
- •Foreword
- •Preface
- •Contents
- •About the Editors
- •Contributors
- •1: Tracheobronchial Anatomy
- •Trachea
- •Introduction
- •External Morphology
- •Internal Morphology
- •Mucous Layer
- •Blood Supply
- •Anatomo-Clinical Relationships
- •Bronchi
- •Main Bronchi
- •Bronchial Division
- •Left Main Bronchus (LMB)
- •Right Main Bronchus (RMB)
- •Blood Supply
- •References
- •2: Flexible Bronchoscopy
- •Introduction
- •History
- •Description
- •Indications and Contraindications
- •Absolute Contraindications
- •Procedure Preparation
- •Technique of FB Procedure
- •Complications of FB Procedure
- •Basic Diagnostic Procedures
- •Bronchoalveolar Lavage (BAL)
- •Transbronchial Lung Biopsy (TBLB)
- •Transbronchial Needle Aspiration (TBNA)
- •Bronchial Brushings
- •Advanced Diagnostic Bronchoscopy
- •EBUS-TBNA
- •Ultrathin Bronchoscopy
- •Transbronchial Lung Cryobiobsy (TBLC)
- •Therapeutic Procedures Via FB
- •LASER Bronchoscopy
- •Electrocautery
- •Argon Plasma Coagulation (APC)
- •Cryotherapy
- •Photodynamic Therapy
- •Airway Stent Placement
- •Endobronchial Valve Placement
- •Conclusion
- •References
- •History and Historical Perspective
- •Indications and Contraindications
- •Procedure Description
- •Procedure Planning
- •Target Approximation
- •Sampling
- •Complications
- •Future Directions
- •Summary and Recommendations
- •References
- •4: Rigid Broncoscopy
- •Innovations
- •Ancillary Equipment
- •Rigid Bronchoscopy Applications
- •Laser Bronchoscopy
- •Tracheobronchial Prosthesis
- •Transbronchial Needle Aspiration (TBNA)
- •Rigid Bronchoscope in Other Treatments for Bronchial Obstruction
- •Mechanical Debridement
- •Pediatric Rigid Bronchoscopy
- •Tracheobronchial Dilatation
- •Foreign Bodies Removal
- •Other Indications
- •Complications
- •The Procedure
- •Some Conclusions
- •References
- •History and Historical Perspective
- •Indications and Contraindications
- •Preprocedural Evaluation and Preparation
- •Physical Examination
- •Procedure-Related Indications
- •Application of the Technique
- •Topical Anesthesia
- •Anesthesia of the Nasal Mucosa and Nasopharynx
- •Anesthesia of the Mouth and Oropharynx
- •Superior Laryngeal Nerve Block
- •Recurrent Laryngeal Nerve Block (RLN)
- •Conscious Sedation
- •Monitored Anesthesia Care (MAC)
- •General Anesthesia
- •Monitoring the Depth of Anesthesia
- •Interventional Bronchoscopy Suites
- •Airway Devices
- •Laryngeal Mask Airway (LMA)
- •Endotracheal Tube (ETT)
- •Rigid Bronchoscope
- •Modes of Ventilation
- •Spontaneous Ventilation
- •Assisted Ventilation
- •Noninvasive Positive Pressure Ventilation (NIV)
- •Positive Pressure Controlled Mechanical Ventilation
- •Jet Ventilation
- •Electronic Mechanical Jet Ventilation
- •Postprocedure Care
- •Special Consideration
- •Anesthesia for Peripheral Diagnostic and Therapeutic Bronchoscopy
- •Anesthesia for Interventional Bronchoscopic Procedures During the COVID-19 Pandemic
- •Summary and Recommendations
- •Conclusion
- •References
- •Background
- •Curricular Structure and Delivery
- •What Is a Bronchoscopy Curriculum?
- •Tradition, Teaching Styles, and Beliefs
- •Using Assessment Tools to Guide the Educational Process
- •The Ethics of Teaching
- •When Learners Teach: The Journey from Novice to Mastery and Back Again
- •The Future Is Now
- •References
- •Interventional Procedure
- •Assessment of Flow–Volume Curve
- •Dyspnea
- •Analysis of Pressure–Pressure Curve
- •Conclusions
- •References
- •Introduction
- •Adaptations of the IP Department
- •Environmental Control
- •Personal Protective Equipment
- •Procedure Performance
- •Bronchoscopy in Intubated Patients
- •Other Procedures in IP Unit
- •References
- •Introduction
- •Safety
- •Patient Safety
- •Provider Safety
- •Patient Selection and Screening
- •Lung Cancer Diagnosis and Staging
- •Inpatients
- •COVID-19 Clearance
- •COVID Clearance: A Role for Bronchoscopy
- •Long COVID: A Role for Bronchoscopy
- •Preparing for the Next Pandemic
- •References
- •Historical Perspective
- •Indications and Contraindications
- •Evidence-Based Review
- •Summary and Recommendations
- •References
- •Introduction
- •Clinical Presentation
- •Diagnosis
- •Treatment
- •History and Historical Perspectives
- •Indications and Contraindications
- •Benign and Malignant Tumors
- •Tumors with Uncertain Prognosis
- •Application of the Technique
- •Evidence Based Review
- •Summary and Recommendations
- •References
- •12: Cryotherapy and Cryospray
- •Introduction
- •Historical Perspective
- •Equipment
- •Cryoadhesion
- •Indications
- •Cryorecanalization
- •Cryoadhesion and Foreign Body Removal
- •Cryoadhesion and Mucus Plugs/Blood Clot Retrieval
- •Endobronchial Cryobiopsy
- •Transbronchial Cryobiopsy for Lung Cancer
- •Safety Concerns and Contraindications
- •Cryoablation
- •Indications
- •Evidence
- •Safety Concerns and Contraindications
- •Cryospray
- •Indications
- •Evidence
- •Safety Concerns and Contraindications
- •Advantages of Cryotherapy
- •Limitations
- •Future Research Directions
- •References
- •13: Brachytherapy
- •History and Historical Perspective
- •Indications and Contraindications
- •Application of the Technique
- •Evidence-Based Review
- •Adjuvant Treatment
- •Palliative Treatment
- •Complications
- •Summary and Recommendations
- •References
- •14: Photodynamic Therapy
- •Introduction
- •Photosensitizers
- •First-Generation Photosensitizers
- •M-Tetrahidroxofenil Cloro (mTHPC) (Foscan®)
- •PDT Reaction
- •Tumor Damage Process
- •Procedure
- •Indications
- •Curative PDT Indications
- •Palliative PDT Indications
- •Contraindications
- •Rationale for Use in Early-Stage Lung Cancer
- •Rationale
- •PDT in Combination with Other Techniques for Advanced-Stage Non-small Cell Lung Cancer
- •Commentary
- •Complementary Endoscopic Methods for PDT Applications
- •New Perspectives
- •Other PDT Applications
- •Conclusions
- •References
- •15: Benign Airways Stenosis
- •Etiology
- •Congenital Tracheal Stenosis
- •Iatrogenic
- •Infectious
- •Idiopathic Tracheal Stenosis
- •Distal Bronchial Stenosis
- •Diagnosis Methods
- •Patient History
- •Imaging Techniques
- •Bronchoscopy
- •Pulmonary Function Test
- •Treatment
- •Endoscopic Treatment
- •Dilatation
- •Laser Therapy
- •Stents
- •How to Proceed
- •Stent Placement
- •Placing a Montgomery T Tube
- •The Rule of Twos for Benign Tracheal Stenosis (Fig. 15.23)
- •Surgery
- •Summary and Recommendations
- •References
- •16: Endobronchial Prostheses
- •Introduction
- •Indications
- •Extrinsic Compression
- •Intraluminal Obstruction
- •Stump Fistulas
- •Esophago-respiratory Fistulas (ERF)
- •Expiratory Central Airway Collapse
- •Physiologic Rationale for Airway Stent Insertion
- •Stent Selection Criteria
- •Stent-Related Complications
- •Granulation Tissue
- •Stent Fracture
- •Migration
- •Contraindications
- •Follow-Up and Patient Education
- •References
- •Introduction
- •Overdiagnosis
- •False Positives
- •Radiation
- •Risk of Complications
- •Lung Cancer Screening Around the World
- •Incidental Lung Nodules
- •Management of Lung Nodules
- •References
- •Introduction
- •Minimally Invasive Procedures
- •Mediastinoscopy
- •CT-Guided Transthoracic Biopsy
- •Fluoroscopy-Guided Transthoracic Biopsies
- •US-Guided Transthoracic Biopsy
- •Thoracentesis and Pleural Biopsy
- •Thoracentesis
- •Pleural Biopsy
- •Surgical or Medical Thoracoscopy
- •Image-Guided Pleural Biopsy
- •Closed Pleural Biopsy
- •Image-Guided Biopsies for Extrathoracic Metastases
- •Tissue Acquisition, Handling and Processing
- •Implications of Tissue Acquisition
- •Guideline Recommendations for Tissue Acquisition in Mediastinal Staging
- •Methods to Overcome Challenges in Tissue Acquisition and Genotyping
- •Rapid on-Site Evaluation (ROSE)
- •Sensitive Genotyping Assays
- •Liquid Biopsy
- •Summary, Recommendations and Highlights
- •References
- •History
- •Data Source and Methodology
- •Tumor Size
- •Involvement of the Main Bronchus
- •Atelectasis/Pneumonitis
- •Nodal Staging
- •Proposal for the Revision of Stage Groupings
- •Small Cell Lung Cancer (SCLC)
- •Discussion
- •Methodology
- •T Descriptors
- •N Descriptors
- •M Descriptors
- •Summary
- •References
- •Introduction
- •Historical Perspective
- •Fluoroscopy
- •Radial EBUS Mini Probe (rEBUS)
- •Ultrasound Bronchoscope (EBUS)
- •Virtual Bronchoscopy
- •Trans-Parenchymal Access
- •Cone Beam CT (CBCT)
- •Lung Vision
- •Sampling Instruments
- •Conclusions
- •References
- •History and Historical Perspective
- •Narrow Band Imaging (NBI)
- •Dual Red Imaging (DRI)
- •Endobronchial Ultrasound (EBUS)
- •Optical Coherence Tomography (OCT)
- •Indications and Contraindications
- •Confocal Laser Endomicroscopy and Endocytoscopy
- •Raman Spectrophotometry
- •Application of the Technique
- •Supplemental Technology for Diagnostic Bronchoscopy
- •Evidence-Based Review
- •Summary and Recommendations, Highlight of the Developments During the Last Three Years (2013 on)
- •References
- •Introduction
- •History and Historical Perspective
- •Endoscopic AF-OCT System
- •Preclinical Studies
- •Clinical Studies
- •Lung Cancer
- •Asthma
- •Airway and Lumen Calibration
- •Obstructive Sleep Apnea
- •Future Applications
- •Summary
- •References
- •23: Endobronchial Ultrasound
- •History and Historical Perspective
- •Equipment
- •Technique
- •Indication, Application, and Evidence
- •Convex Probe Ultrasound
- •Equipment
- •Technique
- •Indication, Application, and Evidence
- •CP-EBUS for Malignant Mediastinal or Hilar Adenopathy
- •CP-EBUS for the Staging of Non-small Cell Lung Cancer
- •CP-EBUS for Restaging NSCLC After Neoadjuvant Chemotherapy
- •Complications
- •Summary
- •References
- •Introduction
- •What Is Electromagnetic Navigation?
- •SuperDimension Navigation System (EMN-SD)
- •Computerized Tomography
- •Computer Interphase
- •The Edge Catheter: Extended Working Channel (EWC)
- •Procedural Steps
- •Planning
- •Detecting Anatomical Landmarks
- •Pathway Planning
- •Saving the Plan and Exiting
- •Registration
- •Real-Time Navigation
- •SPiN System Veran Medical Technologies (EMN-VM)
- •Procedure
- •Planning
- •Navigation
- •Biopsy
- •Complications
- •Limitations
- •Summary
- •References
- •Introduction
- •Image Acquisition
- •Hardware
- •Practical Considerations
- •Radiation Dose
- •Mobile CT Studies
- •Future Directions
- •Conclusion
- •References
- •26: Robotic Assisted Bronchoscopy
- •Historical Perspective
- •Evidence-Based Review
- •Diagnostic Yield
- •Monarch RAB
- •Ion Endoluminal Robotic System
- •Summary
- •References
- •History and Historical Perspective
- •Indications and Contraindications
- •General
- •Application of the Technique
- •Preoperative Care
- •Patient’s Position and Operative Field
- •Incision and Initial Dissection
- •Palpation
- •Biopsy
- •Control of Haemostasis and Closure
- •Postoperative Care
- •Complications
- •Technical Variants
- •Extended Cervical Mediastinoscopy
- •Mediastinoscopic Biopsy of Scalene Lymph Nodes
- •Inferior Mediastinoscopy
- •Mediastino-Thoracoscopy
- •Video-Assisted Mediastinoscopic Lymphadenectomy
- •Transcervical Extended Mediastinal Lymphadenectomy
- •Evidence-Based Review
- •Summary and Recommendations
- •References
- •Introduction
- •Case 1
- •Adrenal and Hepatic Metastases
- •Brain
- •Bone
- •Case 1 Continued
- •Biomarkers
- •Case 1 Concluded
- •Case 2
- •Chest X-Ray
- •Computerized Tomography
- •Positive Emission Tomography
- •Magnetic Resonance Imaging
- •Endobronchial Ultrasound with Transbronchial Needle Aspiration
- •Transthoracic Needle Aspiration
- •Transbronchial Needle Aspiration
- •Endoscopic Ultrasound with Needle Aspiration
- •Combined EUS-FNA and EBUS-TBNA
- •Case 2 Concluded
- •Case 3
- •Standard Cervical Mediastinoscopy
- •Extended Cervical Mediastinoscopy
- •Anterior Mediastinoscopy
- •Video-Assisted Thoracic Surgery
- •Case 3 Concluded
- •Case 4
- •Summary
- •References
- •29: Pleural Anatomy
- •Pleural Embryonic Development
- •Pleural Histology
- •Cytological Characteristics
- •Mesothelial Cells Functions
- •Pleural Space Defense Mechanism
- •Pleura Macroscopic Anatomy
- •Visceral Pleura (Pleura Visceralis or Pulmonalis)
- •Parietal Pleura (Pleura Parietalis)
- •Costal Parietal Pleura (Costalis)
- •Pleural Cavity (Cavitas Thoracis)
- •Pleural Apex or Superior Pleural Sinus [12–15]
- •Anterior Costal-Phrenic Sinus or Cardio-Phrenic Sinus
- •Posterior Costal-Phrenic Sinus
- •Cost-Diaphragmatic Sinus or Lateral Cost-Phrenic Sinus
- •Fissures18
- •Pleural Vascularization
- •Parietal Pleura Lymphatic Drainage
- •Visceral Pleura Lymphatic Drainage
- •Pleural Innervation
- •References
- •30: Chest Ultrasound
- •Introduction
- •The Technique
- •The Normal Thorax
- •Chest Wall Pathology
- •Pleural Pathology
- •Pleural Thickening
- •Pneumothorax
- •Pulmonary Pathology
- •Extrathoracic Lymph Nodes
- •COVID and Chest Ultrasound
- •Conclusions
- •References
- •Introduction
- •History of Chest Tubes
- •Overview of Chest Tubes
- •Contraindications for Chest Tube Placement
- •Chest Tube Procedural Technique
- •Special Considerations
- •Pneumothorax
- •Empyema
- •Hemothorax
- •Chest Tube Size Considerations
- •Pleural Drainage Systems
- •History of and Introduction to Indwelling Pleural Catheters
- •Indications and Contraindications for IPC Placement
- •Special Considerations
- •Non-expandable Lung
- •Chylothorax
- •Pleurodesis
- •Follow-Up and IPC Removal
- •IPC-Related Complications and Management
- •Competency and Training
- •Summary
- •References
- •32: Empyema Thoracis
- •Historical Perspectives
- •Incidence
- •Epidemiology
- •Pathogenesis
- •Clinical Presentation
- •Radiologic Evaluation
- •Biochemical Analysis
- •Microbiology
- •Non-operative Management
- •Prognostication
- •Surgical Management
- •Survivorship
- •Summary and Recommendations
- •References
- •Evaluation
- •Initial Intervention
- •Pleural Interventions for Recurrent Symptomatic MPE
- •Especial Circumstances
- •References
- •34: Medical Thoracoscopy
- •Introduction
- •Diagnostic Indications for Medical Thoracoscopy
- •Lung Cancer
- •Mesothelioma
- •Other Tumors
- •Tuberculosis
- •Therapeutic Indications
- •Pleurodesis of Pneumothorax
- •Thoracoscopic Drainage
- •Drug Delivery
- •Procedural Safety and Contraindications
- •Equipment
- •Procedure
- •Pre-procedural Preparations and Considerations
- •Procedural Technique [32]
- •Medical Thoracoscopy Versus VATS
- •Conclusion
- •References
- •Historical Perspective
- •Indications and Contraindications
- •Evidence-Based Review
- •Endobronchial Valves
- •Airway Bypass Tracts
- •Coils
- •Other Methods of ELVR
- •Summary and Recommendations
- •References
- •36: Bronchial Thermoplasty
- •Introduction
- •Mechanism of Action
- •Trials
- •Long Term: Ten-Year Study
- •Patient Selection
- •Bronchial Thermoplasty Procedure
- •Equipment
- •Pre-procedure
- •Bronchoscopy
- •Post-procedure
- •Conclusion
- •References
- •Introduction
- •Bronchoalveolar Lavage (BAL)
- •Technical Aspects of BAL Procedure
- •ILD Cell Patterns and Diagnosis from BAL
- •Technical Advises for Conventional TLB and TLB-C in ILD
- •Future Directions
- •References
- •Introduction
- •The Pediatric Airway
- •Advanced Diagnostic Procedures
- •Endobronchial Ultrasound
- •Virtual Navigational Bronchoscopy
- •Cryobiopsy
- •Therapeutic Procedures
- •Dilation Procedures
- •Thermal Techniques
- •Mechanical Debridement
- •Endobronchial Airway Stents
- •Metallic Stents
- •Silastic Stents
- •Novel Stents
- •Endobronchial Valves
- •Bronchial Thermoplasty
- •Discussion
- •References
- •Introduction
- •Etiology
- •Congenital ADF
- •Malignant ADF
- •Cancer Treatment-Related ADF
- •Benign ADF
- •Iatrogenic ADF
- •Diagnosis
- •Treatment Options
- •Endoscopic Techniques
- •Stents
- •Clinical Results
- •Stent Complications
- •Other Available Stents
- •Other Endoscopic Methods
- •References
- •Introduction
- •Anatomy and Physiology of Swallowing
- •Functional Physiology of Swallowing
- •Epidemiology and Risk Factors
- •Types of Foreign Bodies
- •Organic
- •Inorganic
- •Mineral
- •Miscellaneous
- •Clinical Presentation
- •Acute FB
- •Retained FB
- •Radiologic Findings
- •Bronchoscopy
- •Airway Management
- •Rigid Vs. Flexible Bronchoscopy
- •Retrieval Procedure
- •Instruments
- •Grasping Forceps
- •Baskets
- •Balloons
- •Suction Instruments
- •Ablative Therapies
- •Cryotherapy
- •Laser Therapy
- •Electrocautery and APC
- •Surgical Management
- •Complications
- •Bleeding and Hemoptysis
- •Distal Airway Impaction
- •Iron Pill Aspiration
- •Follow-Up and Sequelae
- •Conclusion
- •References
- •Vascular Origin of Hemoptysis
- •History and Historical Perspective
- •Diagnostic Bronchoscopy
- •Therapeutic Bronchoscopy
- •General Measures
- •Therapeutic Bronchoscopy
- •Evidence-Based Review
- •Summary
- •Recommendations
- •References
- •History
- •“The Glottiscope” (1807)
- •“The Esophagoscope” (1895)
- •The Rigid Bronchoscope (1897–)
- •The Flexible Bronchoscope (1968–)
- •Transbronchial Lung Biopsy (1972) (Fig. 42.7)
- •Laser Therapy (1981–)
- •Endobronchial Stents (1990–)
- •Electromagnetic Navigation (2003–)
- •Bronchial Thermoplasty (2006–)
- •Endobronchial Microwave Therapy (2004–)
- •American Association for Bronchology and Interventional Pulmonology (AABIP) and Journal of Bronchology and Interventional Pulmonology (JOBIP) (1992–)
- •References
- •Index
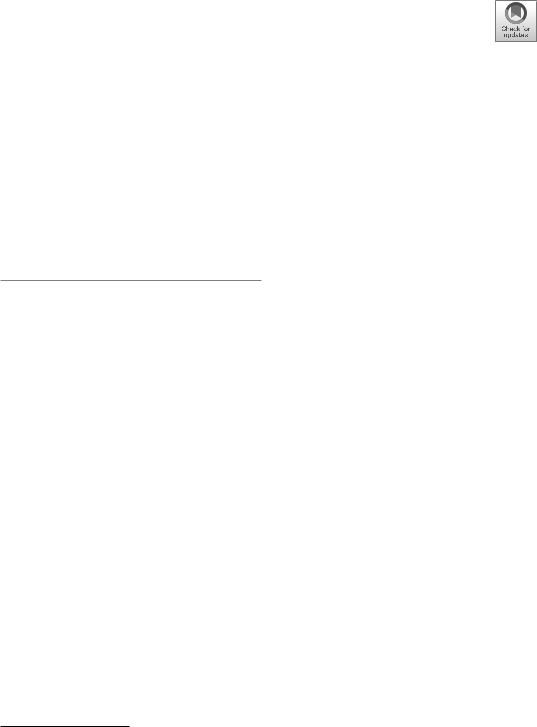
Robotic Assisted Bronchoscopy |
26 |
|
|
Tarek Dammad and Bilal A. Jalil |
|
Historical Perspective
The word robot comes from the Czech word “robota” meaning laborer. In 1921, the term robot was rst introduced by Karel Capek in his play Rossom’s Universal Robots. He described the creation and evolution of robots and eventually their revolt against humans. In 1942, writer Isaac Asimov de ned the three rules of robotics in his science ction books “Runaround” and “I, Robot”: robots must not harm humans, must follow instructions, and protect their existence [1].
Before the term “robot” came to life, autonomously operated machines could be dated to 400 BC when Archytas developed a steam- powered, self-propelling wooden pigeon capable of fying 200 meters [2]. However, Leonardo da Vinci in 1495 designed the “Metal-Plated Warrior”, the rst robot that imitated human movements of the jaw, arms, and neck (Fig. 26.1). This invention inspired Gianello Torriano, who created a robotic mandolin-play- ing lady in 1540 [1].
It was not until 1985 that robotics entered theeld of medicine. Robot-assisted surgeries have
T. Dammad (*)
AdventHealth Orlando, Orlando, FL, USA
Houston Methodist, Houston, TX, USA
B. A. Jalil
Heart and Vascular Institute, West Virginia University,
Morgantown, WV, USA
been an ongoing development in the last ve decades. The rst robot to be used in surgical procedures was the PUMA 560, introduced in 1985 and assisted in performing computed tomography (CT)-guided biopsies of the brain. Almost a decade after the introduction of the PUMA 560, an endoscopic surgical robot called theAESOP (Automated Endoscopic System for Optimal Positioning) was developed. A decade after introducing the AESOP, Intuitive Surgical, in 1997, introduced the Da Vinci Surgery System for laparoscopic surgery, where the rst laparoscopic cholecystectomy was performed in Belgium [3]. The Da Vinci system has seen an immense expansion in its utility from general surgery, gynecologic surgery, and cardiac and thoracic surgical procedures.
The limitations in conventional bronchoscopy led the path of innovations over the years to attempt tissue sampling in peripheral airways, beyond the visualization of a bronchoscope. One of these innovations is electromagnetic navigational bronchoscopy (ENB), which is the most common modality used to approach peripheral nodules that are beyond the visualization of the subsegmental anatomy and relies on using a combination of a virtual map of the tracheobronchial tree generated from reconstructed CT images and electromagnetically-mapped images to navigate more peripheral airways and lesions. The other modality is radial-endobronchial ultrasound (R-EBUS) which allows for sonographic visualization of peripheral lung lesions combined with
© The Author(s), under exclusive license to Springer Nature Switzerland AG 2023 |
453 |
J. P. Díaz-Jiménez, A. N. Rodríguez (eds.), Interventions in Pulmonary Medicine, https://doi.org/10.1007/978-3-031-22610-6_26
Данная книга находится в списке для перевода на русский язык сайта https://meduniver.com/
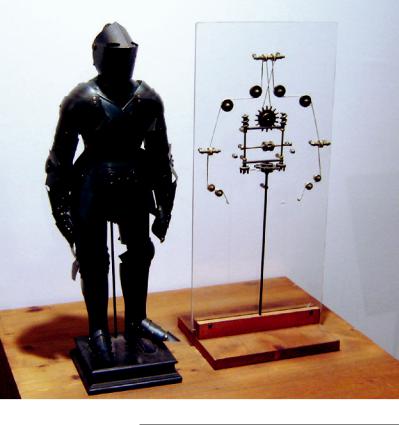
454 |
T. Dammad and B. A. Jalil |
|
|
Fig. 26.1 The “Metal-Plated Warrior” designed by Leonardo da Vinci in 1495, therst robot that imitated human movements of the jaw, arms, and neck
guidance sheath, with or without fuoroscopy or ENB. Although prior data suggested diagnostic yields as high as 70%, more recent data suggests the diagnostic yield to be inconsistent and closer to 40–60% [4–6]. There are a multitude of technical concerns with the stability and extension beyond the bronchoscope during sampling of peripheral lung tissue, which may explain the lower diagnostic yield. Robotic-assisted bronchoscopy (RAB) is an attempt to improve on the problems experienced during ENB.
The rst robotic-assisted bronchoscopy (RAB) platform is the Monarch (Auris Health), utilizing electromagnetic (EM) Guidance that attained Food and Drug Administration (FDA) approval in March 2018. In February 2019, the FDA approved a second RAB platform, the Ion Robotic Endoluminal platform (Intuitive Surgical), utilizing Shape Sensing Technology. Both platforms comprise similar equipment, including a bronchoscope, a robotic interface, and a controller; however, they have a few operational differences.
Description andDesign
The Monarch Platform (Auris Health,
Inc. Redwood City, CA)
The Monarch RAB platform (Fig. 26.2) consists of four main components: The bronchoscope, cart (robotic arms), tower, and the electromagnetic generator.
The bronchoscope (Fig. 26.3a–c) consists of an inner bronchoscope with an outside diameter of 4.2 mm, a working channel of 2.1 mm, a camera, and an integrated light source that enable direct visualization during the procedure. The outer sheath has a 6 mm outer diameter.
The inner bronchoscope is made to telescope through the outer sheath, and its movement can be coupled or uncoupled when advanced in the bronchial tree. Usually, their movement is un- coupled past the three-fourth generation of the bronchial tree. The outer sheath and inner bronchoscope both offer four-way steering control
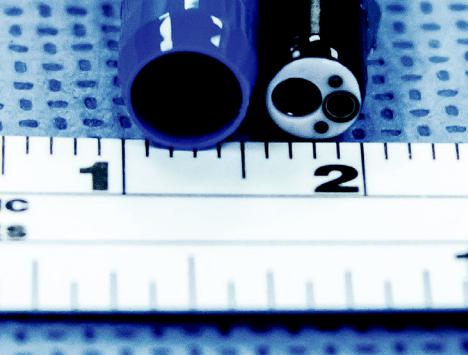
26 Robotic Assisted Bronchoscopy |
455 |
|
|
Fig. 26.2 The Monarch RAB platform with its 4 main components: the bronchoscope, cart (robotic arms), tower, and the electromagnetic generator
and articulate up to 180 degrees. This con guration enhances the stability of the bronchoscope and maneuverability of the scope to access lesions further into the lung. The proximal end of the bronchoscope (Fig. 26.3d) is equipped with a valve that accesses the 2.1 mm working channel and enables irrigation, suction, and the insertion of various ancillary tools, such as radial ultrasound probe, needle, brush, or biopsy forceps.
The cart (Fig. 26.4) comprises two robotic arms with rotary pulleys (Fig. 26.5) that connect to the bronchoscope cables and exert proper tension to drive the inner bronchoscope and the outer sheath in coupled or uncoupled modes. The bronchoscopist uses a video game-type controller to move the robotic arms to drive and navigate the bronchoscope. The cart contains the electronic systems required to operate the platform and adjust its height and level the robotic arms and the bronchoscope with the entry point of the outer tip of the endotracheal tube.
The tower (Fig. 26.6) connects to the bronchoscope, controller, and the electromagnetic gener-
ator. The controller (Fig. 26.7), with its two joysticks and other control buttons, is used to drive, articulate the bronchoscope, and navigate the screen of the tower to reach the target lesion. The tower has two computers that operate the system, a non-real-time computer and a real-time computer that communicate with each other during the procedure. The non-real-time computer receives input from the pendant, keyboard, mouse, camera, electromagnetic localizer, and power distribution unit. It also contains an interface to the camera at the bronchoscope’s tip that performs the necessary image processing and generates video output streams. On the other hand, the real-time computer receives inputs from the non-real-time computer. Real-time video captured from the bronchoscope’s tip and overlaid with other information from the robotic system is displayed on the tower monitor.
The electromagnetic eld generator is placed close to the patient’s chest with the attached reference electromagnetic sensors, and it is an essential tool for navigation guidance [7].
Данная книга находится в списке для перевода на русский язык сайта https://meduniver.com/

456 |
T. Dammad and B. A. Jalil |
|
|
|
|
Fig. 26.3 (a) The |
a |
|
Monarch Bronchoscope. |
||
|
||
Photograph by Leslie |
|
|
Kumpf RRT, CPFT. (b) |
|
|
The Monarch |
|
|
Bronchoscope in |
|
|
articulation. Photograph |
|
|
by Leslie Kumpf RRT, |
|
|
CPFT. (c) The inner |
|
|
bronchoscope of the |
|
|
Monarch platform with |
|
|
the bronchoscope |
|
|
extending beyond the |
|
|
outer sheath. Photograph |
|
|
by Leslie Kumpf RRT, |
|
|
CPFT |
|
b |
c |
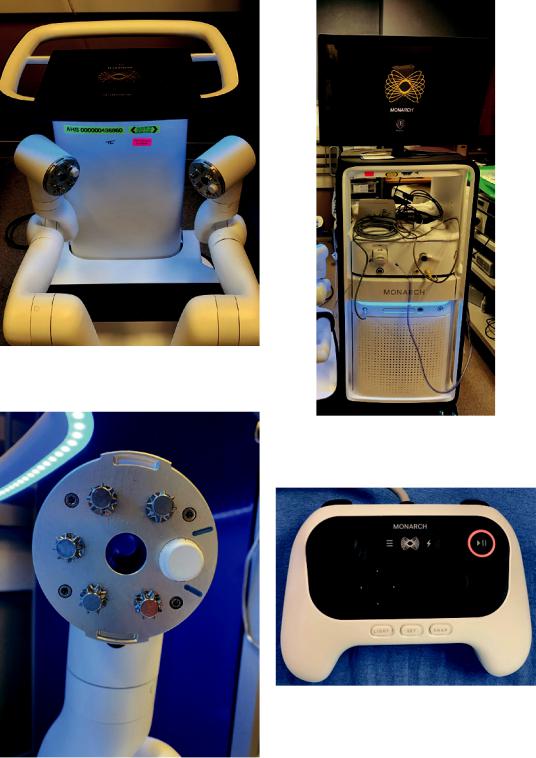
26 Robotic Assisted Bronchoscopy |
457 |
|
|
Fig. 26.4 The robotic arms of the Monarch platform
Fig. 26.6 The bronchoscope tower of the Monarch platform
Fig. 26.7 The video-game based controller of the
Monarch platform
Fig. 26.5 A close-up view of the robotic arm of the
Monarch platform
Данная книга находится в списке для перевода на русский язык сайта https://meduniver.com/
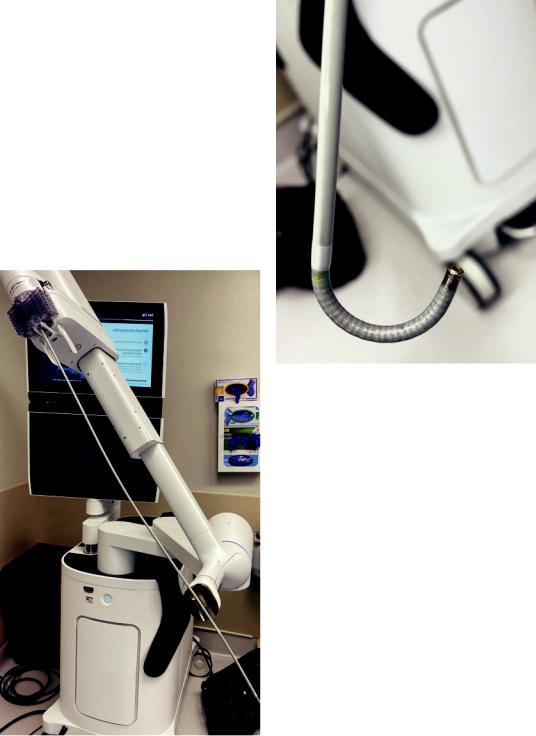
458 |
T. Dammad and B. A. Jalil |
|
|
The Ion Robotic Endoluminal System
(Intuitive Surgical, Sunnyvale,
CA, USA)
The Ion Endoluminal System uses shape-sensing technology rather than electromagnetic navigation. It consists of three main components: the fexible robotic catheter (bronchoscope), the robotic cart with display screens, and a controller (Fig. 26.8).
The fexible robotic catheter (bronchoscope) has an outer diameter 3.5 mm and a working channel of 2.0 mm. Within the catheter sits the removable vision probe. The robotic catheter articulates 180 degrees in all directions
|
Fig. 26.9 The shape-sensing |
catheter of the Ion |
|
platform |
|
|
(Fig. 26.9). It contains |
shape-sensing bers |
|
through its entire length that communicates in |
|
|
real-time to the robotic software and system, |
|
|
enabling positional and shape feedback, precise |
|
|
location of the bronchoscope, and its distance to |
|
|
the target and nearest pleural surface, all to ensure |
|
|
smooth maneuverability and precise reach and |
|
|
sampling of the target lesion. The robot cart has |
|
|
display screens that can project the broncho- |
|
|
scopic and radial ultrasound views. Finally, there |
|
|
is a controller cart with a trackball and scroll |
|
|
wheel. |
|
|
Once the target lesion is reached or |
|
|
approached, the robotic catheter/bronchoscope |
|
|
is locked in position, and the vision probe is |
|
|
removed to enable insertion of diagnostic tools |
|
|
like radial US probe, needles, forceps, or brush. |
|
|
The bronchoscopist receives real-time feedback |
|
|
through the displayed catheter shape, con rm- |
|
Fig. 26.8 The Ion platform with its components |
ing non-slippage. |
|
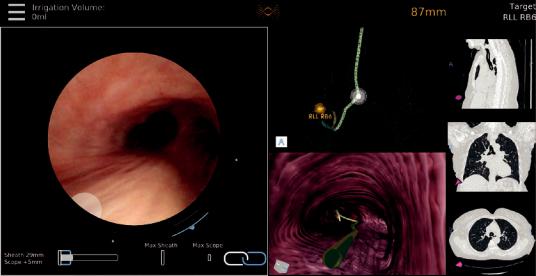
26 Robotic Assisted Bronchoscopy |
459 |
|
|
Procedure andTechnique
Pre-procedure planning is essential for the success of the procedure. It starts with thin-cut chest computed tomography images with a slice thickness of 0.5–1 mm and slice interval of 0.5–0.8 mm.
Three-dimensional virtual lung reconstruction is generated via the software. The target lesion is marked and sized. Then, the robotic system software generates and maps the pathway. Manual planning or segmentation of the airway is possible, especially in the absence of a bronchus sign. The computed tomography scan should preferably be done within 27 days of the procedure.
Metallic objects should be removed from the immediate EM eld during the setup, registration, and electromagnetic navigation part of the procedure in the case of the Monarch Robotic System to prevent signal interference. There is no need to this when using the Ion Endoluminal Robotic system.
After consent is obtained and time-out is done, the patient undergoes general anesthesia by the anesthesia team. Paralytics are not mandatory but deep sedation is important. Patient is usually intubated with an endotracheal tube no smaller than 7.5 mm. A tidal volume of 8 mL/kg of predicted body weight and positive end expiratory pressure
(PEEP) of 8 to 10 cmH2O is recommended to prevent atelectasis in the anesthetized patients.
Conventional fexible bronchoscopy is rst done with a fexible bronchoscope to inspect the tracheobronchial tree thoroughly and identify other abnormalities in addition to clearing secretions. Once completed, the fexible bronchoscope is removed and the robotic bronchoscope is advanced to the patient’s airways. Next, registration is done to couple virtual anatomy with the patient’s real-time airway anatomy. The bronchoscopist thereafter navigates to the target lesion.
In the case of the Monarch Robotic Bronchoscope, the outer sheath and the inner bronchoscope are advanced in coupled mode. Once the segmental airway is reached or around the 3-4th bronchial generation, the outer sheath is locked in place, and the inner bronchoscope is uncoupled and advanced further to navigate to the target lesion. The Monarch platform preserves real-time white light vision, while ancillary tools are utilized at the target lesion (Fig. 26.10).
On the other hand, the robotic catheter of the Ion Endoluminal System with the bronchoscope will reach the target lesion, and it will be locked in position; at that point, the vision probe must be removed
Fig. 26.10 The view screen of the Monarch platform with a live view, virtual map, and CT images
Данная книга находится в списке для перевода на русский язык сайта https://meduniver.com/