
Учебники / Computer-Aided Otorhinolaryngology-Head and Neck Surgery Citardi 2002
.pdf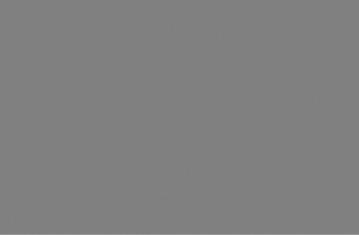
A Neurosurgical Perspective |
175 |
FIGURE 10.3. Deformable atlas applied to the hippocampus. This bilateral structure has been automatically segmented from the overlying tissues by a process that uses an automated protocol.
countered that must be identified before proceeding further. Several deformable atlases have now been produced for neurosurgical applications (Nowinski et al., 1997, 1998), although there is still some skepticism about their value in conservative circles.
10.6CURRENT APPLICATIONS IN NEUROSURGERY
Computer-aided surgery techniques have been found to be valuable in diverse neurosurgical procedures, including tumor removal and ablation, treatment of arteriovenous malformations, functional neurosurgery for the treatment of epilepsy or tremor-inducing conditions, and implantation of devices for the control of hydrocephalus.
10.6.1 Tumors
CAS is of great value for planning tumor-removal procedures. The actual location of certain types of tumor with distinct margins is usually apparent in preoperative imaging. However, some forms of tumor are not so easily delineated. Also, with deep-seated tumors, it is necessary to plan an approach that avoids causing damage to functional cortex. Postoperative imaging will reveal whether the tumor
176 |
Bucholz and Laycock |
has been fully excised, and a follow-up surgery can be planned to remove any residual material. However, it is clearly preferable to remove the tumor in a single procedure if possible.
Primary glial neoplasms, especially astrocytomas, have an infiltrating nature that makes them very difficult to resect in their entirety, resulting in a high recurrence rate. Image guidance can facilitate a more complete excision of the tumor, either during the initial surgery or in a subsequent procedure to remove a recurrent growth.
In patients with intracranial meningiomas, finding and delineating the tumor is not normally a problem, as it is usually clearly visible on the patient’s image set. Thus, the application of frame-based stereotactic techniques was limited to planning of the craniotomy over the tumor location. However, the advent of freehand surgical navigation systems has permitted more extensive use of CAS in meningioma surgery. In addition to planning the craniotomy location and approach to the tumor, CAS techniques are now used to avoid vascular structures and sinuses and to determine the position of the instruments inside the large, featureless mass of the tumor. Another possible application of CAS for meningioma treatment would be the placement of radioactive seeds directly inside tumors that are otherwise inoperable.
10.6.2 Vascular Malformations
Intracerebral vascular malformations normally require surgical intervention to prevent a future hemorrhage and the attendant neurological deficits. Excision of such malformations is particularly difficult when they occur in eloquent areas of the brain such as those responsible for speech or motor-sensory functions. CAS technology enables the malformation to be modeled in three dimensions and thus permits the optimal resection to be planned, taking into account the relationships of the feeding and draining vessels.
10.6.3 Ventriculostomy
The most common application of the neuroendoscope is in the performance of third ventriculostomies in patients with obstructive hydrocephalus or aqueductal stenosis (Kelly, 1991; Dalrymple and Kelly, 1992; Drake, 1993). Obviously, the ventricles must be large enough to accommodate the endoscope in order for this procedure to be performed, and once the endoscope is successfully inserted it is frequently possible to proceed on the basis of visual guidance. However, com- puter-based image guidance can ensure a straight trajectory from the burr hole through the foramen of Monroe to the floor of the third ventricle and help avoid unseen branches of the basilar artery. Image guidance is also of particular value in cases of abnormal anatomy, such as patients with myelomeningoceles or small
A Neurosurgical Perspective |
177 |
foramina of Monroe, or where orientation becomes difficult owing to bloody or blurry cerebrospinal fluid (Muacevic and Muller, 1999). Recent developments in the field of virtual endoscopy promise to aid in the planning and conduct of these procedures (Burtscher et al., 2000)
10.6.4 Functional Neurosurgery
Patients with medically intractable epilepsy are now frequently referred for functional neurosurgery to alleviate their condition. In order to treat the condition surgically, it is first necessary to identify and localize the seizure focus within the brain. Once localized, the region can be eradicated. These processes require accurate spatial localization during preoperative imaging and electro-physiologi- cal investigations, and accurate co-registration of the patient data with the surgical field is essential to prevent destruction of uninvolved tissue.
While the advent of CT and MR imaging had a significant impact on epilepsy management, the more recent development of PET, SPECT, functional MRI (fMRI), and magnetic source imaging (MEG) has enabled greater understanding of the underlying neurophysiology and has permitted refinement of treatment protocols. The ability to co-register each of the imaging modalities with the others (and with the patient) is fundamental to the process of determining the nature of the pathology in a given patient.
Video-EEG recordings of actual seizure events also provide vital information for determining which cases are suitable for surgery. Invasive recording techniques are used to evaluate more difficult cases, and placement of the required recording hardware can be facilitated by image guidance. A common approach involves the insertion of depth electrodes into critical mesial structures to localize the seizure origins (McCarthy et al., 1991). Another evaluation technique requires the placement of subdural strip electrodes on the cortical surface. Accurate placement of subdural strips is crucial, and frameless image guidance is potentially of great assistance in the process, though many centers still place the strips without such guidance. A variation on this approach uses subdural grid electrodes, which are similar in construction to the strips but have the contacts arranged in a series of rows and columns embedded in a flexible sheet. At present these are usually placed without stereotactic guidance, but employment of navigational techniques would facilitate more accurate positioning.
Therapeutic procedures for treating seizure disorders associated with structural lesions have been highly successful in producing seizure-free outcomes. A summary of a combined series of 293 lesionectomy cases reported a seizure-free outcome in 66.6% of cases and noted improvement in an additional 21.5% of cases (Engel et al., 1993). These results are comparable to seizure-outcome results achieved with temporal lobe resections and considerably better than can be expected with nonlesional extratemporal resections. Similarly, Piepgras et al. (1993)
178 |
Bucholz and Laycock |
reported a series of 102 patients with seizures apparently arising from arteriovenous malformations and found that 83% were seizure free at long-term followup.
All such surgical interventions for seizure disorders benefit considerably from CAS, since it is desirable to fully eliminate the causative lesion, while minimizing damage to surrounding tissue. However, it has been found that the optimal resection to achieve a seizure-free outcome may require removal of tissue that lies outside the visible lesion, but which is still involved in the seizure focus according to EEG data (Jooma et al., 1995).
It is likely that the future will see development of noninvasive source localization that will obviate the need for surgical implantation of recording electrodes. However, there will still be a requirement for CAS in therapeutic surgery. Improvements in noncontact registration should enable the registration of patient image data to be accomplished and updated more rapidly. Finally, it is anticipated that real-time electrocorticography and similar recording techniques will enable intraoperative evaluation of the effectiveness of the surgical procedure, ensuring more complete resection of the seizure focus.
10.6.5 Stereotactic Radiosurgery
Another area in which CAS has been of considerable assistance is stereotactic radiosurgery (SRS), in which one or more highly collimated beams of radiation are directed at a tumor. This is usually an outpatient, nonsurgical procedure using the LINAC scalpel or Gamma Knife. For certain types of brain tumor, this treatment is an effective alternative to conventional surgery. However, the radiation does not remove the tumor (although it may shrink it) and resultant scar tissue may make any future surgery more difficult.
A number of technologies may be used in SRS, each with its preferred applications and limitations.
10.6.5.1 Linear Accelerator (LINAC)
The use of conventional LINAC-based radiation therapy is, at best, rarely indicated for the treatment of pituitary microadenomas, but the approach continues to play an important role in the management of macroadenomas. Reports of visual improvement from conventional radiotherapy for pituitary tumors from the 1970s revealed a range of improvement from 41 to 80%. Trans-sphenoidal surgery, however, has shown a rate of visual improvement of approximately 74–90%, which seems superior overall to radiation only. LINAC-based therapy continues to be widely used as an adjuvant treatment. Radiation injury to the optic nerves or optic chiasm is the most feared complication from conventional radiotherapy of pituitary adenomas.
A Neurosurgical Perspective |
179 |
Although the Gamma Knife has supplanted the use of LINAC in many applications, a recent report found that LINAC treatment of acoustic neuromas gave results comparable to those obtained with the Gamma Knife (Spiegelmann et al., 2001).
10.6.5.2 Gamma Knife
The Gamma Knife was developed by Lars Leksell in Stockholm and was first used to treat a pituitary tumor patient in January 1968. Although ideally suited for irradiation of pituitary tumors, these cases account for only approximately 8% of Gamma Knife cases to date.
The technical features of the device are as follows:
It contains 201 cobalt-60 sources in an approximately hemispherical array. Each source has a tungsten precollimator and a lead collimator. Secondary collimation of each beam is accomplished by a helmet. Sources can be selectively blocked with occlusive plugs to prevent irradia-
tion of specific regions.
Gamma Knife therapy offers numerous advantages over conventional radiotherapy for neurological tumors. It is particularly valuable for treating tumors in close proximity to vital structures that would be damaged by conventional techniques [such as pituitary tumors unsuited to transphenoidal surgery (Hayashi et al., 1999; Landolt and Lomax, 2000; Sheehan et al., 2000), acoustic neuromas (Noren et al., 1993; Pollock et al., 1995; Kondziolka et al., 2000), or tumors adjacent to the optic nerve or brain stem]. The most recent reports indicate that radiosurgery for acoustic neuroma performed using current procedures is associated with lower rates of posttreatment morbidity than in earlier reports (Flickinger et al., 2001).
Intracranial arteriovenous malformations and other nontumoral disorders are also often treated with the Gamma Knife (Lundsford et al, 1990; Guo, 1993). The device is occasionally used to treat meningiomas (Kondziolka et al., 1991; Ganz et al., 1993), but slow-growing tumors of this type are usually removed by conventional surgery unless their location dictates the use of radiosurgery. However, Gamma Knife treatment is useful in conjunction with microsurgery for the treatment of skull base meningiomas, where the proximity of the cranial nerves sometimes makes total resection problematic (Aichholzer et al., 2000; Pollock et al., 2000).
Another possible application of the Gamma Knife is in functional surgery, such as the treatment of intractable epilepsy (Regis et al., 2000) or thalamotomy for essential or multiple sclerosis–related tremor (Niranjan et al., 2000).
Other advantages of Gamma Knife treatment include the following:
180 |
Bucholz and Laycock |
Stereotactic radiosurgery of tumors with the Gamma Knife is generally administered in a single session, unlike fractionated LINAC-based radiotherapy, which is administered four to five times per week over a 6-week period.
Because of the nature of the Gamma Knife therapy and the fact that the radiation dose conforms to the tumor shape, there is a steep fall-off of radiation dosage to surrounding tissue such as the brain. Development of secondary tumors or neurocognitive complications is therefore much less likely with Gamma Knife than with conventional radiotherapy.
Gamma Knife radiosurgery is safe as long as the dose of radiation to the optic structures is kept under 10 Gy. Treatment mortality is zero. Longterm follow-up is required.
10.6.5.3 Particle Beam
Proton beam therapy offers certain theoretical advantages over Gamma Knife and LINAC because it makes use of the quantum wave properties of protons to reduce doses to surrounding tissue beyond the target to a theoretical minimum of zero. This is particularly useful when treating unusually shaped brain tumors and arteriovenous malformations. The circular collimators currently in use in photon radiosurgery units result in roughly spherical or elliptical target volumes, and the homogeneous doses delivered also make fractionated therapy possible; no portion of the target in a proton field is likely to receive a complicationproducing dose because of superimposition of high-dose areas.
Like the Gamma Knife, stereotactic proton beam therapy was seen as a possible treatment for intracranial arteriovenous malformations (AVMs) and cavernous malformations (CMs) that were otherwise inaccessible. However, recent reports indicate that the therapy is ineffective for the treatment of mediumor large-sized AVMs larger than 3 cm (Seifert et al., 1994), and a retrospective study concluded that although radiosurgery does seem to reduce hemorrhage in such CMs, there is potential for complications and continued lesion progression afterwards (Amin-Hanjani et al., 1998).
10.7CONCLUSION
As can be seen from this review, the field of CAS has grown dramatically by employing the power of current computers and technology. Based upon the advances made in imaging, CAS has gone from a few applications limited to the midline of the brain to being employed in nearly every cranial procedure. As this technology improves, and as more effectors are built that rely upon precise placement, CAS will become the standard of care for all cranial interventions. This technology will provide the infrastructure upon which the future of neurosur-
A Neurosurgical Perspective |
181 |
gery will grow, and attention to the concepts and assumptions behind CAS will ensure that the technology is used appropriately.
REFERENCES
Adler JR Jr. Image-based frameless stereotactic radiosurgery. In: Maciunas RJ, ed. Interactive Image-Guided Neurosurgery. Park Ridge, IL: American Association of Neurological Surgeons, 1993. 81–89.
Aichholzer M, Bertalanffy A, Dietrich W, Roessler K, Pfisterer W, Ungersboeck K, Heimberger K, Kitz K. Gamma knife radiosurgery of skull base meningiomas. Acta Neurochirurg 2000; 142:647–652, discussion 652–653.
Amin-Hanjani S, Ogilvy CS, Candia GJ, Lyons S, Chapman PH. Stereotactic radiosurgery for cavernous malformations: Kjellberg’s experience with proton beam therapy in 98 cases at the Harvard Cyclotron. Neurosurgery 1998; 42:1229–1236; discussion 1236–1238.
Barnett GH, Kormos DW, Steiner CP, et al. Intraoperative localization using an armless, frameless stereotactic wand. J Neurosurg 1993; 78:510–514.
Barnett GH, Kormos DW, Steiner CP, et al. Frameless stereotaxy using a sonic digitizing wand: Development and adaptation to the Picker Vistar medical imaging system. In: Maciunas RJ, ed. Interactive Image-Guided Neurosurgery. Park Ridge, IL: American Association of Neurological Surgeons, 1993:113–120.
Bucholz RD, Smith KR. A comparison of sonic digitizers versus light-emitting diodebased localization. In: Maciunas RJ, ed. Interactive Image-Guided Neurosurgery. Park Ridge, IL: American Association of Neurological Surgeons, 1993:179–200.
Burtscher J, Dessl A, Bale R, Eisner W, Auer A, Twerdy K, Felber S. Virtual endoscopy for planning endoscopic third ventriculostomy procedures. Pediatric Neurosurg 2000; 32:77–82.
Dalrymple SJ, Kelly PJ. Computer-assisted stereotactic third ventriculostomy in the management of non-communicating hydrocephalus. Stereotact Funct Neurosurg 1992; 59:105–110.
Drake JM. Ventriculostomy for treatment of hydrocephalus. Neurosurg Clin North Am 1993; 4:657–666.
Engel J Jr, Van Ness PC, Rasmussen TB, et al. Outcome with respect to epileptic seizures. In: Engle J Jr, ed. Surgical Treatment of the Epilepsies. New York: Raven Press, 1993:609–621.
Flickinger JC, Kondziolka D, Niranjan A, Lunsford LD. Results of acoustic neuroma radiosurgery: an analysis of 5 years’ experience using current methods. J Neurosurg 2001; 94:1–6.
Ganz JC, Backlund EO, Thorsen FA. The results of Gamma Knife surgery of meningiomas, related to size of tumor and dose. Stereotactic Funct Neurosurg 1993; 61:23– 29.
Guo W. Radiological aspects of Gamma Knife radiosurgery for arteriovenous malformations and other non-tumoural disorders of the brain. Acta Radiol Suppl 1993; 34: 388.
182 |
Bucholz and Laycock |
Guthrie BL, Adler JR Jr. Computer-assisted preoperative planning, interactive surgery, and frameless stereotaxy. Clin Neurosurg 1992; 38:112–131.
Hayashi M, Izawa M, Hiyama H, Nakamura S, Atsuchi S, Sato H, Nakaya K, Sasaki K, Ochiai T, Kubo O, Hori T, Takakura K. Gamma Knife radiosurgery for pituitary adenomas. Stereotact Funct Neurosurg 1999; 72 (Suppl 1):111–118.
Jooma R, Yeh H-S, Privitera MD, et al. Lesionectomy versus electrophysiologically guided resection for temporal lobe tumors manifesting with complex partial seizures. J Neurosurg 1995; 83:231–236.
Kelly PJ. Stereotactic third ventriculostomy in patients with nontumoral adolescent/adult onset aqueductal stenosis and symptomatic hydrocephalus. J Neurosurg 1991; 75: 865–873.
Kondziolka D, Lunsford LD, Coffey RJ, Flickinger JC. Gamma Knife radiosurgery of meningiomas. Stereotactic Funct Neurosurg 1991; 57:11–21.
Kondziolka D, Lunsford LD, Flickinger JC. Gamma knife radiosurgery for vestibular schwannomas. Neurosurg Clin of North Am 2000; 11:651–658.
Landolt AM, Lomax N. Gamma knife radiosurgery for prolactinomas. J Neurosurg 2000; 93 (suppl 3):14–18.
Lunsford LD, Coffey RJ, Bissonette D, Flickinger JC. Stereotactic radiosurgery for arteriovenous malformations. Case selection and initial results from the first North American Gamma Unit. Proc Harvard Radiosurgery Update, Boston, 1990.
McCarthy G, Spencer DD, Riker RJ. The stereotaxic placement of depth electrodes in epilepsy. In: Lu¨ders H, ed. Epilepsy Surgery. New York: Raven Press, 1991:371– 384.
Macewen W. Intra-cranial lesions, illustrating some points in connexion with the localization of cerebral affections and the advantages of antiseptic trephining. Lancet 1881; 2:541–543.
Maciunas RJ, Fitzpatrick JM, Galloway RL, et al. Beyond stereotaxy: Extreme levels of application accuracy are provided by implantable fiducial markers for interactive image-guided neurosurgery. In: Maciunas RJ, ed. Interactive Image-Guided Neurosurgery. Park Ridge, IL: American Association of Neurological Surgeons, 1993: 259–270.
Maurer CM, Fitzpatrick JM. A review of medical image registration. In: Maciunas RJ, ed. Interactive Image-Guided Neurosurgery. Park Ridge, IL: American Association of Neurological Surgeons, 1993:17–44.
Muacevic A, Muller A. Image-guided endoscopic ventriculostomy with a new frameless armless neuronavigation system. Comp Aid Surg 1999; 4:87–92.
Niranjan A, Kondziolka D, Baser S, Heyman R, Lunsford LD. Functional outcomes after gamma knife thalamotomy for essential tremor and MS-related tremor. Neurology 2000; 55:443–446.
Noren G, Greitz A, Lax I. Gamma Knife surgery in acoustic tumors. Acta Neurochir (Wien) 1993; 58:104–107.
Nowinski WL, Bryan RN, Raghavan R. The Electronic Clinical Brain Atlas. Multi-Planar Navigation of the Human Brain. New York: Thieme, 1997.
Nowinski WL, Yeo TT, Thirunavuukarasuu A. Microelectrode-guided functional neurosurgery assisted by Electronic Clinical Brain Atlas CD-ROM. Comp Aid Surg 1998; 3:115–122.
A Neurosurgical Perspective |
183 |
Pelizzari CA, Chen GTY, Spelbring DR, Weichselbaum RR, Chen C. Accurate threedimensional registration of CT, PET, and/or MR images of the brain. J Comput Assist Tomogr 1989; 13:20–26.
Piepgras DG, Sundt TM Jr., Ragoowansi AT, et al. Seizure outcome in patients with surgically treated cerebral arteriovenous malformations. J Neurosurg 1993; 78:5– 11.
Pollock BE, Lunsford LD, et al. Outcome analysis of acoustic neuroma management: a comparison of microsurgery and stereotactic radiosurgery. Neurosurgery 1995; 36: 215–229.
Pollock BE, Stafford SL, Link MJ. Gamma knife radiosurgery for skull base meningiomas. Neurosurg Clin North Am 2000; 11:659–666.
Regis J, Bartolomei F, Hayashi M, Roberts D, Chauvel P, Peragut JC. The role of gamma knife surgery in the treatment of severe epilepsies. Epileptic Disord 2000; 2:113– 122.
Roberts DW, Strohbehn JW, Hatch JF, et al. A frameless stereotaxic integration of computerized tomographic imaging and the operating microscope. J Neurosurg 1986; 64: 545–549.
Seifert V, Stolke D, Mehdorn HM, Hoffmann B. Clinical and radiological evaluation of long-term results of stereotactic proton beam radiosurgery in patients with cerebral arteriovenous malformations. J Neurosurg 1994; 81:683–689.
Sheehan JM, Vance ML, Sheehan JP, Ellegala DB, Laws ER. Radiosurgery for Cushing’s disease after failed transsphenoidal surgery. J Neurosurg 2000; 93:738–742.
Spiegelmann R, Lidar Z, Gofman J, Alezra D, Hadani M, Pfeffer R. Linear accelerator radiosurgery for vestibular schwannoma. J Neurosurg 2001; 94:7–13.
Watanabe E. The neuronavigator: A potentiometer-based localization arm system. In: Maciunas RJ, ed. Interactive Image-Guided Neurosurgery. Park Ridge, IL: American Association of Neurological Surgeons, 1993:135–148.
West J, Fitzpatrick JM, Wang MY, et al. Comparison and evaluation of retrospective intermodality image registration techniques. J Comput Assist Tomogr 1997; 21: 554–566.
Zernov DN. L’ence´phalome`tre. Rev Gen Clin Ther 1890; 19:302.

11
Role of Computer-Aided Surgery in
Functional Endoscopic Surgery
Stephanie A. Joe, M.D., and
David W. Kennedy, M.D., F.R.C.S.I.
University of Pennsylvania Health System, Philadelphia,
Pennsylvania
11.1INTRODUCTION
The treatment of sinonasal disease is based on patient symptomatology and diagnostic information obtained from the combination of endoscopy and radiography. Both endoscopic and diagnostic radiographic techniques have rapidly improved in recent years; simultaneously, the characterization of paranasal sinus disease has also progressed in parallel with these technological advances. Computed tomography (CT) scans are routinely used in the initial assessment of sinus disease and for surgical planning. Magnetic resonance (MR) images can provide valuable soft tissue information in more unusual situations. Advanced computer technology allows ready manipulation of the digitally stored images, and advances in digitization of three-dimensional objects have made computer-aided surgery a viable reality. These tools and other advances in surgical instrumentation have assisted in the refinement of sinus surgery and aided in the extension of endoscopic procedures to include more invasive surgical approaches involving both sinus and parasinus pathology.
The adaptation of computer-aided surgery (CAS) for intraoperative localization in otorhinolaryngology has proven to be a beneficial supplement to functional
185