
- •Contents
- •Preface to the first edition
- •Flagella
- •Cell walls and mucilages
- •Plastids
- •Mitochondria and peroxisomes
- •Division of chloroplasts and mitochondria
- •Storage products
- •Contractile vacuoles
- •Nutrition
- •Gene sequencing and algal systematics
- •Classification
- •Algae and the fossil record
- •REFERENCES
- •CYANOPHYCEAE
- •Morphology
- •Cell wall and gliding
- •Pili and twitching
- •Sheaths
- •Protoplasmic structure
- •Gas vacuoles
- •Pigments and photosynthesis
- •Akinetes
- •Heterocysts
- •Nitrogen fixation
- •Asexual reproduction
- •Growth and metabolism
- •Lack of feedback control of enzyme biosynthesis
- •Symbiosis
- •Extracellular associations
- •Ecology of cyanobacteria
- •Freshwater environment
- •Terrestrial environment
- •Adaption to silting and salinity
- •Cyanotoxins
- •Cyanobacteria and the quality of drinking water
- •Utilization of cyanobacteria as food
- •Cyanophages
- •Secretion of antibiotics and siderophores
- •Calcium carbonate deposition and fossil record
- •Chroococcales
- •Classification
- •Oscillatoriales
- •Nostocales
- •REFERENCES
- •REFERENCES
- •REFERENCES
- •RHODOPHYCEAE
- •Cell structure
- •Cell walls
- •Chloroplasts and storage products
- •Pit connections
- •Calcification
- •Secretory cells
- •Iridescence
- •Epiphytes and parasites
- •Defense mechanisms of the red algae
- •Commercial utilization of red algal mucilages
- •Reproductive structures
- •Carpogonium
- •Spermatium
- •Fertilization
- •Meiosporangia and meiospores
- •Asexual spores
- •Spore motility
- •Classification
- •Cyanidiales
- •Porphyridiales
- •Bangiales
- •Acrochaetiales
- •Batrachospermales
- •Nemaliales
- •Corallinales
- •Gelidiales
- •Gracilariales
- •Ceramiales
- •REFERENCES
- •Cell structure
- •Phototaxis and eyespots
- •Asexual reproduction
- •Sexual reproduction
- •Classification
- •Position of flagella in cells
- •Flagellar roots
- •Multilayered structure
- •Occurrence of scales or a wall on the motile cells
- •Cell division
- •Superoxide dismutase
- •Prasinophyceae
- •Charophyceae
- •Classification
- •Klebsormidiales
- •Zygnematales
- •Coleochaetales
- •Charales
- •Ulvophyceae
- •Classification
- •Ulotrichales
- •Ulvales
- •Cladophorales
- •Dasycladales
- •Caulerpales
- •Siphonocladales
- •Chlorophyceae
- •Classification
- •Volvocales
- •Tetrasporales
- •Prasiolales
- •Chlorellales
- •Trebouxiales
- •Sphaeropleales
- •Chlorosarcinales
- •Chaetophorales
- •Oedogoniales
- •REFERENCES
- •REFERENCES
- •EUGLENOPHYCEAE
- •Nucleus and nuclear division
- •Eyespot, paraflagellar swelling, and phototaxis
- •Muciferous bodies and extracellular structures
- •Chloroplasts and storage products
- •Nutrition
- •Classification
- •Heteronematales
- •Eutreptiales
- •Euglenales
- •REFERENCES
- •DINOPHYCEAE
- •Cell structure
- •Theca
- •Scales
- •Flagella
- •Pusule
- •Chloroplasts and pigments
- •Phototaxis and eyespots
- •Nucleus
- •Projectiles
- •Accumulation body
- •Resting spores or cysts or hypnospores and fossil Dinophyceae
- •Toxins
- •Dinoflagellates and oil and coal deposits
- •Bioluminescence
- •Rhythms
- •Heterotrophic dinoflagellates
- •Direct engulfment of prey
- •Peduncle feeding
- •Symbiotic dinoflagellates
- •Classification
- •Prorocentrales
- •Dinophysiales
- •Peridiniales
- •Gymnodiniales
- •REFERENCES
- •REFERENCES
- •Chlorarachniophyta
- •REFERENCES
- •CRYPTOPHYCEAE
- •Cell structure
- •Ecology
- •Symbiotic associations
- •Classification
- •Goniomonadales
- •Cryptomonadales
- •Chroomonadales
- •REFERENCES
- •CHRYSOPHYCEAE
- •Cell structure
- •Flagella and eyespot
- •Internal organelles
- •Extracellular deposits
- •Statospores
- •Nutrition
- •Ecology
- •Classification
- •Chromulinales
- •Parmales
- •Chrysomeridales
- •REFERENCES
- •SYNUROPHYCEAE
- •Classification
- •REFERENCES
- •EUSTIGMATOPHYCEAE
- •REFERENCES
- •PINGUIOPHYCEAE
- •REFERENCES
- •DICTYOCHOPHYCEAE
- •Classification
- •Rhizochromulinales
- •Pedinellales
- •Dictyocales
- •REFERENCES
- •PELAGOPHYCEAE
- •REFERENCES
- •BOLIDOPHYCEAE
- •REFERENCE
- •BACILLARIOPHYCEAE
- •Cell structure
- •Cell wall
- •Cell division and the formation of the new wall
- •Extracellular mucilage, biolfouling, and gliding
- •Motility
- •Plastids and storage products
- •Resting spores and resting cells
- •Auxospores
- •Rhythmic phenomena
- •Physiology
- •Chemical defense against predation
- •Ecology
- •Marine environment
- •Freshwater environment
- •Fossil diatoms
- •Classification
- •Biddulphiales
- •Bacillariales
- •REFERENCES
- •RAPHIDOPHYCEAE
- •REFERENCES
- •XANTHOPHYCEAE
- •Cell structure
- •Cell wall
- •Chloroplasts and food reserves
- •Asexual reproduction
- •Sexual reproduction
- •Mischococcales
- •Tribonematales
- •Botrydiales
- •Vaucheriales
- •REFERENCES
- •PHAEOTHAMNIOPHYCEAE
- •REFERENCES
- •PHAEOPHYCEAE
- •Cell structure
- •Cell walls
- •Flagella and eyespot
- •Chloroplasts and photosynthesis
- •Phlorotannins and physodes
- •Life history
- •Classification
- •Dictyotales
- •Sphacelariales
- •Cutleriales
- •Desmarestiales
- •Ectocarpales
- •Laminariales
- •Fucales
- •REFERENCES
- •PRYMNESIOPHYCEAE
- •Cell structure
- •Flagella
- •Haptonema
- •Chloroplasts
- •Other cytoplasmic structures
- •Scales and coccoliths
- •Toxins
- •Classification
- •Prymnesiales
- •Pavlovales
- •REFERENCES
- •Toxic algae
- •Toxic algae and the end-Permian extinction
- •Cooling of the Earth, cloud condensation nuclei, and DMSP
- •Chemical defense mechanisms of algae
- •The Antarctic and Southern Ocean
- •The grand experiment
- •Antarctic lakes as a model for life on the planet Mars or Jupiter’s moon Europa
- •Ultraviolet radiation, the ozone hole, and sunscreens produced by algae
- •Hydrogen fuel cells and hydrogen gas production by algae
- •REFERENCES
- •Glossary
- •Index
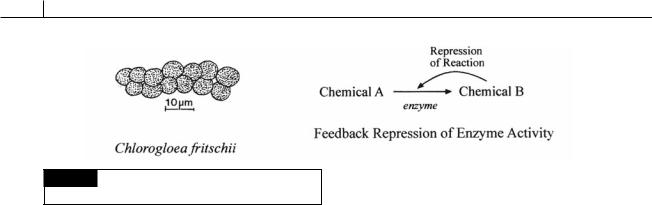
56 THE PROKARYOTIC ALGAE
Fig. 2.37 Cyanobacteria, such as Chlorogloea fritschii (left),
lack feedback inhibition of enzyme activity (right).
Lack of feedback control of enzyme biosynthesis
Cyanobacteria, such as Chlorogloea fritschii (Fig. 2.37), lack metabolic control of many pathways by repression and derepression of enzyme biosynthesis as occurs in many other organisms. In other organisms, as an end product of a pathway builds up to excess, the end product represses an enzyme involved in its synthesis, thereby directing precursors to other parts of the cell’s metabolism, where they can be better used. When the amount of end product falls, the enzyme is derepressed, allowing manufacture of the end product again.
Cyanobacteria often release considerable quantities of nitrogenous and organic substances into the medium, with most of the compounds being excreted as peptides (Fogg, 1942, 1952; Walsby, 1974). The secretion of nitrogenous substances represents a considerable waste of potential metabolites which have been formed from carbon dioxide with an expenditure of ATP and reducing potential (e.g., NADH). Such an excretion, however, could well be a necessary consequence of ill-controlled amino acid biosynthesis. Unable accurately to adjust the synthesis of each amino acid to the needs of protein synthesis, the cells would inevitably have to synthesize an excess of amino acids to ensure that protein synthesis would proceed smoothly. This excess would then form the basis of the extracellular peptide material associated with the cyanobacteria.
The general lack of repression and derepression of enzyme synthesis in the cyanobacteria implies that the algal metabolic pathways developed before the control systems responsible
for their regulation. The failure to develop transcriptional or regulatory control places the cyanobacteria under a selective disadvantage relative to other organisms possessing such metabolic regulators. They would be unable to divert biosynthetic capacity away from surplus synthesis to more necessary activity. Such a selective advantage would survive only in microorganisms less open to competition than most, or, in other words, in organisms in a particular ecological niche or in an environment with an abundant energy source. Autotrophic microorganisms such as the cyanobacteria fall into this category. Their source of energy (light) is at times more than sufficient, and in certain cases they occur in eccentric ecological situations that extremes of temperature or pH make relatively inhospitable.
Symbiosis
The cyanobacteria occur in basically two types of associations: those in which the cyanobacterium is extracellular and those in which it is intracellular.
Extracellular associations
Cyanobacteria in extracellular associations all show a decrease in growth, assimilation of CO2 and assimilation of NH4 (Meeks and Elhai, 2002). Conversely, there is an increase in the rate of N2 fixation that accompanies the increased numbers of heterocysts. The heterocysts and vegetative cells of Nostoc within leaf cavities of Azolla are up to fourfold larger than the vegetative cells of free-living cyanobacteria. In the symbiosis, Nostoc receives hexose sugars from the host, while Nostoc provides fixed nitrogen to the host. There is also a fiveto ten-fold increase in the production of
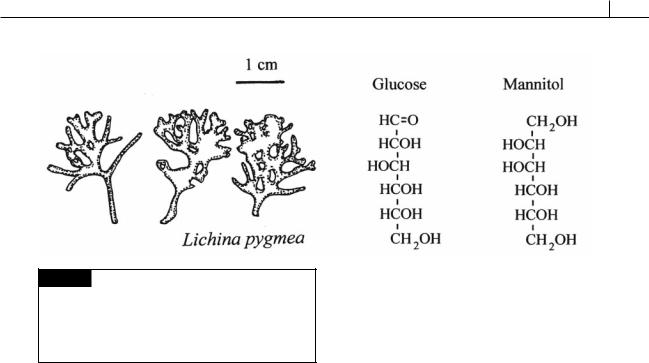
CYANOBACTERIA 57
Fig. 2.38 Lichina pygmea is a lichen containing a cyanobacteria as the phycobiont. Lichina occurs on rocks near the high-tide mark and looks like a stubby seaweed with its club-shaped branches making a sward of uniform height. The cyanobacterial phycobiont transfers glucose to the mycobiont which converts the glucose to mannitol.
motile hormogonia that serve as infective units in the establishment of the symbioses (Meeks, 1998).
The most common type of extracellular association is that with fungi to form lichens. In most lichens, there is a single algal component (phycobiont), which is a green alga, cyanobacterium, or, as in species of Verrucaria, a member of the Xanthophyceae. Cyanobacteria occur in about 8% of the species of lichens (Fig. 2.38). Almost all of the fungal partners (mycobionts) are ascomycetes, but certain imperfect fungi and basidiomycetes also occur in lichens (Ahmadjian and Hale, 1973). Because the mycobionts frequently reproduce sexually whereas the phycobionts usually do not, the lichens are classified with the fungi. In the symbiotic association, the phycobiont fixes carbon in photosynthesis and liberates it as glucose, which the mycobiont converts into mannitol and assimilates (Fig. 2.38). Approximately 40% of the photosynthate of the alga is secreted as glucose in the lichen association (Smith et al., 1970). Whereas the benefits to the fungus in the association are obvious, the benefits to the alga are probably limited to some protection against desiccation.
The water fern Azolla (Fig. 2.40(a), (b)) has cavities in the dorsal lobe of the leaf that are occupied by Anabaena azollae (Fig. 2.39(c), (d)). This cyanobacterium fixes nitrogen (Peters and Mayne, 1974), some of which is excreted into the cavity and taken up by the cells of the Azolla. It is possible to obtain alga-free Azolla plants by treating them with antibiotic or subjecting them to growth conditions that allow the fern to outgrow the alga. The Anabaena is apparently unable to live outside of the host. The heterocyst frequency (compared to vegetative cells) in A. azollae is about 30%, which is very high, the highest for free-living Anabaena being about 8% (Hill, 1975). The host Azolla somehow modifies the surface properties of the Anabaena. The alga in the fern cavity has different surface antigens, as compared to when it is growing free in culture (Gates et al., 1980). Nitrogen fixation by the cyanobacterial symbionts of Azolla has for many years been utilized to advantage in the Far East; here the water fern has been used as a green manure in rice fields, where about 3 kg of atmospheric nitrogen per hectare per day is fixed by Azolla symbionts (Swaminathan, 1984; Canini et al., 1992).
Cycad roots are frequently infected with cyanobacteria that cause distortions of the roots (coralloid roots) (Figs. 2.39(a), (b), 2.40(c)). In these roots the cyanobacteria occupy a clearly defined cortical zone midway between the pericycle and the epidermis. The cyanobacteria occur in the intercellular spaces and are surrounded by a
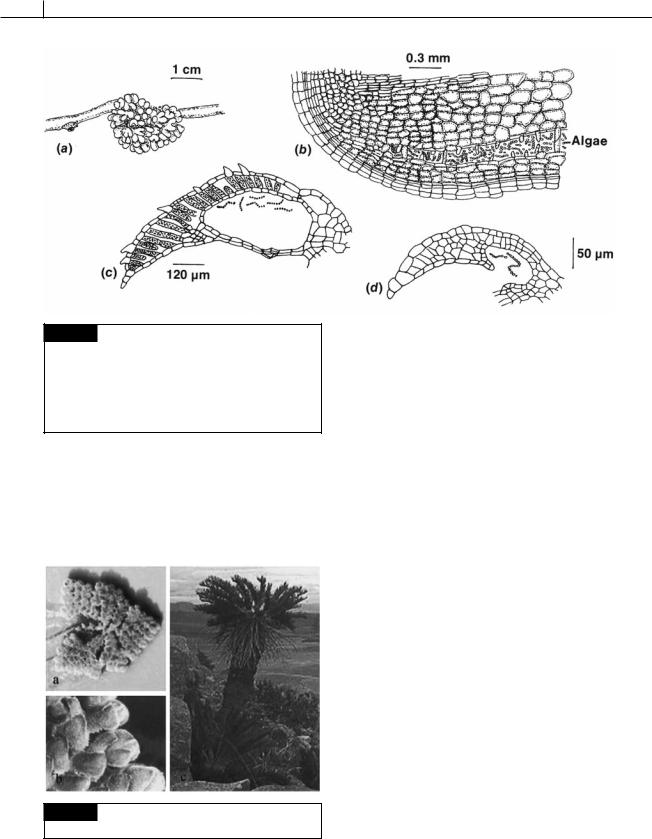
58 THE PROKARYOTIC ALGAE
Fig. 2.39 (a) Root nodules of the cycad Dioon spinulosum. (b) Part of a longitudinal section of a nodule of Encephalartos with an algal zone. Longitudinal sections through the
dorsal lobe of a mature (c) and an immature (d) leaf of the water fern Azolla illustrating filaments of Anabaena in leaf cavities. ((a),(b) after Spratt, 1915; (c),(d) after Smith, 1955.)
sheath and a multilayered wall, whereas the adjacent cycad cells have a reduced chromosome number and secrete slime (Storey, 1968; Caiola, 1975). These cyanobacteria are able to fix nitrogen and contribute a portion of the
Fig. 2.40 Plants that have symbiotic cyanobacteria.
(a,b) The water fern Azolla (c) The cycad Encephalartos.
fixed nitrogen to the cycad cells (Watanabe and Kiyohara, 1963).
Intracellular associations
Intracellular associations involving cyanobacteria are usually more specialized than extracellular associations, and it is not possible to culture the cyanobacteria away from the host. Pascher (1914) coined the term cyanelle for intracellular cyanobacteria in symbioses, and the term cyanome for the host cell. Cyanelles are present in a variety of cyanomes; such a variety that it is apparent that they are the result of a number of different symbiotic establishments and are not derived from a single event. It is probable that one of these endosymbiotic associations led to the development of chloroplasts in some of the algal groups. This line of evolution is considered under Glaucophyta (see beginning of Chapter 3).
Colonies of the coral Montastraea cavernosa contain endosymbiotic coccoid cyanobacteria in vacuoles in the epithelial cells of the coral (Lesser et al., 2004). The cyanobacteria are capable of nitrogen fixation. Anaerobic conditions (necessary for function of the nitrogen-fixing enzyme nitrogenase) are created by the Mehler reaction (Fig. 2.41) where glycerol provided by dinoflagellate zooxanthellae is reduced.