
Учебники / Computer-Aided Otorhinolaryngology-Head and Neck Surgery Citardi 2002
.pdfVirtual Reality and Surgical Simulation |
113 |
7.5.7 Virtual Instructor
A virtual instructor named Martin was created for the simulator. Martin provides hands-free control of the simulator so that a simulator user can train without assistance from another person. Martin serves two purposes. He acts as the ‘‘surgical technician’’ providing the student surgeon instruments at the student’s voice command, and he has built-in intelligence in the form of procedural knowledge. Martin tracks the progress of the student and can provide the student with recommendations for the next surgical sequence.
Martin recognizes a wide variety of voices and responds to over 60 commands. He has a vocabulary of over 180 words and recognizes multiple words for the same command. For example, a user may say ‘‘hummer’’ or ‘‘microdebrider’’ to select the same tool. The sequence of events that causes the simulator to react to spoken commands is as follows. The student surgeon issues a command that is transmitted as corresponding string over the Ethernet connection to the simulation computer. A background task in the simulation computer decodes the string, places the command into shared memory, and forwards the string to the audio server. The real-time software acts on the command. The audio server fetches the appropriate file and plays it through the speakers. The student hears and sees the response, closing the loop.
7.5.8 Endoscopic Sinus Simulator Evaluation
The Madigan sinus surgical simulator was evaluated by the Human Interface Technology Lab at the University of Washington. In general, two types of evaluations were performed during development. Formative evaluations provided design specifications input to the development team, and summative evaluation assessed the success of that effort by formally analyzing the system’s effectiveness.
The findings of the evaluation team suggest that the simulator represents a valid and useful implementation of the target endoscopic sinus surgery tasks. In addition, the thoughtful integration of an organized curriculum perspective makes this system uniquely valuable among emerging medical simulation systems [12].
7.6 TRANSFER OF TRAINING
The use of VR and related techniques for medical training is clearly embryonic. Nevertheless, a significant portion of any development team’s time and resources should be dedicated towards determining training efficacy and cost-effectiveness. Computer-based simulation in its present state is expensive and unproved. Assessments of training effectiveness can conserve scarce resources, which may be better expended on other projects.
114 |
Edmond |
The basic measure of training efficacy is percent transfer of training. In aviation, the transfer effectiveness ratio (TER) has been used to monitor the efficiency of training simulations. Research has suggested that such simulations can yield a TER of .48, indicating that one hour in a simulator saves approximately one-half hour in the air [13]. The TER also addresses which method of training provides the greatest learning in the least amount of time. If one training technique costs much less than another but takes a lot longer for a student to reach a certain level of proficiency, a TER experimental design can determine the costeffectiveness of each training program per hour of training device operation [14]. It is clear that if surgeons cannot perform efficiently in virtual environments, then further pursuit of this technology may be fruitless.
The author recently completed a pilot study evaluating the transfer effectiveness of the sinus surgical simulator. The results, which will be formally presented in an upcoming publication, demonstrate positive effect of the Madigan sinus surgical simulator on the development of critical surgical skills.
7.7 SUMMARY
In the twenty-first century, simulators have the potential to play a tremendous role in the training and credentialing of surgeons. The magnitude of the role will depend on advances that are made in creating more realistic visual imagery, development of haptic sensations that are realistic and convincing, and production of training systems that are affordable. Developers of these systems must continue to assess transfer of training and continue to seek out and explore the emerging technologies (including artificial intelligence, tele-proctoring, tele-mentoring, and tele-presence) that can be used to either enhance the functionality of the simulators or as adjuncts to conventional training.
ACKNOWLEDGMENTS
This work is supported by the U.S. Army Medical Research and Material Command under cooperative agreement DAMD 17–95–2-5023. The author wishes to express his gratitude to Dave Heskamp and Jeff Miller of Lockheed Martin Tactical Defense Systems, Akron, OH; Douglas Sluis, Ph.D., of ATL Inc., Bothell, WA; Donald Stredney and Dennis Sessana of Ohio Supercomputer Center, Columbus, OH; Roni Yagel, Ph.D., and Naeem Shareef of the Department of Computer and Information Science, OSC, Columbus, OH.; Suzanne Weghorst, Peter Oppenheimer, Chris Airola, and Mark Billinghurst of the University of Washington—Human Interface Technology Laboratory, Seattle, WA; and Michael Levin and Louis Rosenberg, Ph.D., of Immersion Corp., San Jose, CA. The above individuals have contributed significantly to the success of our project.
Virtual Reality and Surgical Simulation |
115 |
REFERENCES
1.Edmond C, Heskamp D, Sluis D, Stredney D, Wiet G, Yagel R, Weghorst S, Miller J, Levin M. ENT Surgical Training Simulator. Stud. Health Technology Informatics, 1997, No. 39, pp. 518–528.
2.Edmond C, Wiet G, Bolger B. Virtual environments surgical simulation in otolaryngology. Otolaryngol Clin North Am. April, 1998.
3.Rosenberg L, Stredney D. A haptic interface for virtual simulation of endoscopic surgery. In: Health Care in the Information Age. Amsterdam, The Netherlands, IOS Press, 1996, pp. 371–387.
4.Aukstakalnis S, Blatner D, eds. Silicon Mirage: The Art and Science of Virtual Reality, Berkeley, CA: Peachpit Press, 1992.
5.Billinghurst M, Savage P, Oppenheimer P, Edmond C. Expert surgical assistant: an intelligent virtual environment with multimodal input. In: Health Care in the Information Age. Amsterdam, The Netherlands, IOS Press and Ohmsha, 1996.
6.Cohen PR, Dalrymple M, Pereira FCN, Sullivan JW, Gargan Jr., RA, Schlossberg JL, Tyler SW. Synergistic use of direct manipulation and natural language. Conference on Human Factors in Computing Systems (CHI ’’89). Austin, TX, IEEE, ACM, 1989, pp. 227–233.
7.Cohen PR. The role of natural language in a multimodal interface. UIST Proceedings, 1992, pp. 143–149.
8.Hauptman AG, McAvinny P. Gestures with Speech for Graphics Manipulations. Int
JMan-Machine Studies, 38:231–249, 1993.
9.Bolt RA. ‘‘Put-that-there’’: voice and gesture at the graphics interface. Computer Graphics 14(3):262–270. In: Proceeding of ACM SIGGRAPH, 1980.
10.Buchanan BG, Shorlife EH. Rule based expert systems: The MYCIN Experiments of the Stanford Heuristic Programming Project. Reading, MA: Addison Wesley, 1984.
11.Weimer D, Ganapathy SK. A synthetic visual environment with hand gesturing and voice input. Conference on Human Factors in Computing Systems (CHI ’89). Austin, TX, IEEE, ACM, April 30–May 4, 1989, pp. 235–240.
12.Weghorst S, Airola C, Oppenheimer P, Edmond C, Patience T, Heskamp D, Miller
J.Validation of the Madigan endoscopic sinus simulator. Stud. Health Technology Informatics 50:399–408, 1998.
13.Johnson R, Bhoyrul S, Way L, Satava R, McGovern K, Fletcher D, Rangel S, Loftin
B.Assessing a virtual reality surgical skills simulator. Health Care in the Information Age. Amsterdam, The Netherlands, IOS Press, pp. 608–617.
14.Kozak JJ, Hancock PA. Transfer of Training from VIZ Ergonomics.

8
Digital Imaging in
Otorhinolaryngology–Head
and Neck Surgery
Eiji Yanagisawa, M.D., F.A.C.S.
Yale University School of Medicine, Yale–New Haven Hospital, and Hospital of St. Raphael, New Haven, Connecticut
John K. Joe, M.D.
Memorial Sloan-Kettering Cancer Center, New York,
New York
Ray Yanagisawa, B.A.
Woodbridge, Connecticut
8.1 INTRODUCTION
Digital imaging represents one of the most significant recent technological advances in both the consumer and professional markets. The downside to writing about any groundbreaking technology is that, by the time the article makes it into print, the subject matter may have become passe´. Despite this caveat, the continuing implementation of digital imaging in the field of medicine warrants discussion about its clinical applications in otolaryngology and head and neck surgery.
117
118 |
Yanagisawa et al. |
8.2 FUNDAMENTALS OF DIGITAL IMAGING
8.2.1 Image
Photodocumentation of still images has traditionally been captured onto 35 mm negative or slide film, and moving images have traditionally been recorded on VHS, SVHS, Hi8, or 3/4 inch videotape [1–4]. Such analog video input captures images as a continuous stream of varying signal strengths on videotape.
In contrast, a digital camera utilizes a charge-coupled device (CCD) in place of film. A CCD is a silicon chip composed of individual pixels that transform light into voltage in a manner similar to the transformation of chemical emulsions into silver grains on film [5]. A computer within the camera converts this voltage into binary data as a series of ones and zeroes. These discrete binary units provide the fundamental difference between a digital image and the continuous spectrum of values of an analog signal.
By placing a red, green, or blue filter in front of the CCD, the chip is able to digitize the individual color components of an image for color representation. The resolution of a camera refers to its density of light-sensitive picture elements, or pixels. The degree of recorded detail varies directly with the resolution of the recorded digital image; that is, the greater the resolution of a digital image, the higher degree of recorded detail. A greater resolution allows image enlargement with less degradation of picture quality from discrete separation between adjacent points in the image (a phenomenon called pixelation) [6]. The gold standard for image quality is the typical 35 mm Kodachrome slide, which contains silver grains at a density of approximately 2500 lines per inch, or 4096 2736 pixels per frame. Data from the dermatology literature indicate that the minimum resolution needed to recognize relevant details of patient images is 768 512 pixels [5]. Although a higher resolution image provides greater detail, the resulting greater file size presents several downsides as well. A higher resolution image requires more memory for storage, more powerful processors for manipulation, and faster networks for transmission.
8.2.2 Compression
To compensate for the greater hardware requirements necessary for higher resolution, an image may be compressed by one of two methods to decrease its file size [5,6]. One approach retains all image information and is commonly called ‘‘lossless.’’ The other approach is termed ‘‘lossy,’’ since various amounts of redundant color data are discarded. Lossy compression is commonly used in digital cameras at the time of this writing. Reducing the size of the image file through compression is ideal if memory space is a concern or if network data transmission rates are slow. Even with compression, however, the tradeoff between maximal
Digital Imaging |
119 |
resolution for optimal image quality and the smallest possible file size remains a dilemma.
8.3 TECHNIQUES OF DIGITAL IMAGING
There are three primary approaches to creating digital images [7]. The first is to convert a photograph or slide into a still digital image using an image scanner connected to a computer. The second is to convert an analog signal from a video camera or videotape into streaming digital video with an image capture board installed in a computer. This method is limited by the relatively low resolution of the analog video signal. Using this method, the senior author (EY) has produced excellent images for his publications [1–4,8,9]. He uses 3/4-inch prerecorded videotapes, a Macintosh computer, Avid Media Suite Pro video editing software, Adobe Photoshop, and a Sony digital dye-sublimation printer. The third method is to capture the image directly with a charge-coupled device digital camera. This method bypasses conversion and starts with a digital image at the time of capture.
8.4 HARDWARE REQUIREMENTS
Components of a digital imaging system include a digital camera, computer, annotation software, removable memory storage, digital video recorder, color printer, and digital projector.
8.4.1 Digital Cameras
Digital still cameras, like conventional film-based cameras, come in point-and- shoot and single lens reflex (SLR) models [5,10–12]. Utilizing fixed lenses and a built-in flash, point-and-shoot cameras are inexpensive, easy to use, and generally smaller and lighter than SLR cameras. The disadvantages of point-and-shoot models include less flexibility in controlling the features of the camera and lower resolution. In contrast, SLR cameras have modified the typical film-based 35 mm camera by replacing the film advance system with the CCD and processing hardware and a LCD (liquid crystal diode) screen. SLR-derived digital cameras are usually larger and more expensive but produce superior quality images.
For medical use, one should choose a megapixel digital camera, which produces high-quality images. Some of the digital still cameras commercially available at the time of this writing are illustrated in Figure 8.1. The Olympus C-3030 camera is shown in Figure 8.1A. This model is a 3.3 megapixel camera with 2048 1536 resolution (approximately $990 at the time of this writing). The Nikon Coolpix 950 (approximately $650) is a 2.11 megapixel camera with 1600 1200 resolution (Figure 8.1B). The Sony Mavica MVC-FD95 digital still
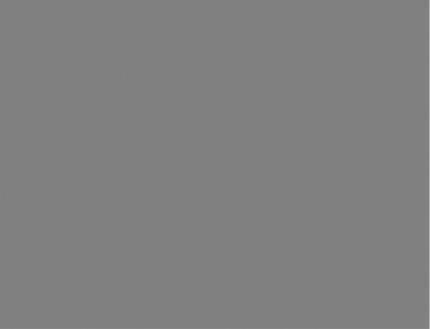
120 |
Yanagisawa et al. |
FIGURE 8.1 Digital still cameras: (A) Olympus C-3030 digital camera with a Smart Media card and a floppy disk adapter; (B) Nikon Coolpix 950 digital camera with a Compact Flash card; (C) Sony Mavica FD-95 digital camera; (D) Sony Cyber-shot DSC-F505V digital camera. The insert between C and D shows a Sony Memory Stick with a floppy disk adapter.
camera (approximately $900) is illustrated in Figure 8.1C. It has a 1600 1200 pixel resolution, a Carl Zeiss lens, and 10 optical/20 digital zoom. Figure 8.1D shows the Sony Cyber-shot DSC-F505V digital still camera (approximately $900), which has a 5 optical/10 digital zoom.
These digital still cameras include models with which we are familiar. Other digital still cameras available at the time of this writing include the Epson Photo PC 3000Z (3.3 megapixel camera), the Kodak DC290 Zoom (2.1 megapixel camera with 1792 1200 resolution), the Sony Mavica MVC-FD73 (640 480 resolution), the Sony Mavica MVC-CD1000 (2.1 megapixel with 1600 1200 resolution), the HP Photo Smart 315 (2.1 megapixel with 1600 1200 resolution and 2.5 digital zoom), and the HP Photosmart 912 (2.24 megapixel with 1600 1280 resolution and 3 optical/2 digital zoom). The aforemen-
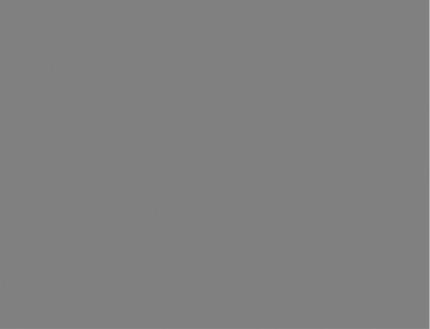
Digital Imaging |
121 |
tioned list of digital still cameras is intended to be comprehensive. In addition, the authors do not wish to state and/or imply an endorsement for any particular model.
Although some current digital still cameras are able to record several seconds of moving video segments, other digital cameras are dedicated to the capture of longer segments of digitized streaming video images (Figure 8.2). The camera pictured in Figure 8.2A is an analog Stryker 888 3-chip CCD camera utilized by otolaryngologists for documentation of sinonasal or laryngeal endoscopy. Although this Stryker camera captures an analog signal, the images may be recorded onto either an analog or digital video recorder. In contrast, the cameras illustrated in Figures 8.2B–D represent digital camcorders (also called DV or DVCAM camcorders) that capture a digitized signal. The Canon ZR-10 digital video camcorder (approximately $900) is shown in Figure 8.2B. The Sony DCR-PC100 Mini-DV video camcorder (approximately $1,800) is pictured in Figure 8.2C.
FIGURE 8.2 Digital video cameras and camcorders: (A) Stryker 888 3-chip CCD camera; (B) Canon ZR-10 digital video camcorder; (C) Sony DCR-PC100 Mini-DV video camcorder; (D) Sony professional DSR-PD150 DVCAM camcorder.
122 |
Yanagisawa et al. |
Although the cameras previously described represent models manufactured for consumer use, Figure 8.2D illustrates a representative Sony DVCAM DSRPD150 camcorder (approximately $4,000) for the professional market.
8.4.2 Computer
The computer utilized in a digital imaging system may be either a PC or a Macintosh, although compatibility of images across both platforms is ideal to ensure the widest possible audience. Because higher resolution images tend to result in larger file sizes, the computer should utilize the largest processing power, hard disk memory capacity, and monitor size that are reasonably affordable.
Digital cameras transfer images to the computer by many ways. Some cameras require direct connection through a serial or SCSI cable. Others use removable cards or diskettes that are placed into an appropriate receiver on the computer, and some even use infrared remote transfer of the data. Some cameras function best with an external card reader [12]. Before acquiring a digital camera, users are encouraged to understand the method and process through which digital images are transferred from the camera to the computer.
8.4.3 Software
Proprietary software is necessary to import images captured with a digital still camera or digital video camcorder onto a computer’s hard drive. Image processing software usually comes with most digital cameras. Once transferred onto the computer, captured images may then be annotated or manipulated using this software. The speed at which images are transferred from the camera to the computer depends on the type of connection established between the two. Newer camera models use faster connections, e.g., USB (universal serial bus).
8.4.4 Removable Storage Media
Removable storage media are necessary with some cameras to store captured images prior to their download onto a computer. These storage cards allow for the camera to function without a physical connection to the computer when images are captured. Different models of digital image storage cards are currently available, and representative types are illustrated in Figure 8.3. Figure 8.3A shows the Olympus Camedia floppy disk adapter for a Smart Media card. A Smart Media card is pictured in Figure 8.3B. This type of storage media is used by several models of digital still cameras, and cards with different amounts of memory are available, including 8, 16, 32, and 64 MB. Cards with greater amounts of memory storage allow for a larger number of images that may be captured prior to download. Figure 8.3C illustrates the Sony floppy disk adapter for a Sony Memory Stick. The Memory Stick, shown in Figure 8.3D, is the proprietary memory stor-
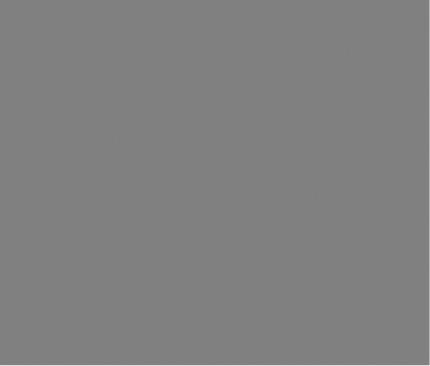
Digital Imaging |
123 |
FIGURE 8.3 Digital image removable storage memory cards: (A) Olympus Camedia floppy disk adapter for Smart Media cards; (B) 8 MB Smart Media card (also available in 16, 32, and 64 MB cards); (C) Sony floppy disk adapter for Sony Memory Stick; (D) 32 MB Sony Memory Stick (also available in 16 and 64 MB sticks); (E) 8 MB Compact Flash card (used by Nikon Coolpix series cameras) (also available in 16, 32, 48, 64, and 128 MB cards).
age card used by Sony digital still cameras. It is also available with a variety of memory sizes, including 16, 32, and 64 MB. The Compact Flash card pictured in Figure 8.3E represents another type of storage media. The Nikon Coolpix digital still cameras use this type of card, which is available with a variety of sizes of memory, including 8, 16, 32, 48, 64, and 128 MB.
One current problem with removable storage media for digital still cameras is the lack of a uniform standard. As shown in Figure 8.1, different camera models utilize different types of proprietary memory cards, which are not cross-compati- ble. This lack of uniformity can be both confusing and expensive for consumers and professionals, who may use more than one model of digital still camera.