
Yang Fluidization, Solids Handling, and Processing
.pdf
Pressure and Temperature Effects 135
jets at the grid which accelerate the particles and cause them to collide with others at a very high velocity.
Sishtla et al. (1989) reported that the particle attrition rate at the grid is proportional to the kinetic energy of the gas jets (ρgUj2) issuing from the grid. They varied both system pressure and temperature while maintaining gas velocity constant, and found that increasing system pressure resulted in an increase in the attrition rate (Fig. 16), while increasing system temperature decreased the rate of particle attrition (Fig. 17). They attributed this behavior to the change in gas density produced by changes in temperature and pressure. Kono (1981) also observed the same effects of temperature and pressure on mechanical attrition.
Fines Generated, %
|
|
Material: 65% Coke Breeze/35% Limestone |
|||||
|
|
Particle Size: 1000 microns |
|
|
|||
70 |
|
Column Diameter: 15 cm |
|
|
|||
|
Gas: Nitrogen |
|
|
|
|
||
|
|
|
|
|
|
||
|
|
Fluidizing Velocity: |
0.46 |
|
|
||
60 |
|
Grid Type: Bubble Cap |
|
|
|||
|
|
|
|
|
|
|
|
50 |
|
|
|
|
Pressure |
|
|
|
|
|
|
|
1 bar |
|
|
|
|
|
|
|
|
|
|
|
|
|
|
|
|
4 bar |
|
|
|
|
|
|
|
12 bar |
|
40 |
|
|
|
|
|
|
|
30 |
|
|
|
|
|
|
|
20 |
|
|
|
|
|
|
|
10 |
|
|
|
|
|
|
|
0 |
|
|
|
|
|
|
|
0 |
2 |
4 |
6 |
8 |
10 |
12 |
14 |
Time, h
Figure 16. The effect of system pressure on attrition at the grid. (Sishtla, et al.)
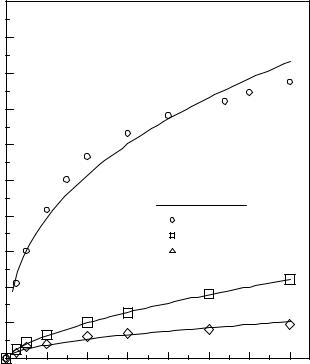
136 Fluidization, Solids Handling, and Processing
1.8Particle Attrition in Cyclones
There can also be substantial particle attrition in cyclones in fluidized-bed systems because particles are accelerated at the inlet of the cyclone and impacted against the cyclone wall. Although there is little information on particle attrition in cyclones in the literature, it has been reported (Sishtla) that increasing system pressure decreases the attrition rate in cyclones operating with coal char. The mechanism by which this occurred was not determined.
|
100 |
|
|
|
|
|
|
|
|
|
Material: Lignite Char |
|
|
|
|
||
|
90 |
Gas: Nitrogen |
|
|
|
|
|
|
|
|
Column Diameter: 15 cm |
|
|
|
|
||
|
80 |
Velocity: |
0.46 m/s |
|
|
|
|
|
|
|
Bed Height: 30 cm |
|
|
|
|
||
% |
70 |
Particle Diameter: |
|
|
|
|
|
|
Generatied, |
|
1000 microns |
|
|
|
|
|
|
60 |
|
|
|
|
|
|
|
|
50 |
|
|
|
|
|
|
|
|
Fines |
|
|
|
|
|
o |
|
|
|
|
|
|
Temperature, C |
|
|
||
40 |
|
|
|
|
21 |
|
|
|
|
|
|
|
|
|
|
||
|
|
|
|
|
|
|
|
|
|
|
|
|
|
|
427 |
|
|
|
30 |
|
|
|
|
871 |
|
|
|
20 |
|
|
|
|
|
|
|
|
10 |
|
|
|
|
|
|
|
|
0 |
|
|
|
|
|
|
|
|
0 |
2 |
4 |
6 |
8 |
10 |
12 |
14 |
Time, h
Figure 17. The effect of temperature on attrition at the grid. (Sishtla et al.)

Pressure and Temperature Effects 137
1.9Jet Penetration
Vertical jet penetration in fluidized beds increases significantly with system pressure as shown in Fig. 18. Vertical jets fluctuate in the vertical direction between a minimum and a maximum value. Most jet penetration correlations are given in terms of the maximum jet penetration length which is designated Lmax (Hirsan et al. 1980). Over a pressure range of 1 to 50 bar with jet nozzles up to 3.8 cm in diameter, Hirsan et al. (1980) developed the following empirical correlation for the maximum jet penetration.
|
Lmax |
|
é |
ρg Uo |
||
Eq. (13) |
= 19.3 |
ê |
||||
|
|
|
||||
d o |
|
ê |
ρp gd p |
|||
|
|
ê |
||||
|
|
|
ë |
|
|
ù |
0.83 |
|
|
ú |
éUcf |
||
ú |
ê |
|
|
U |
|||
ê |
|||
ú |
ë |
|
|
û |
|
|
ù 0.54
ú
úû
This correlation predicts that the maximum vertical jet penetration into a fluidized bed varies with gas density to the 0.67 power, and decreases with increasing fluidizing gas velocity and increasing particle diameter.
Yang (1981) incorporated the high-pressure data of Hirsan et al. (1980) to produce the following correlation for Lmax
|
L |
|
|
éæ |
1 |
öæ |
|
|
|
|
ρ |
öæ |
U 2 |
öù0.472 |
|
Eq. (14) |
max |
= |
êç |
֍ |
|
|
|
|
g |
֍ |
o |
÷ú |
|||
d |
o |
7.65 |
ç R |
֍ |
|
ρ |
p |
- ρ |
֍ gd |
÷ |
|||||
|
|
|
êè |
cf øè |
|
|
g ø |
o |
ú |
||||||
|
|
|
|
ë |
|
|
|
|
|
|
|
|
è |
|
øû |
where Rcf is defined as: |
|
|
|
|
|
|
|
|
|
|
|
||||
|
Rcf |
= |
(U cf |
) |
pressure |
|
|
|
|
|
|||||
Eq. (15) |
|
|
|
|
|
|
|
||||||||
|
|
|
|
|
|
|
|
|
|
|
|
||||
(U cf |
) |
ambient |
|
|
|
|
|
|
|
||||||
|
|
|
|
|
|
|
|
|
|
|
|||||
|
|
|
|
|
|
|
|
|
|
|
|
|
Yates et al. (1986) varied system pressure up to 20 bar with jet nozzles of 2 and 4 mm diameter, and also found that the maximum jet penetration varied significantly with pressure. They then developed the following correlation for the maximum jet penetration:

138 Fluidization, Solids Handling, and Processing
|
L |
|
éæ |
1 |
öæ |
|
|
ρg |
öæ |
U 2 |
öù0.38 |
|
Eq. (16) |
max |
= 9.77êç |
֍ |
|
|
|
֍ |
o |
|
÷ú |
||
|
|
ρ |
|
- ρ |
|
|
||||||
d |
o |
ç R |
֍ |
p |
֍ gd |
o |
÷ |
|||||
|
|
êè |
cf øè |
|
g ø |
|
ú |
|||||
|
|
|
ë |
|
|
|
|
|
è |
|
|
øû |
which is similar in form to the correlation of Yang. Yates and Cheesman also incorporated high-temperature jet penetration data (obtained at temperatures up to 800°C) into this correlation.
|
|
Particle Size: 400 microns |
|
|
|
|
|
Gas: Nitrogen |
|
|
|
|
|
Nozzle Diameter: 2.54 cm |
|
|
|
|
|
Jet Velocity: 7.6 m/s |
|
|
|
|
30 |
Fluidization Velocity: U |
cf |
|
|
|
|
|
|
|
|
o |
|
|
|
|
|
/d |
20 |
|
|
|
|
b |
|
|
|
|
|
L |
|
|
|
|
|
|
|
|
|
|
|
|
10 |
|
Material |
Particle Density, g/cc |
|
|
|
|
|
|
|
|
|
|
FMC Char |
1.2 |
|
|
|
|
Sand |
2.6 |
|
|
|
|
Siderite |
4.0 |
|
|
0 |
|
|
|
|
|
0 |
10 |
20 |
30 |
40 |
Pressure, bar
Figure 18. The effect of pressure on jet penetration. (Knowlton and Hirsan.)
Findlay and Knowlton (1985) investigated the effect of gas viscosity on jet penetration by varying system temperature while maintaining gas density constant. This was accomplished by adjusting system pressure at
Pressure and Temperature Effects 139
each temperature studied. They concluded that viscosity had a negligible effect on jet penetration, and that temperature only affects jet penetration through its effect on gas density.
1.10 Regime Transitions
The transition boundaries between various fluidization regimes (i.e., bubbling/slugging to turbulent, and turbulent to fast fluidization) are also affected by gas properties. Because the minimum fluidization velocity changes with pressure for large particles, it would be expected that the transition velocities where the bed changes from the bubbling to the turbulent regime, and where the turbulent regime changes to the fast fluidization regime, would also be affected by system pressure. Indeed this is the case.
If the superficial gas velocity of a fluidized bed operating in a bubbling/slugging mode is increased, the bed is gradually transformed into a turbulent fluidized bed. The transition to turbulence occurs over a velocity range which is bounded by two unique gas velocities: Uc, the velocity at which the bed starts the transition from bubbling/slugging to turbulent, and Uk , the velocity at which the bed is fully at turbulent fluidization. Uc is often taken to be where the amplitude of the differential-pressure fluctuations in the bed are highest, and Uk is taken to be the velocity at which the amplitude of the differential-pressure fluctuations level off as indicated in Fig. 19. Recently, Marzocchella and Salatino (1996) reported that Uc and Uk can also be determined by plotting the variance of the pressure drop across the bed versus the superficial gas velocity through the bed. They conducted their tests with 175-micron glass beads fluidized by CO2 at 40 and 60 bar pressures. They found that Uc occurred at the maximum value of the variance of the pressure drop across the bed versus superficial gas velocity curve, while Uk was determined where the variance of the pressure drop across the bed levels off (Fig. 20). This figure shows that Uc and Uk both decrease with increasing pressure. Marzocchella and Salatino also showed that power spectral densities of signals from a hot-wire anemometer inserted into the fluidized bed also can also be used to determine the packed-bed, particulate, bubbling, and turbulent regions in the fluidized bed.
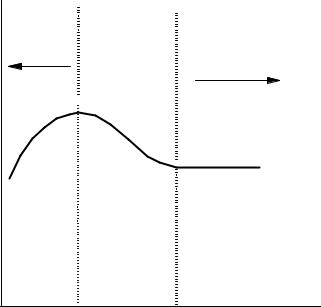
140 Fluidization, Solids Handling, and Processing
Several other researchers have investigated the effects of pressure as well as temperature on the transition from bubbling/slugging to turbulent fluidization (Cai et al., 1989; Canada and McLaughlin, 1978; Yang and Chitester, 1988). Cai et al. (1989) varied system pressure over a range of 1 to 6 atmospheres for solids with different average particle sizes. They found that Uc decreased with system pressure. Yang and Chitester (1988) varied system pressure up to 64 atmospheres and also reported that increasing pressure caused a decrease in the velocity at which the transition from bubbling to turbulent fluidization occurred.
Magnitude of Pressure DropFluctuations |
Bubbling/ |
Transition |
|
|
Slugging |
|
|||
Region |
Turbulent |
|||
|
||||
|
|
|||
|
|
|
||
|
|
U c |
U k |
Gas Velocity
Figure 19. Bubbling-to-turbulent transition.
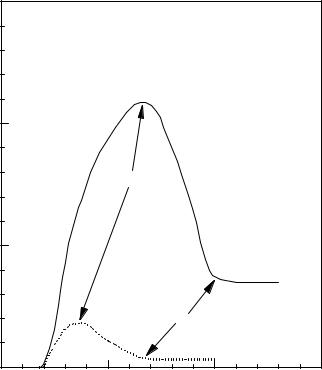
Pressure and Temperature Effects 141
Variance of Pressure Drop, mbar 2
1.5
Material: Glass Beads Size: 175 Microns Gas: Carbon Dioxide Bed Diameter: 30 mm
1
Uc
0.5
40 Bar
Uk
60 Bar
0
0 |
10 |
20 |
30 |
Superficial Gas Velocity, cm/s
Figure 20. The variance of bed pressure drop versus superficial gas velocity.
(Marzocchella & Salatino.)
Canada and McLaughlin (1978), working with 650-micron and 2600-micron particles over a pressure range of 1 to 10 atmospheres, found that Uk decreased significantly with pressure for both particle sizes (Fig. 21). They also reported that the ratio (Uk /Ut) remained relatively constant over this pressure range for both materials.

142 Fluidization, Solids Handling, and Processing
|
6 |
|
|
|
|
|
|
|
|
|
|
Material: Glass Beads |
|
|
|
|
5 |
|
|
Column: 30-cm Square |
|
||
|
|
|
Gas: Air |
|
|
|
|
, m/s |
|
|
|
|
|
|
|
4 |
|
|
|
|
|
|
|
k |
|
|
|
|
|
|
|
U |
|
|
|
|
|
|
|
Velocity, |
3 |
|
|
|
|
|
|
|
|
|
2600 microns |
|
|
||
Transition |
|
|
|
|
|
||
2 |
|
|
|
|
|
|
|
1 |
|
650 microns |
|
|
|
||
|
|
|
|
|
|
|
|
|
0 |
|
|
|
|
|
|
|
0 |
2 |
4 |
6 |
8 |
10 |
12 |
Pressure, Atmospheres
Figure 21. Effect of system pressure on Uk . (Canada & McLaughlin.)
Cai et al. (1989) also determined Uc as a function of temperature over the range 50° to 450°C. They found that Uc increased with temperature for materials belonging to Groups A, B, and D. It appears that the decrease in gas density with increasing temperature caused Uc to increase.
The boundary between the turbulent and the fast fluidization regimes has been of some dispute in the fluidization field. However, the choking velocity (Uch) appears to be a practical lower-velocity boundary for this regime (Karri and Knowlton, 1991; Takeuchi et al., 1986).

Pressure and Temperature Effects 143
Knowlton (1975), and Karri and Knowlton (1996), have also shown that the choking velocity for Group B solids decreases significantly with pressure. This is illustrated in Fig. 22 for 400-micron sand. As system pressure was increased from 1 to 31 bar, the choking velocity decreased from approximately 2.2 to 6.7 m/s for a mass flux of 210 kg/s-m2. This occurs because the carrying capacity of a gas increases as the density of the gas increases due to increased drag on the particles. This results in the pressure-drop-per-unit-length-vs.-velocity curves to be shifted to the left on the phase diagram for vertical pneumatic conveying as shown in Fig. 23.
Choking Velocity, m/s
8
Material: Sand
Size: 400 microns |
|
Riser Dia.: 7.6 cm |
|
Gas: Nitrogen |
1 Bar |
6
4
31.6 Bar
2
0
0 |
50 |
100 |
150 |
200 |
250 |
Solids Mass Flux, kg/s-m 2
Figure 22. The variation of choking velocity with pressure. (Knowlton.)
