a pile with only one thing left: the needle. That was the strategy the researchers used to isolate the toxin. A biological test was developed to inject a small amount of the sample into mice for producing a very distinctive neurological reaction if the toxin was present. Standard physical methods for separating complex mixtures were applied to the poisoned mussel samples. At the same time, uncontaminated mussels were subjected to the same separations, to allow the analysts to compare fractions. Any differences in spectra or chromatograms between the control and toxic samples might be valuable clues in the search for the toxic agent. Mice were exposed to each fraction of the separation. Fractions found to be toxic were retained for further analysis. The others were discarded. If chromatograms and spectra indicated that the toxic fraction was still a complex mixture, another separation technique was applied:
34

Most drugs and poisons are either fat-soluble or water-soluble, so a logical first step in the isolation was solvent extraction. To prevent potential decomposition of the compound by heat or harsh solvents, ground mussel samples were extracted at room temperature with aqueous methanol, a mild solvent. The extraction was inefficient but successful: mice had the same neurological reaction to the methanol extract that they had to the original mussel samples. The extract was concentrated by evaporation. The vapor was not toxic, but the residue after evaporation was. The poison apparently was nonvolatile, which could indicate a high molecular weight compound, or a compound that ionized in solution.
A second extraction was performed by shaking the concentrated extract with a mixture of a nonpolar solvent (dichloromethane) and water, which is polar. The dichloromethane fraction gave a negative result in the mouse bioassay. The aqueous layer contained the toxin, indicating that it was probably a polar, ionizable substance. This was a lucky break, because the researchers could discard the complex dichloromethane fraction and concentrate on the much simpler aqueous fraction.
Column chromatography was used to separate the aqueous layer into simpler components. The sample was passed through a narrow tube packed with beads of a resin called XAD-2, which grabs the nonpolar parts of passing molecules, but lets ions pass freely. Of the many fractions that passed out of the XAD-2 column, only one was toxic. For the final stage of the purification, the toxic fraction was separated with high performance liquid chromatography (HPLC).
Again, a polar solution containing the sample was passed through a column packed with a nonpolar stationary phase. A single, highly purified fraction collected from the HPLC column accounted for all of the toxicity present in the original mussel sample, and the toxin was isolated. Then, mass spectrometry was used to determine the toxin's molecular weight (312 g/mol) and molecular formula (C15H22NO6). Spectroscopic analysis revealed the presence of conjugated double bonds and features characteristic of an amino acid. By matching the spectra with those from STN International Registry system, the compound was unambiguously identified as domoic acid, an triprotic amino acid.
Domoic acid is a molecular Trojan Horse. Nerve cells mistakenly recognize domoic acid as glutamic acid—a fatal error. Domoic acid's structure is obviously similar to glutamic acid. But its five-sided ring makes it less flexible than glutamate, which causes it to bind very tightly to glutamate receptors. As a result, the damaging effect of domoate is 30 to 100 times more powerful than that of glutamate.
35
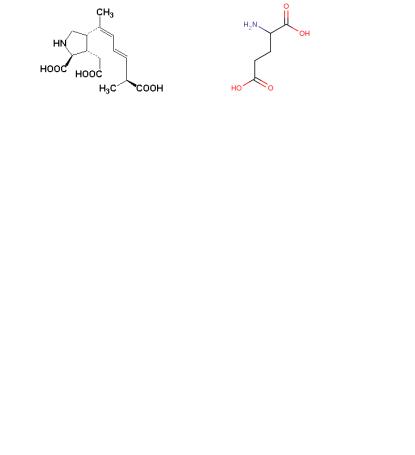
Domoic acid |
Glutamic acid |
How did the domoic acid get into the shellfish (and the anchovies eaten by the birds at Capitola)? Remember that phytoplankton pigments were found in the aqueous layer after solvent extraction. This wasn't quite a smoking gun, but it was definitely a fingerprint of the killer. An extensive investigation traced the domoic acid to an obscure species of needle-like diatom, called Pseudo-nitzschia pungens. Pseudo-nitzschia has been found in oceans around the world, so further outbreaks are possible in many locations. Commercial shellfish and seafood is now monitored regularly for domoic acid, using HPLC to identify the toxin. The screening and testing procedures have so far been successfulnot a single instance of domoic acid poisoning in humans has been reported since the 1987 outbreak.
To most people solutions mean finding the answer, but to
chemists solutions are things that are still all mixed up...
VIII. Mastering English Grammar
Translate the sentences paying special attention to the equivalent-lack- ing grammatical structures:
1. If you’ve ever fumbled with a ring of nearly identical keys, you understand that a subtle difference in an object’s shape can make a large difference in the way it functions.
2.A similar lock-and-key type of model has been used to explain why different substances have different flavors.
3.These seven receptors were believed to be the only letters in the olfactory alphabet in the early 1970’s. Now we know about over a thousand olfactory receptors, not just seven.
4.Perfume chemists have long known that adding a hydrocarbon tail to some perfume molecules increases their potency.
5.The higher molecular weight of zingerone in combination with the
36

polar side-chain carbonyl group makes zingerone molecules attract each other more strongly than eugenol and vanillin molecules do.
6.The fat solubility allows eugenol to penetrate tissues and bind more tightly to the vanilloid receptor, which is believed to have a fatty side chain.
7.Most (though not all) of the hot in hot peppers comes from capsaicin and a closely related compound, dihydrocapsaicin.
8.The compound’s molecular weight is the highest of any of the vanilloids we’ve looked at so far, and the side chain contains a polar amide (-NHCO-) group. That makes capsaicin’s volatility very low, and it is completely odorless.
9.One expects that the long hydrocarbon tail will make capsaicin less water soluble than vanillin. This is indeed the case.
10.This is why drinking water after munching an habanero pepper won’t stop the burning. For relief from a chile burn, drink milk, which contains casein, a lipophilic (fat-loving) substance that surrounds and washes away the fatty capsaicin molecules in much the same way that soap washes away grease.
IX. Fostering Critical Thinking Skills
Read the text. Find additional material
to expand the topic and write a commented essay in Russian on Chemistry of Taste and Smell:
The Molecular Basis For Flavor
If you’ve ever fumbled with a ring of nearly identical keys, you understand that a subtle difference in an object’s shape can make a large difference in the way it functions. Living things recognize molecules in much the same way that locks “recognize” keys—by shape. A similar lock-and-key type of model has been used to explain why different substances have different flavors. The stereochemical theory of odor suggests that a molecule that fits into an olfactory receptor can fire
nerve cells, ultimately producing a particular odor perception.
Five basic odors were associated with different molecular shapes. Football shaped molecules fit in to a “camphoraceous” receptor, and smell like mothballs. Necklace-shaped molecules have a musky odor because they fit into a “musky” receptor. Wedged-shaped molecules have a pepperminty odor, tadpole-shaped molecules smell like flowers, and long thin ether molecules are ethereal. Putrid and pungent smells were explained on the basis of partial charges on atoms within the molecule, rather than by shape alone. Putrid molecules have a buildup of negative charge somewhere in the molecule that’s strongly attracted to a partially positive site on the “putrid” receptor. Pungent molecules (like acetic acid, in vinegar) are just the opposite: they have an electron-deficient region that is
37
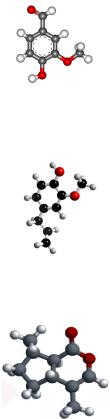
strongly attracted to an electron-rich site on the “pungent” receptor. These seven receptors were believed to be the only letters in the olfactory alphabet in the early 1970’s. Now we know about over a thousand olfactory receptors, not just seven. The molecule’s ability to move through tissue containing layer after layer of receptors also determines how its odor is perceived. Perfume chemists have long known that adding a hydrocarbon tail to some perfume molecules increases their potency.
Let’s look at some specific examples. The vanilloids (vanillin, eugenol, zingerone, and capsaicin) are molecules with distinctive flavors but obviously similar molecular structures. All contain a six-sided hexagonal ring of carbons (called a benzene ring). Subtle changes in the sizes or positions of groups of atoms attached to the ring dramatically change the compound’s flavor.
Vanillin has a soothing, pleasant aroma. Its molecular weight is relatively low, and it is fairly volatile. Molecules containing only carbon and hydrogen are mostly insoluble in water. The oxygen-contain- ing groups attached to the ring in vanillin can form strong hydrogen bonds with water. Vanillin’s solubility in water is responsible for the “finish” acquired by wines aged in oak casks. Vanilla present in the wood lignin of the wine barrels slowly leaches into the wine over time.
Eugenol is found in bay leaves, allspice, and oil of cloves. Eugenol has a short hydrocarbon chain attached to the ring, which makes it practically insoluble in water, though it freely mixes with fats and oils.Its fat solubility allows it to penetrate tissues and bind more tightly to the vanilloid receptor, which is believed to have a fatty side chain. The tail gives eugenol a stronger odor than vanillin has. One bay leaf is enough to season a pot of soup; more than one or two ground cloves overpower a pumpkin pie. Eugenol has a numbing, analgesic effect to be used as a dental antiseptic. The hydrocarbon tail in combination with the polar OH group on the ring make eugenol rather soap-like to disrupt the cell membranes of bacteria the way soap disrupts a spot of grease.
Zingerone puts the zing in ginger and is also a flavor ingredient in mustard oil. Zingerone is sparingly soluble in water, but also freely soluble
38

in fats and oils. The hydrocarbon tail attached to its vanillin foundation ring doesn’t lower the solubility of zingerone because it contains a carbonyl group (C=O) that can form strong hydrogen bonds with water molecules. The higher molecular weight of zingerone in combination with the polar side-chain carbonyl group makes zingerone molecules attract each other more strongly than eugenol and vanillin molecules do. As a result, zingerone is less volatile than either eugenol or vanillin. Zingerone reacts with free radicals that can cause tissue damage and inflammation. Some studies show that a topically applied extract containing zingerone may help prevent some skin cancers.
Most (though not all) of the hot in hot peppers comes from capsaicin and a closely related compound, dihydrocapsaicin. It occurs in much lower quantities in oregano, cinnamon, and cilantro. The compound’s molecular weight is the highest of any of the vanilloids we’ve looked at so far, and the side chain contains a polar amide (-NHCO-) group.
That makes capsaicin’s volatility very low, and it is completely odorless. Though without a telltale fragrance, capsaicin’s presence in foods is hard to miss. A solution that contains only 10 parts per million produces a persistent burning sensation when placed on the tongue. The intense flavor results from the molecule’s long hydrocarbon tail. The chain allows it to bind very strongly with its lipoprotein receptor, which has some hydrocarbon side chains of its own.
The fatty tail also allows the molecule to slip through lipid-rich cell membranes, making the burn more pervasive and persistent. Several capsaicin-like compounds found in chiles have slight structural variations in the hydrocarbon tail, which changes their ability to bind to the receptors and their ability to penetrate layers of receptors on the tongue, mouth, and throat. That may explain why some chiles burn in the mouth, while others burn deep in the throat. The perception that peppers are “hot” is not an accident. The capsaicin key opens a door in the cell membrane that allows calcium ions to flood into the cell. That ultimately triggers a pain signal that is transmitted to the next cell. When the cells are exposed to heat, the same events occur. Chile burns and heat burns are similar at the molecular, cellular, and sensory levels.
One expects that the long hydrocarbon tail will make capsaicin less water soluble than vanillin. This is indeed the case. Capsaicin is insoluble in cold water, but freely soluble in alcohol and vegetable oils. This is why drinking water after munching an habanero pepper won’t stop the burning. For relief from a chile burn, drink milk, which contains casein, a lipophilic (fat-loving) substance that surrounds and washes away the fatty capsaicin molecules in much the same way that soap washes away grease. High concentrations are toxic. Exposure is painful and even incapacitating. Capsaicin prevents nerve cells from communicating with each other by blocking the production of certain neurotransmitters; at
39

high concentrations it destroys the cells! Capsaicin’s toxicity makes chiles more than just a foodthey’re also a weapon. The Mayans burned chiles to create a stinging smoke screen, and threw gourds filled with pepper extract in battle. Nowadays, capsaicin is the active ingredient in pepper sprays, used to ward off attacking muggers, dogs, and bears
Paradoxically, capsaicin’s ability to cause pain makes it useful in alleviating pain. Exposure to capsaicin lowers sensitivity to pain, and it is applied as a counter irritant in the treatment of arthritis and other chronically painful conditions. People that eat lots of spicy capsaicin-rich foods build up a tolerance to it. The incentive: a small jolt of capsaicin excites the nervous system into producing endorphins, which promote a pleasant sense of well-being. The endorphin lift makes spicy foods mildly addictive (and for some, an obsession).
X. Organizing Ideas
Make a concept map on Chemical Science and fill
it with basic notions, associated words and phrases you’ve learned in this unit.
40

Unit III
Mathematics
I. Getting Started
Read the text “Algebraic Expressions“. Divide it into several key parts and compose 3-5 questions to the each part. Put your questions to class.
II. Working With Vocabulary
Place the words and phrases below into the “Word“ column and com- plete the table:
Word |
English |
Examples |
Russian |
|
definition |
of usage |
translation |
||
|
algebra, formal language, term, string of symbols, syntax of the language, variables, constants, transformational rule, operating symbol, term, equal sign, coefficient, numbers in disguise, real number, integer, fraction, decimal (noun), non-terminating decimal number, rational number, quotient, irrational number, shorthand notation, delimiter, exponent, binomial expansion, multiplication operator, corollary, factoring, multiplying through.
III. Practising Translation Techniques
Make a written translation of the following text:
Algebraic Expressions
Algebra is a formal symbolic language, composed of strings of symbols. Some strings of symbols form sentences within the language (X + Y = Z), while others do not (X += Y Z). The set of rules that determines which
strings belong to the language and which do not, is called the syntax of the language. Transformational rules change a given sentence in the language into another sentence without changing the meaning of the sentence.
Math problems?
Call 1-800-[(10x)(13i)2]-[sin(xy)/2.362x]
41

Algebraic expressions can include variables, constants, operating symbols, such as plus and minus signs. It’s only a phrase, not the whole sentence, so it doesn’t include an equal sign:
3x2 + 2y + 7xy + 5
In an algebraic expression, terms are the elements separated by the plus or minus signs. This example has four terms, 3x2, 2y, 7xy, and 5. Terms may consist of variables and coefficients, or constants.
In algebraic expressions, letters represent variables. These letters are actually numbers in disguise. In this expression, the variables are x and y. We call these letters “variables” because the numbers they represent can vary—that is, we can substitute one or more numbers for the letters in the expression. Coefficients are the number part of the terms with variables.
In 3x2 + 2y + 7xy + 5,
the coefficient of the first term is 3. The coefficient of the second term is 2, and the coefficient of the third term is 7. If a term consists of only variables, its coefficient is 1.
Constants are the terms in the algebraic expression that contain only numbers. That is, they’re the terms without variables. We call them constants because their value never changes, since there are no variables in the term that can change its value. In the expression 7x2 + 3xy + 8 the constant term is “8.” In algebra, we work with the set of real numbers, which we can model using a number line:
Real numbers describe real-world quantities such as amounts, distances, age, temperature, and so on. A real number can be an integer, a fraction, or a decimal. They can also be either rational or irrational. Numbers that are not “real” are called imaginary. Imaginary numbers are used by mathematicians to describe numbers that cannot be found on the number line. We call the set of real integers and fractions “rational numbers.” Rational comes from the word “ratio” because a rational number can always be written as the ratio, or quotient, of two integers.
Examples of rational numbers: The fraction ½ is the ratio of 1 to 2; the number “0.57” is also a rational number, as it can be written as a fraction.
Some real numbers can’t be expressed as a quotient of two integers. We call these numbers “irrational numbers”. The decimal form of an irra-
42