
- •Contents
- •Preface to the first edition
- •Flagella
- •Cell walls and mucilages
- •Plastids
- •Mitochondria and peroxisomes
- •Division of chloroplasts and mitochondria
- •Storage products
- •Contractile vacuoles
- •Nutrition
- •Gene sequencing and algal systematics
- •Classification
- •Algae and the fossil record
- •REFERENCES
- •CYANOPHYCEAE
- •Morphology
- •Cell wall and gliding
- •Pili and twitching
- •Sheaths
- •Protoplasmic structure
- •Gas vacuoles
- •Pigments and photosynthesis
- •Akinetes
- •Heterocysts
- •Nitrogen fixation
- •Asexual reproduction
- •Growth and metabolism
- •Lack of feedback control of enzyme biosynthesis
- •Symbiosis
- •Extracellular associations
- •Ecology of cyanobacteria
- •Freshwater environment
- •Terrestrial environment
- •Adaption to silting and salinity
- •Cyanotoxins
- •Cyanobacteria and the quality of drinking water
- •Utilization of cyanobacteria as food
- •Cyanophages
- •Secretion of antibiotics and siderophores
- •Calcium carbonate deposition and fossil record
- •Chroococcales
- •Classification
- •Oscillatoriales
- •Nostocales
- •REFERENCES
- •REFERENCES
- •REFERENCES
- •RHODOPHYCEAE
- •Cell structure
- •Cell walls
- •Chloroplasts and storage products
- •Pit connections
- •Calcification
- •Secretory cells
- •Iridescence
- •Epiphytes and parasites
- •Defense mechanisms of the red algae
- •Commercial utilization of red algal mucilages
- •Reproductive structures
- •Carpogonium
- •Spermatium
- •Fertilization
- •Meiosporangia and meiospores
- •Asexual spores
- •Spore motility
- •Classification
- •Cyanidiales
- •Porphyridiales
- •Bangiales
- •Acrochaetiales
- •Batrachospermales
- •Nemaliales
- •Corallinales
- •Gelidiales
- •Gracilariales
- •Ceramiales
- •REFERENCES
- •Cell structure
- •Phototaxis and eyespots
- •Asexual reproduction
- •Sexual reproduction
- •Classification
- •Position of flagella in cells
- •Flagellar roots
- •Multilayered structure
- •Occurrence of scales or a wall on the motile cells
- •Cell division
- •Superoxide dismutase
- •Prasinophyceae
- •Charophyceae
- •Classification
- •Klebsormidiales
- •Zygnematales
- •Coleochaetales
- •Charales
- •Ulvophyceae
- •Classification
- •Ulotrichales
- •Ulvales
- •Cladophorales
- •Dasycladales
- •Caulerpales
- •Siphonocladales
- •Chlorophyceae
- •Classification
- •Volvocales
- •Tetrasporales
- •Prasiolales
- •Chlorellales
- •Trebouxiales
- •Sphaeropleales
- •Chlorosarcinales
- •Chaetophorales
- •Oedogoniales
- •REFERENCES
- •REFERENCES
- •EUGLENOPHYCEAE
- •Nucleus and nuclear division
- •Eyespot, paraflagellar swelling, and phototaxis
- •Muciferous bodies and extracellular structures
- •Chloroplasts and storage products
- •Nutrition
- •Classification
- •Heteronematales
- •Eutreptiales
- •Euglenales
- •REFERENCES
- •DINOPHYCEAE
- •Cell structure
- •Theca
- •Scales
- •Flagella
- •Pusule
- •Chloroplasts and pigments
- •Phototaxis and eyespots
- •Nucleus
- •Projectiles
- •Accumulation body
- •Resting spores or cysts or hypnospores and fossil Dinophyceae
- •Toxins
- •Dinoflagellates and oil and coal deposits
- •Bioluminescence
- •Rhythms
- •Heterotrophic dinoflagellates
- •Direct engulfment of prey
- •Peduncle feeding
- •Symbiotic dinoflagellates
- •Classification
- •Prorocentrales
- •Dinophysiales
- •Peridiniales
- •Gymnodiniales
- •REFERENCES
- •REFERENCES
- •Chlorarachniophyta
- •REFERENCES
- •CRYPTOPHYCEAE
- •Cell structure
- •Ecology
- •Symbiotic associations
- •Classification
- •Goniomonadales
- •Cryptomonadales
- •Chroomonadales
- •REFERENCES
- •CHRYSOPHYCEAE
- •Cell structure
- •Flagella and eyespot
- •Internal organelles
- •Extracellular deposits
- •Statospores
- •Nutrition
- •Ecology
- •Classification
- •Chromulinales
- •Parmales
- •Chrysomeridales
- •REFERENCES
- •SYNUROPHYCEAE
- •Classification
- •REFERENCES
- •EUSTIGMATOPHYCEAE
- •REFERENCES
- •PINGUIOPHYCEAE
- •REFERENCES
- •DICTYOCHOPHYCEAE
- •Classification
- •Rhizochromulinales
- •Pedinellales
- •Dictyocales
- •REFERENCES
- •PELAGOPHYCEAE
- •REFERENCES
- •BOLIDOPHYCEAE
- •REFERENCE
- •BACILLARIOPHYCEAE
- •Cell structure
- •Cell wall
- •Cell division and the formation of the new wall
- •Extracellular mucilage, biolfouling, and gliding
- •Motility
- •Plastids and storage products
- •Resting spores and resting cells
- •Auxospores
- •Rhythmic phenomena
- •Physiology
- •Chemical defense against predation
- •Ecology
- •Marine environment
- •Freshwater environment
- •Fossil diatoms
- •Classification
- •Biddulphiales
- •Bacillariales
- •REFERENCES
- •RAPHIDOPHYCEAE
- •REFERENCES
- •XANTHOPHYCEAE
- •Cell structure
- •Cell wall
- •Chloroplasts and food reserves
- •Asexual reproduction
- •Sexual reproduction
- •Mischococcales
- •Tribonematales
- •Botrydiales
- •Vaucheriales
- •REFERENCES
- •PHAEOTHAMNIOPHYCEAE
- •REFERENCES
- •PHAEOPHYCEAE
- •Cell structure
- •Cell walls
- •Flagella and eyespot
- •Chloroplasts and photosynthesis
- •Phlorotannins and physodes
- •Life history
- •Classification
- •Dictyotales
- •Sphacelariales
- •Cutleriales
- •Desmarestiales
- •Ectocarpales
- •Laminariales
- •Fucales
- •REFERENCES
- •PRYMNESIOPHYCEAE
- •Cell structure
- •Flagella
- •Haptonema
- •Chloroplasts
- •Other cytoplasmic structures
- •Scales and coccoliths
- •Toxins
- •Classification
- •Prymnesiales
- •Pavlovales
- •REFERENCES
- •Toxic algae
- •Toxic algae and the end-Permian extinction
- •Cooling of the Earth, cloud condensation nuclei, and DMSP
- •Chemical defense mechanisms of algae
- •The Antarctic and Southern Ocean
- •The grand experiment
- •Antarctic lakes as a model for life on the planet Mars or Jupiter’s moon Europa
- •Ultraviolet radiation, the ozone hole, and sunscreens produced by algae
- •Hydrogen fuel cells and hydrogen gas production by algae
- •REFERENCES
- •Glossary
- •Index
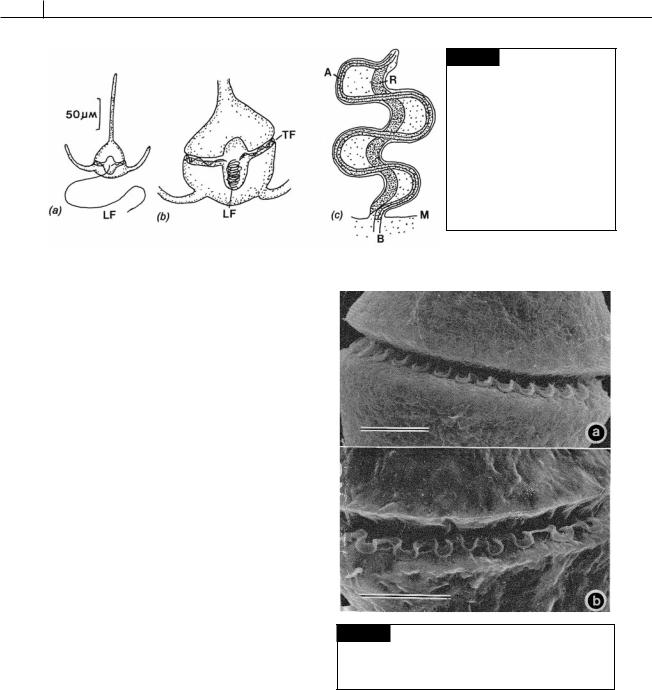
268 CHLOROPLAST E.R.: EVOLUTION OF ONE MEMBRANE
Fig. 7.12 Ceratium tripos. (a) Vegetative cell with a relaxed longitudinal flagellum (LF) at full length. (b) A cell with the longitudinal flagellum (LF) fully contracted and folded in the sulcus. (TF) transverse flagellum. (c) Schematic drawing of a retracted flagellum showing the contracted R- fiber (R) and the axoneme (A). (B) Basal body; (M) plasma membrane. (Adapted from Maruyama, 1982.)
counterclockwise when seen from the cell apex (leiotropic direction). The cell always rotates in the direction of the flagellar beat (in the leiotropic direction), with the fluid propelled in the opposite direction (in the dexiotropic direction) (Fig. 7.15) (Gaines and Taylor, 1985; Fenchel, 2001). The longitudinal flagellum swings in a narrow orbit acting as a rudder.
The dinoflagellates are the fastest swimmers among the algae although they are slower than Mesodinium, the photosynthetic symbiosis between a ciliate and a cryptophyte (see Chapter 9, Cryptophyta, Symbiotic associations). Dinoflagellates swim from 200 to 500 m s 1 (Raven and Richardson, 1984). The cells of Lingulodinium polyedrum (Fig. 7.40) swim at a linear rate of 250 m s 1 at 20 C whereas cells of Gyrodinium swim at a mean linear rate of velocity of 319 m s 1 at 20 °C. Marine dinoflagellates frequently move into deeper, nutrient-rich waters at night and better illuminated waters near the surface during the day. The diel (over a 24-hour period) migrations of dinoflagellates are 5 to 10 m in relatively quiet waters. A dinoflagellate swimming at 500 m s 1 would take about 6 hours of the 24-hour period for 5 m of upward migration around dawn, and a further 6 hours for downward migration around dusk (Raven and Richardson, 1984).
Pusule
A pusule is a sac-like structure that opens by means of a pore into the flagellar canal and probably has an osmoregulatory function similar to that of a contractile vacuole. The pusule of
Fig. 7.13 Scanning electron micrograph showing the form of the transverse flagellum in Akashiwo sanguinea
( Gymnodinium sanguineum) (a) and Gyrodinium uncatenum
(b). Bar 10 m. (From Gaines and Taylor, 1985.)
Amphidinium carteri is representative of those dinoflagellates that have a pusule (Fig. 7.16) (Dodge and Crawford, 1968). In this organism there are two pusules, one associated with each flagellar canal. The pusule consists of about 40 vesicles which open by small pores into the flagellar canal. Whereas the flagellar canal is lined by a single membrane continuous with the plasma
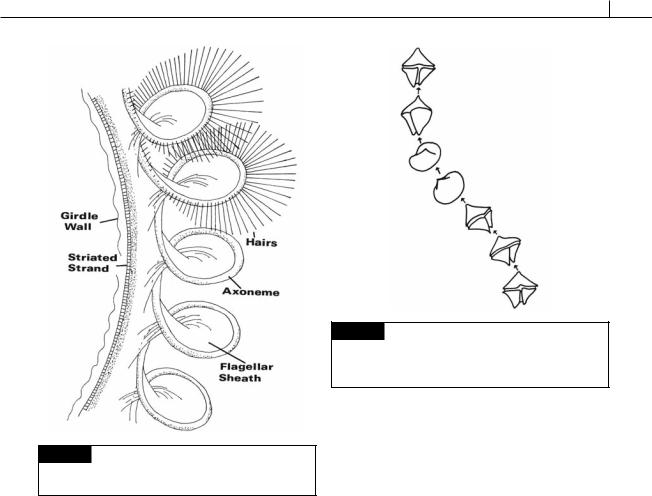
DINOPHYTA 269
Fig. 7.14 Diagram of part of the transverse flagellum of
Peridinium cinctum. Only a portion of the fibrillar hairs have been drawn in. (After Berdach, 1977.)
membrane, the pusule vesicles are lined by a double membrane.
Chloroplasts and pigments
The plastids of most photosynthetic dinoflagellates originated from a secondary endosymbiosis with a red alga. These plastids are surrounded by three membranes (two membranes of chloroplast envelope and one membrane of chloroplast endoplasmic reticulum) (Fig. 7.2) and contain chlorophyll a and c, and peridinin (Fig. 7.17) as the major photosynthetic pigments (Ishida and Green, 2002).
There are a small number of dinoflagllates that have plastids derived from a tertiary endosymbiosis (Fig. 7.18). Tertiary endosymbiosis begins with the loss of the plastid (originally derived from a secondary endosymbiosis) from a dinoflagellate followed by endosymbiosis of an
Fig. 7.15 Tracings from successive video frames of
Protoperidinium conicum swimming, showing the leiotropic rotation of the cell. Time between intervals is 1/5 second. (From Gaines and Taylor, 1985.)
alga from the Prymnesiophyceae (a haptophyte alga). All of the protoplasm of the endosymbiont was lost, except for the chloroplast surrounded by one membrane of chloroplast endoplasmic reticulum, which became the permanent chloroplast of the dinoflagellate. Instead of peridinin, these algae have 19 -hexanoyloxy-fucoxanthin (Fig. 7.17) and/or 19 butanoyloxy-fucoxanthin, pigments characteristic of the Prymnesiophyceae. Karenia brevis (Fig. 7.19(a)), Karenia mikimotoi (Fig. 7.19(b)), and Karlodinium veneficum (Fig. 7.34) are dinoflagellates that are derived from tertiary endosymbioses (Ishida and Green, 2002; Takishita et al., 2005; Yoon et al., 2002).
A number of dinoflagellates contain short-term plastids stolen from their food source (kleptoplastids) and it is sometimes difficult to distinguish between these plastids and permanent plastids (Keeling, 2004). Dinoflagellates have transferred most of the plastid genome to the nucleus of the cell, making them the only eukaryotes that encode the majority of the plastid genes in the nucleus (Hackett et al., 2004). Having the genes for plastidtargeted proteins may actually be an advantage since it provides the cell with the freedom to replace the original plastid with a new one (Green,
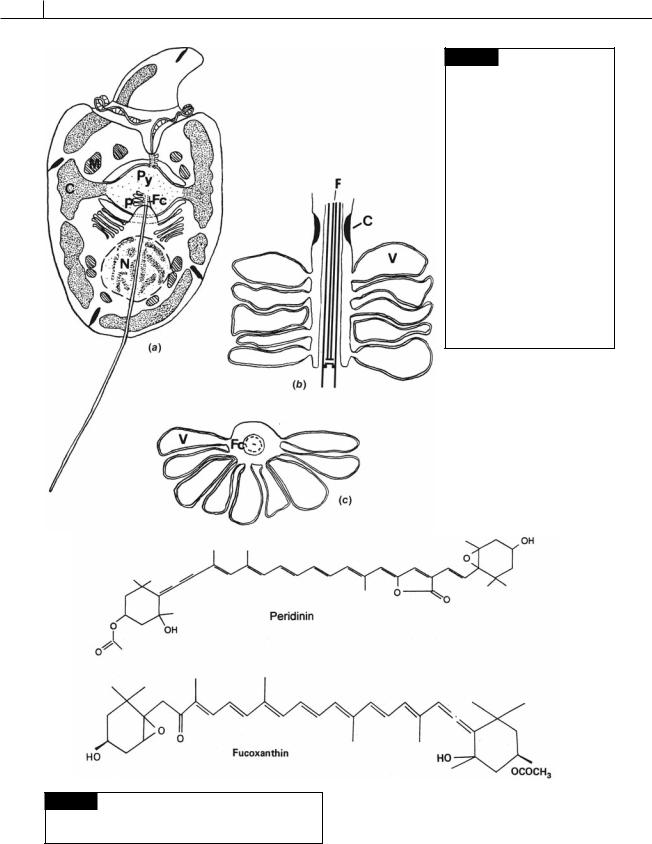
270 CHLOROPLAST E.R.: EVOLUTION OF ONE MEMBRANE
Fig. 7.16 (a) Drawing of
Amphidinium carteri. The small epicone is separated from the hypocone by the encircling girdle, which contains the transverse flagellum. The peripheral chloroplast
(C) is shown only at the sides of the cell so the positions of the nucleus (N), mitochondria (M), pyrenoid (Py), and the pusule (P) associated with the flagellar canals can be seen. (b) Longitudinal section through a pusule showing the flagellar pore constriction (C), the pusule vesicles (V), and the flagellum (F).
(c) Transverse section of a pusule showing the flagellum within the flagellar canal (Fc) and the pusule vesicles (V) opening into the canal. (From Dodge and Crawford, 1968.)
Fig. 7.17 The chemical structure of peridinin and 19 - hexanoyloxy-fucoxanthin. Peridinin has three rings instead of the two found in other light-harvesting carotenoids.
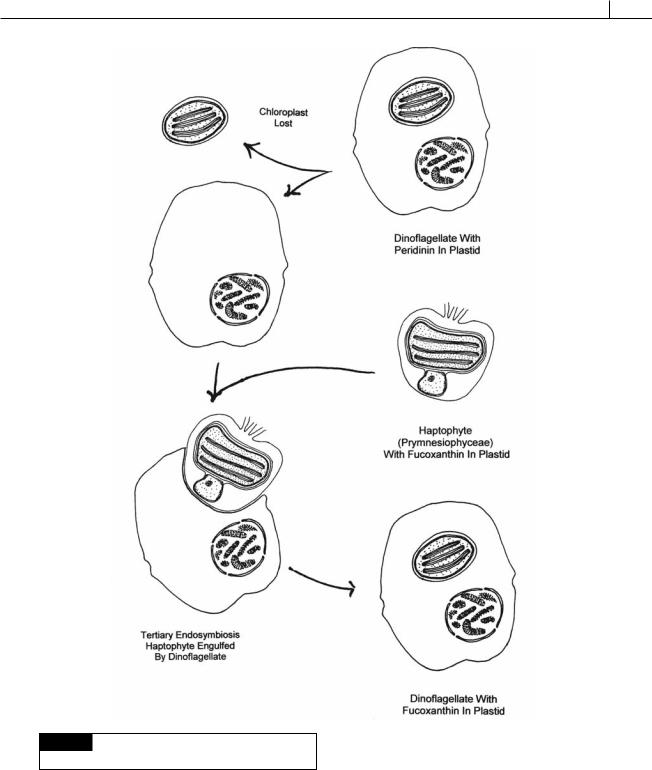
DINOPHYTA 271
Fig. 7.18 The sequence of events leading to plastids
evolved from a tertiary endosymbiosis.
2004). The plastid genome of peridinin-containing dinoflagellates is reduced and broken up into minicircles that encode only 16 proteins.
Most of the chlorophyll a and peridinin occur
together in a water-soluble protein complex called peridinin–chlorophyll a–protein (PCP). Within the chromophore the peridinin and chlorophyll a are in a 4 : 1 ratio (Prézelin and Haxo, 1976). PCP is similar to the phycobiliproteins in cyanobacteria