
Учебники / Computer-Aided Otorhinolaryngology-Head and Neck Surgery Citardi 2002
.pdfPrinciples of Registration |
71 |
is usually incorrect selection of the point in the preoperative imaging data set and/or in the operating field volume. Both of these are user errors.
User errors rarely influence the accuracy of automatic registration, since the registration process simply localizes the fiducial markers on the relocatable headset in the preoperative imaging data set. As a practical matter, user error can compromise the next step in these systems. If the relocatable headset is not correctly placed on the patient, then differences between the intraoperative placement and the placement used at the time of preoperative imaging leads to obvious errors. For this reason, radiology personnel and operating room personnel (including the surgeon) must be familiar with the relocatable headset.
Registration protocols are designed to support surgical navigation. If registration fails, then surgical navigation will be impossible. In some cases, registration may seem successful, but the surgical navigation accuracy will be poor. For this reason, surgeons must continually check the fidelity of surgical navigation by localizing against known anatomical landmarks in the operating field volume throughout the case. When surgical navigation accuracy is compromised, the cause usually can be tracked to one of the following problems:
1.The initial registration was not as robust as it initially appeared.
2.The DRF has shifted relative to the operating field volume.
3.The CAS pointer is damaged, or the CAS pointer is no longer calibrated due to even a slight shift in the alignment of the tip relative to the ILD.
4.The tracking system has failed. The actual hardware can fail. Transient errors are more common. If the line of sight between the ILDs and the camera array is not maintained for optical systems, then the system cannot track. Similarly, distortions of the electromagnetic field can disrupt tracking based on electromagnetic sensors.
5.The CAS software can freeze/crash. These bugs can be obvious, but they can be more subtle. If the latter is true, then detection of this error can be much more difficult.
4.7CONCLUSION
Registration refers to the process through which corresponding fiducial points in the preoperative imaging data set volume and the intraoperative operating field volume are aligned. As a result, registration serves as the foundation for surgical navigation. Paradigms for registration may be divided into the categories of manual point mapping, automatic registration (with a relocatable headset), and contour mapping. Each of these approaches offers specific advantages and disadvantages. Because of the complexity of registration and its impact on clinical applications, surgeons should be familiar with registration principles. This will serve to enhance the overall clinical effectiveness of CAS.

5
Intraoperative Magnetic Resonance
Imaging
Neil Bhattacharyya, M.D., F.A.C.S.,
and Liangge Hsu, M.D.
Brigham and Women’s Hospital, Harvard Medical School,
Boston, Massachusetts
Marvin P. Fried, M.D.
Albert Einstein College of Medicine and Montefiore Medical Center, Bronx, New York
5.1 INTRODUCTION
The surgical anatomy of the head and neck is replete with a higher density of vascular, nervous, and specialized soft tissue structures than virtually any other portion of the human body. Surgery within the head and neck therefore confronts the otolaryngologist with a complex array of interrelated three-dimensional structures that govern the approach, safety, and efficacy for operative procedures within the head and neck. The otolaryngologist is often faced with balancing complete extirpation of the lesion, preservation of complex functions, avoidance of vitally important surrounding structures, and cosmesis when considering surgery within the head and neck.
The advent of multiplanar imaging technology has had a profound impact
73
74 |
Bhattacharyya et al. |
on the diagnosis and surgical treatment of otolaryngologic disease. Computed tomography (CT) has virtually supplanted plain film radiography in the diagnosis of chronic rhinosinusitis, chronic otitis media, and upper airway anatomy [1]. It is now widely employed in the staging of head and neck tumors, both at the primary site and as a screening tool for nodal metastases to the neck. CT is also extremely valuable in the preoperative anatomical planning for endoscopic sinus surgery and extirpative procedures for head and neck neoplasia [2]. CT derives its utility mainly from its highly accurate delineation of bony and soft tissue detail, separating the morphology of pathological processes from normal anatomy. More recently, magnetic resonance imaging (MRI) has seen wider utilization in the diagnosis of otolaryngologic disease. It is now considered the gold standard test for the diagnosis of acoustic neuroma and neoplastic conditions of the skull base. While both of these modalities provide excellent spatial resolution, MRI has the advantages of imaging in multiple planes and better delineation of soft tissue detail [3].
Until recently, both CT and MRI have been primarily employed in the preoperative diagnosis and surgical planning for lesions within the head and neck; real-time or intraoperative use of these modalities has been quite limited. Several groups have explored CT for localization and needle biopsy of head and neck tumors [4]. CT has shown promise in adding to the accuracy of fine needle biopsy for nodal staging of head and neck tumors [5]. Even more recently, intraoperative MRI has been explored as a surgical adjunct for biopsy and operative treatment of head and neck lesions [3,6]. The advent of new technology has largely been responsible for these contemporary advances.
Intraoperative multiplanar imaging may present several advantages for surgery within the head and neck. First, as a navigational tool, it may assist the surgeon in avoiding important surrounding structures while performing the surgical procedure within the intended anatomy. In addition, it may provide real-time confirmation that the intended lesion has been biopsied, removed, or appropriately modified by the procedure [7]. These potential advantages have driven current investigators to pursue intraoperative or interventional magnetic resonance imaging (I-MRI) and to develop additional technologies to provide such information intraoperatively.
5.2 DESCRIPTION OF THE TECHNOLOGY
5.2.1 Description of the Open MRI Unit
While several interventional MRI units are under construction or in early use, only a few have been employed with published data for head and neck purposes. We describe here in the prototype open MR interventional suite currently in use
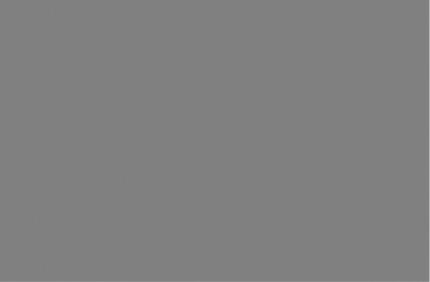
Intraoperative Magnetic Resonance Imaging |
75 |
at the Brigham and Women’s Hospital and Harvard Medical School [8]. This magnet has been operational since January 1994.
The prototype open MRI magnet is housed in a 3500 square foot suite within a 25 25 feet procedure room (Figure 5.1). The design was implemented by General Electric in 1987 in collaboration with the Brigham and Women’s Hospital, Boston, and installed in January 1994. The magnet itself is a 0.5 Tesla superconducting magnet constructed for the sole purpose of providing image guidance for minimally invasive surgical procedures. The unit itself has an ‘‘open’’ configuration, with six superconducting coils arranged to accommodate a split cryostat, allowing a 56 cm wide vertical gap. This vertical gap allows the surgeon ‘‘bedside’’ access to the patient, similar to that provided in the conventional operating suite configuration. This essential vertical gap for access to the patient is achieved by modifying the two-coil Helmholtz design, and altering the superconducting material by using niobium-tin with the slightly higher transition temperature of 18.1 K (versus niobium-titanium with a transition temperature of 10.1 K). This small difference in temperature permits the coils to operate at a higher temperature for which a two-stage cryocooler assembly is adequate, thus obviating the need for the liquid helium bath. The absence of this liquid helium bath permits an increase space between the superconducting coils, thus allowing for the vertical gap [9]. Three pairs of superconducting coils are housed in a
FIGURE 5.1 Open magnet interventional magnetic resonance imaging unit.
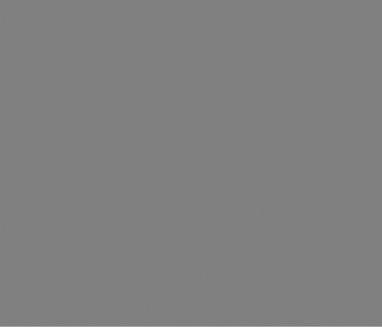
76 |
Bhattacharyya et al. |
separate but communicating cryostat, which provides a homogeneous spherical imaging volume approximately 30 cm in diameter. Surface flexible transmission and receiving radio frequency coils are placed over the area of interest for image acquisition. The system is also designed to allow differing patient positions, including the sitting position, as well as allowing the operate surgeon to be positioned sitting or standing at either the patient’s head or side (Figure 5.2). A highquality two-way audio system provides communication between the operating surgeon and radiology personnel during the procedure (Figure 5.3).
In addition to the magnet, the operating suite is specially designed to merge features of a radiology suite with the essential features of a traditional operating room. In order to do so, multiple special innovations were conceived to allow the introduction of many of the basic instrumentation, anesthesia, and monitoring devices into and around the magnetic field. The relevant concerns include safety of both the patient and health-care personnel and the compatibility of instruments, monitoring devices, and anesthesia delivery, as well as proper functioning of various image display and integration systems.
FIGURE 5.2 Open magnet interventional magnetic resonance imaging unit. The surgeon’s access to the operative field is demonstrated.
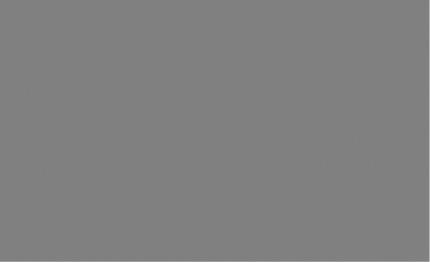
Intraoperative Magnetic Resonance Imaging |
77 |
FIGURE 5.3 Interventional MR console. The radiologists and MR technicians control the I-MRI unit through this interface. Direct interaction with the surgical team influences the progress of the surgical procedure.
5.2.2 Anesthesia Considerations
All of the anesthesia equipment employed in the I-MRI suite must be magnetic resonance (MR) compatible. The anesthesia workstation (XL10 MRI Compatible Anesthesia Station: Ohmeda, Madison, WI), ventilator (Omnivent, Topeka, KS), and monitoring equipment systems, including pulse oximetry, electrocardiogram, automated noninvasive blood pressure and invasive pressure transducer, were specially designed to be MRI compatible. Most of the other anesthesia equipment such as intravenous lines, needles, and syringes are standard, and no modifications were required. Other items meeting modification included aluminum oxygen tanks and IV poles as well as nonmagnetic lithium batteries. Unfortunately, MRI-compatible infusion pumps and intubating devices are not currently available. This requires that patients be intubated outside the operating enclosure, followed by subsequent transfer into the I-MRI procedure room [8].
One of the major concerns for patient safety involves the inability to perform a standard 12-lead EKG within the procedure room because of the ST wave distortion caused by the magnetic field. A limited monitoring EKG system was developed with EKG leads composed of carbon-fiber or fiber optics to provide intraoperative cardiac monitoring. In the event of a potential intraoperative cardiopulmonary event, the patient would be brought outside the procedure room
78 |
Bhattacharyya et al. |
immediately. The magnetic field may then be ‘‘quenched’’ within 70 seconds and cardiac defibrillation performed; cardiac defibrillation itself is not MRI compatible.
5.2.3 Surgical Instrumentation
As most standard surgical instrumentation is made of stainless steel or other ferromagnetic products, special equipment must be designed that is MR compatible. This is especially important in otolaryngology, where multiple sets of specialized instruments are required for various procedures. Special equipment has been designed in conjunction with surgical instrument manufacturers to be used in the I-MRI suite [8]. Most are made from material such as low iron content stainless steel, copper, or titanium. Surgical instruments made from brass, ceramic, and carbon fiber have an additional advantage in that they demonstrate the least imaging artifact on intraoperative MRI scans. For procedures such as endoscopic sinus surgery (ESS), additional special instrumentation was required including endoscopes, forceps, and probes (Storz Surgical Instruments, St. Louis, MO) [10]. High-speed pneumatic drills (Midas Rex, Fort Worth, TX) are similar to conventional drills except that the drill bearings are made of ceramic materials.
5.2.4 Image-Guidance Methodologies
5.2.4.1 Optical Tracking System
Image guidance during the surgical procedure is provided by near real-time MR imaging via a three-dimensional optical tracking system that utilizes two infrared light-emitting diodes (LED) mounted on a handpiece or incorporated into various surgical instruments. Three high-resolution digital cameras mounted overhead within the magnet track the position of the handpiece, in an arrangement that is similar to other optical tracking devices. Spatial position and three-dimensional coordinates are then calculated and MR images in three orthogonal planes are generated on the MR images, providing image localization. A 5-inch square liquid crystal display monitor, which is mounted within the vertical gap, displays a crosshair on any one of three orthogonal MR images, thus providing the surgeon with image localization of the surgical instrument [11]. Other investigators have also developed nonoptical image tracking techniques that can be localized via real-time MR scanning [12]. Such systems have the advantage of not being subject to optical signal loss, which can occur in a confined imaging volume due to spatial limitations.
5.2.4.2 Real-Time MRI During Surgery
In addition to the optical tracking system, the I-MRI unit is capable of real-time MRI scans of the operative field during the surgical procedure. A methodology
Intraoperative Magnetic Resonance Imaging |
79 |
known as ‘‘interactive scan plane control’’ has been developed to allow interactive scanning with the plane to be scanned determined by the operating surgeon. The scan plane is determined by the surgeon at discrete times during the procedure by using a single-purpose pointer brought into the surgical field [8]. The position of this pointer is recognized by a detection system utilizing infrared LED that are mounted above the vertical operating gap within the magnet. The position of the LED is then calculated by computer algorithm providing coordinates in the sagittal, axial, and coronal planes. The pointing device is then visualized by the surgeon as a small crosshair on the MRI console and the liquid crystal display, and the position of the pointing device is verified. The main purpose of the pointing device is to dictate the three-dimensional planes to be imaged by the realtime scan. Once the desired position has been confirmed, a real-time MR scan may be obtained, and multiplanar MR images centered on the desired position in the surgical field can be visualized. This allows active verification of surgical position within the operating field and shows changes in anatomy produced by the procedure itself [8].
Static pointing devices have also been developed for use in surgical localization. Such pointers can be constructed of gadolinium-filled or labeled tubes and instruments. When real-time scans are conducted with these instruments in the surgical field, these pointers appear as high-intensity objects on T1-weighted MR images. This type of pointing device is non interactive and does not dictate the plane to be imaged, but rather serves to verify surgical position, even when anatomy has changed due to the surgical procedure.
5.2.4.3 Acquisition Times
With the current technology, images may be acquired at discrete intervals during surgery with a mean acquisition time of 14 seconds using a ‘‘fast spin echo’’ sequence, with a relaxation time (TR) of 400 msec and an excitation time (TE) 26 msec. This acquisition time compares quite favorably to conventional spin echo sequences, which require minutes for acquisition. Even faster acquisition times of images during the procedure can be achieved with ‘‘fast gradient sequences.’’ However, though these images only require 1–2 sec to compose, these sequences provide less signal-to-noise quality, and thus provide significantly less anatomical resolution. They have been found to afford good intraoperative instrument localization.
5.2.5 Training of Personnel
Accompanying the development of any innovative technology is a learning curve required to employ the technology effectively. This is even truer in the I-MRI suite because of the complex interactions between the operating surgeon, radiology personnel, and anesthesia personnel. Prior to utilizing the I-MRI suite in
80 |
Bhattacharyya et al. |
clinical trials, extensive training was conducted with cadavers [8]. Such training was conducted not only to familiarize operating surgeons with the spatial limitations of the open magnet system, but also to verify the MR compatibility of the surgical instrumentation. In addition, studies were conducted to verify the accuracy and precision of the optical tracking system and the intraoperative MR images. The three-dimensional optical tracking system has been found to be linear to within 1% through the 30 cm imaging volume. The tracking accuracy of the instrument within the imaging volume exhibits a 1 mm tolerance. During training, it was evident that one problem with the optical tracking system is that it is extremely sensitive to changes in instrumentation angle, which may obscure optical tracking of the LED. Such considerations must be carried over into clinical preparations and planning.
In addition to the surgeon’s operative training, extensive training for support staff and personnel is required to orient them to the unique safety and efficiency considerations required by the open magnet system. A special team of anesthesia personnel familiar with the I-MRI and its limitations was assembled and trained. Similar considerations were employed in assembling the nursing staff for head and neck I-MRI procedures. Patients and operating room personnel with cardiac pacemakers or aneurysm clips that have not been verified as MR compatible must be excluded from the operating suite. Special protocols for emergencies, equipment failure, and unforeseen circumstances must be constructed and implemented in order to ensure proper patient safety [8].
5.3 APPLICATIONS
5.3.1MRI-Guided Biopsy of Head and Neck Lesions
Fine needle aspiration biopsy (FNAB) is now commonplace in the diagnosis of head and neck lesions. It may be used to provide an initial cytological diagnosis for primary lesions arising in major salivary glands, submucosal lesions in the upper aerodigestive tract, and cervical lymphadenopathy. It has been shown to be highly sensitive, specific, and reliable [13,14].
Extensive work has been done with coupling FNAB to various imaging modalities. Such a linkage offers the attractive benefit of ensuring that the desired tissue is actually sampled. Increasing accuracy for FNAB has been demonstrated when ultrasound or CT guidance is used to direct and confirm the biopsy [15,16]. Sensitivity and specificity may be increased to up to 95% with these adjunctive techniques. Image-guided biopsy techniques are essential when the lesion is not palpable, situated within the deeper structures of the head and neck, or when vital structures lie between the scan and the lesion of interest [16].
MR-guided FNAB may provide distinct advantages over other image-

Intraoperative Magnetic Resonance Imaging |
81 |
guided biopsy techniques. First, with the capacity to image in multiple planes, three-dimensional confirmation that the target tissue has been sampled may be realized. Second, of the major imaging techniques, MRI provides the best resolution and distinctions of the various soft tissues within the head and neck [17]. Since in many cases it is desirable to follow the biopsy needle from its entry point continuously until it reaches the target tissue, multiple scans are often required. In this situation, iterative CT scans will result in additional radiation exposure to the patient (and health-care personnel), and such CT scans may require administration of intravenous contrast with potential attendant side effects. Although ultrasound imparts no radiation to the patient, it is less efficient at delineating soft tissue/soft tissue interfaces than MRI. Because of its favorable imaging characteristics (Table 5.1) and the lack of radiation exposure, MR-guided biopsy in the head and neck has recently been explored [3,6].
Fried and associates [3] reported on their initial patient experience with MR-guided needle biopsies within the head and neck. In their series of seven patients, none of the lesions requiring biopsy could be palpated or visualized on routine examination. Target lesions included four parotid gland lesions, two tumors of the parapharyngeal space, and one lesion of the second cervical vertebra. Using intraoperative fast-gradient echo pulse sequences, with 2–4 sec acquisition times, interactive MR-guided biopsies were performed. The authors were able to successfully localize the desired tissue for biopsy using both the previously described optical tracking system as well as sequential intraoperative scanning. Notably, the authors found that the needle trajectory required alteration several times based on the intraoperative images. In five of seven cases the MR-guided
TABLE 5.1 Summary of Important Distinctions Between Potential Intraoperative Imaging Modalities
|
|
Intraoperative imaging modality |
|
|
|
|
|
|
|
Computed |
Magnetic resonance |
|
Ultrasound |
tomography |
imaging |
|
|
|
|
Portability |
Yes |
No |
No |
Imparts radiation |
No |
Yes |
No |
exposure |
|
|
|
Multiplanar imaging |
Single plane |
Multiplanar (2 |
Multiplanar (3 |
|
|
planes)a |
planes) |
Strength of imaging |
Cystic lesions |
Bone/soft tissue |
Soft tissue interfaces |
|
|
interface |
|
|
|
|
|
aThe third (sagittal) imaging plane may be viewed from reconstructed images, but this consumes additional time and is not yet widely employed intraoperatively.