
- •Contents
- •Preface to the first edition
- •Flagella
- •Cell walls and mucilages
- •Plastids
- •Mitochondria and peroxisomes
- •Division of chloroplasts and mitochondria
- •Storage products
- •Contractile vacuoles
- •Nutrition
- •Gene sequencing and algal systematics
- •Classification
- •Algae and the fossil record
- •REFERENCES
- •CYANOPHYCEAE
- •Morphology
- •Cell wall and gliding
- •Pili and twitching
- •Sheaths
- •Protoplasmic structure
- •Gas vacuoles
- •Pigments and photosynthesis
- •Akinetes
- •Heterocysts
- •Nitrogen fixation
- •Asexual reproduction
- •Growth and metabolism
- •Lack of feedback control of enzyme biosynthesis
- •Symbiosis
- •Extracellular associations
- •Ecology of cyanobacteria
- •Freshwater environment
- •Terrestrial environment
- •Adaption to silting and salinity
- •Cyanotoxins
- •Cyanobacteria and the quality of drinking water
- •Utilization of cyanobacteria as food
- •Cyanophages
- •Secretion of antibiotics and siderophores
- •Calcium carbonate deposition and fossil record
- •Chroococcales
- •Classification
- •Oscillatoriales
- •Nostocales
- •REFERENCES
- •REFERENCES
- •REFERENCES
- •RHODOPHYCEAE
- •Cell structure
- •Cell walls
- •Chloroplasts and storage products
- •Pit connections
- •Calcification
- •Secretory cells
- •Iridescence
- •Epiphytes and parasites
- •Defense mechanisms of the red algae
- •Commercial utilization of red algal mucilages
- •Reproductive structures
- •Carpogonium
- •Spermatium
- •Fertilization
- •Meiosporangia and meiospores
- •Asexual spores
- •Spore motility
- •Classification
- •Cyanidiales
- •Porphyridiales
- •Bangiales
- •Acrochaetiales
- •Batrachospermales
- •Nemaliales
- •Corallinales
- •Gelidiales
- •Gracilariales
- •Ceramiales
- •REFERENCES
- •Cell structure
- •Phototaxis and eyespots
- •Asexual reproduction
- •Sexual reproduction
- •Classification
- •Position of flagella in cells
- •Flagellar roots
- •Multilayered structure
- •Occurrence of scales or a wall on the motile cells
- •Cell division
- •Superoxide dismutase
- •Prasinophyceae
- •Charophyceae
- •Classification
- •Klebsormidiales
- •Zygnematales
- •Coleochaetales
- •Charales
- •Ulvophyceae
- •Classification
- •Ulotrichales
- •Ulvales
- •Cladophorales
- •Dasycladales
- •Caulerpales
- •Siphonocladales
- •Chlorophyceae
- •Classification
- •Volvocales
- •Tetrasporales
- •Prasiolales
- •Chlorellales
- •Trebouxiales
- •Sphaeropleales
- •Chlorosarcinales
- •Chaetophorales
- •Oedogoniales
- •REFERENCES
- •REFERENCES
- •EUGLENOPHYCEAE
- •Nucleus and nuclear division
- •Eyespot, paraflagellar swelling, and phototaxis
- •Muciferous bodies and extracellular structures
- •Chloroplasts and storage products
- •Nutrition
- •Classification
- •Heteronematales
- •Eutreptiales
- •Euglenales
- •REFERENCES
- •DINOPHYCEAE
- •Cell structure
- •Theca
- •Scales
- •Flagella
- •Pusule
- •Chloroplasts and pigments
- •Phototaxis and eyespots
- •Nucleus
- •Projectiles
- •Accumulation body
- •Resting spores or cysts or hypnospores and fossil Dinophyceae
- •Toxins
- •Dinoflagellates and oil and coal deposits
- •Bioluminescence
- •Rhythms
- •Heterotrophic dinoflagellates
- •Direct engulfment of prey
- •Peduncle feeding
- •Symbiotic dinoflagellates
- •Classification
- •Prorocentrales
- •Dinophysiales
- •Peridiniales
- •Gymnodiniales
- •REFERENCES
- •REFERENCES
- •Chlorarachniophyta
- •REFERENCES
- •CRYPTOPHYCEAE
- •Cell structure
- •Ecology
- •Symbiotic associations
- •Classification
- •Goniomonadales
- •Cryptomonadales
- •Chroomonadales
- •REFERENCES
- •CHRYSOPHYCEAE
- •Cell structure
- •Flagella and eyespot
- •Internal organelles
- •Extracellular deposits
- •Statospores
- •Nutrition
- •Ecology
- •Classification
- •Chromulinales
- •Parmales
- •Chrysomeridales
- •REFERENCES
- •SYNUROPHYCEAE
- •Classification
- •REFERENCES
- •EUSTIGMATOPHYCEAE
- •REFERENCES
- •PINGUIOPHYCEAE
- •REFERENCES
- •DICTYOCHOPHYCEAE
- •Classification
- •Rhizochromulinales
- •Pedinellales
- •Dictyocales
- •REFERENCES
- •PELAGOPHYCEAE
- •REFERENCES
- •BOLIDOPHYCEAE
- •REFERENCE
- •BACILLARIOPHYCEAE
- •Cell structure
- •Cell wall
- •Cell division and the formation of the new wall
- •Extracellular mucilage, biolfouling, and gliding
- •Motility
- •Plastids and storage products
- •Resting spores and resting cells
- •Auxospores
- •Rhythmic phenomena
- •Physiology
- •Chemical defense against predation
- •Ecology
- •Marine environment
- •Freshwater environment
- •Fossil diatoms
- •Classification
- •Biddulphiales
- •Bacillariales
- •REFERENCES
- •RAPHIDOPHYCEAE
- •REFERENCES
- •XANTHOPHYCEAE
- •Cell structure
- •Cell wall
- •Chloroplasts and food reserves
- •Asexual reproduction
- •Sexual reproduction
- •Mischococcales
- •Tribonematales
- •Botrydiales
- •Vaucheriales
- •REFERENCES
- •PHAEOTHAMNIOPHYCEAE
- •REFERENCES
- •PHAEOPHYCEAE
- •Cell structure
- •Cell walls
- •Flagella and eyespot
- •Chloroplasts and photosynthesis
- •Phlorotannins and physodes
- •Life history
- •Classification
- •Dictyotales
- •Sphacelariales
- •Cutleriales
- •Desmarestiales
- •Ectocarpales
- •Laminariales
- •Fucales
- •REFERENCES
- •PRYMNESIOPHYCEAE
- •Cell structure
- •Flagella
- •Haptonema
- •Chloroplasts
- •Other cytoplasmic structures
- •Scales and coccoliths
- •Toxins
- •Classification
- •Prymnesiales
- •Pavlovales
- •REFERENCES
- •Toxic algae
- •Toxic algae and the end-Permian extinction
- •Cooling of the Earth, cloud condensation nuclei, and DMSP
- •Chemical defense mechanisms of algae
- •The Antarctic and Southern Ocean
- •The grand experiment
- •Antarctic lakes as a model for life on the planet Mars or Jupiter’s moon Europa
- •Ultraviolet radiation, the ozone hole, and sunscreens produced by algae
- •Hydrogen fuel cells and hydrogen gas production by algae
- •REFERENCES
- •Glossary
- •Index
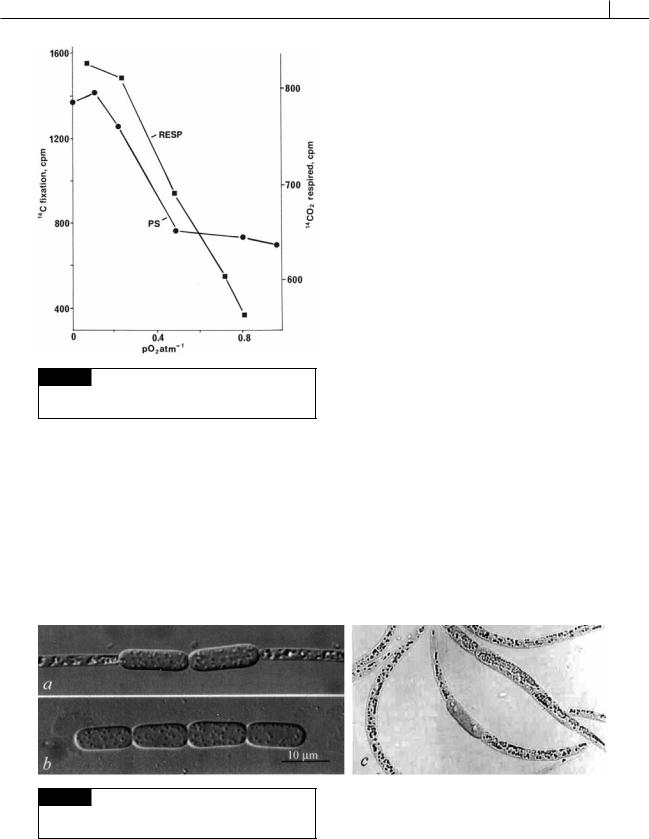
CYANOBACTERIA 45
Fig. 2.21 Graph showing stimulation of photosynthesis (●-●) and respiration (■-■) by low concentrations of atmospheric O2 in Anabaena flos-aquae. (After Stewart and Pearson, 1970.)
Akinetes
Akinetes are generally recognized by their larger size relative to vegetative cells and conspicuous granulation due to high concentrations of glycogen and cyanophycin (Figs. 2.22, 2.23) (Meeks et al., 2002). The most consistent property of akinetes is their greater resistance to cold compared with
vegetative cells. Akinetes have often been compared to endospores in Gram-positive bacteria. Akinetes, however, are neither as metabolically quiescent nor as resistant to various environmental extremes. Akinetes only occur in cyanobacteria that form heterocysts.
In Aphanizomenon (Fig. 2.18(b)) (Wildman et al., 1975), the development of akinetes from vegetative cells involves an increase in cell size, the gradual disappearance of gas vacuoles, and an increase in cytoplasmic density and number of ribosomes and cyanophycin granules. Akinetes of Nostoc lose 90% of their photosynthetic and respiratory capabilities, as compared with vegetative cells. The loss occurs even though there is little change in phycocyanin and chlorophyll, the main photosynthetic pigments (Chauvat et al., 1982). Mature akinetes are usually considerably larger than vegetative cells, contain protoplasm full of food reserves, and have a normal cell wall surrounded by a wide three-layered coat (Jensen and Clark, 1969; Cmiech et al., 1986) (Figs. 2.16, 2.18, 2.19(d), 2.22, 2.23). Loss of flotation by an increase in cytoplasmic density causes filaments with akinetes to sink and overwinter in bottom sediments. In akinete germination, there is a reverse of the above events (Fig. 2.24).
A wide range of physicochemical factors have been reported to stimulate akinete differentiation; for example, phosphate deficiency, low temperature, carbon limitation and reduction in the availability of light energy (Li et al., 1997; van Dok and Hart, 1995).
Fig. 2.22 Light micrographs of akinetes in Raphidiopsis mediterranea (a),(b) and R. curvata (c). ((a),(b) from Watanabe et al., 2003; (c) from Li et al., 2001a.)
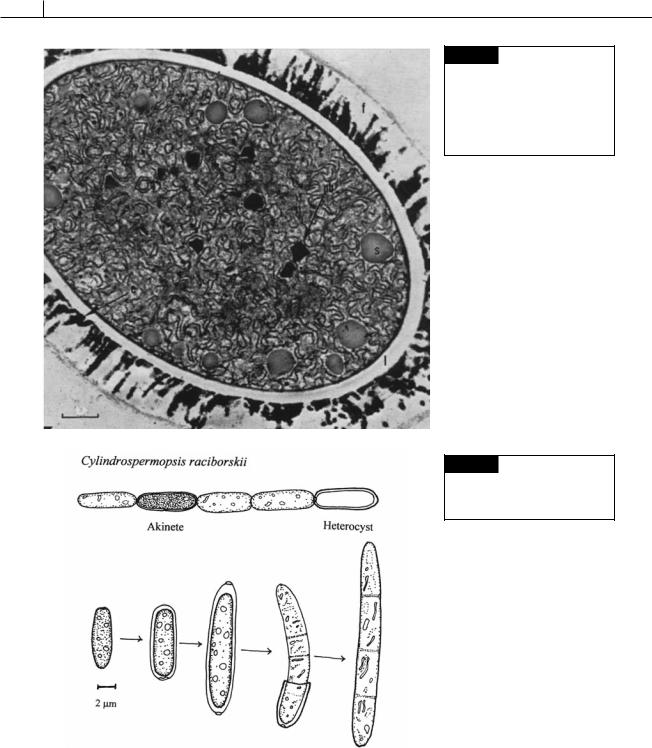
46 THE PROKARYOTIC ALGAE
Fig. 2.23 Electron micrograph of a mature akinete of Cylindrospermum sp. with a thick layered wall (f,l)
and cytoplasm full of proteinaceous cyanophycin (structured) granules (s), polyhedral bodies, and ribosomes. (From Clark and Jensen, 1969.)
Fig. 2.24 The germination of an akinete of Cylindrospermopsis raciborskii. (Modified from Moore et al., 2004.)
Heterocysts
Heterocysts are larger than vegetative cells and appear empty in the light microscope (whereas
akinetes appear full of storage products) (Figs. 2.16, 2.18(a), 2.19(d), 2.25, 2.35). Heterocysts are photosynthetically inactive, they do not fix CO2, nor do they produce O2. They also exhibit a high rate of respiratory O2 consumption and are
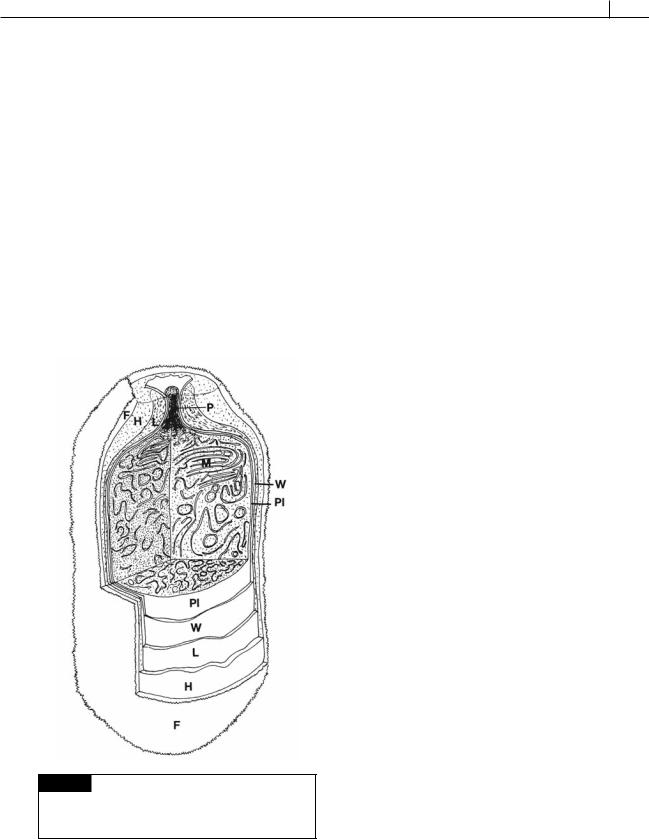
CYANOBACTERIA 47
surrounded by a thick, laminated cell wall that limits ingress of atmospheric gases, including O2. The internal environment of heterocysts is, therefore, virtually anoxic, which is ideal for nitrogenase, a notoriously O2 sensitive enzyme.
Heterocysts are formed at regular intervals from vegetative cells by the dissolution of storage granules, the deposition of a multilayered envelope outside of the cell wall, the breakdown of photosynthetic thylakoids, and the formation of new membranous structures (Fig. 2.25) (Kulasooriya et al., 1972).
The differentiation of heterocysts in Anabaena is triggered by nitrogen deprivation (ammonia, nitrate, nitrite) in two steps: the first step is reversible and the second step is irreversible (Adams, 2000; El-Shehawy and Kleiner, 2003).
Fig. 2.25 Three-dimensional view of a heterocyst. The envelope has homogeneous (H), fibrous (F), and laminated
(L) layers. (M) Membranes; (P) pore channel; (Pl) plasmalemma; (W) cell wall. (After Lang and Fay, 1971.)
Commitment point 1. 2-oxoglutarate ( -keto- glutarate) (Fig. 2.26) is the substrate used for incorporation of ammonium in cyanobacteria. The absence of combined nitrogen leads to an increase in intracellular 2-oxoglutarate since cyanobacteria lack 2-oxoglutarate dehydrogenase and the ability to breakdown 2-oxoglutarate. The increase in intracellular 2-oxoglutarate activates the protein NtcA and results in a drop in the amount of the calcium-binding protein CcbP, and a rise in Ca2 in the cells (Zhao et al., 2005). Heterocysts have 10 times more Ca2 than vegetative cells. The rise in Ca2 up-regulates the hetR gene that forms hetR, a serine-type protease, which induces the vegetative cell to change into a heterocyst. The hetR protein is considered the “master switch” in heterocyst development. The production of hetR protein constitutes commitment point 1. A lack of combined nitrogen over a period of about 10 hours results in continued production of hetR protein and the cell becoming a proheterocyst, which under the light microscope appears less granulated than vegetative cells but still lacks a thick cell wall. The hetR protein also induces the production of an oligopeptide called PatS. This oligopeptide diffuses to adjacent cells where it prohibits the formation of hetR protein in the cells and assures that the adjacent cells do not transform into proheterocysts. This sets up the spacing seen in Anabaena where heterocysts are formed equidistant from each other. Commitment point 1 is reversible. A proheterocyst will revert back to a vegetative cell if combined nitrogen is added to the medium, causing a switch “off” of the hetR gene and formation of hetR protein. Differentiation of a vegetative cell to a proheterocyst also involves loss of photosystem II activity, thus eliminating photosynthetic generation of O2 (which inhibits nitrogen fixation).
Commitment point 2. The second stage can not be reversed by the addition of combined nitrogen and comprises the transformation of a proheterocyst into a mature heterocyst. This involves the formation of a thick cell wall containing glycolipids and polysaccharides to reduce diffusion of O2. The nitrogen-fixing enzyme nitrogenase is activated when 11 kilobases are removed between repeat sequences flanking nitrogenase (nif ) genes.
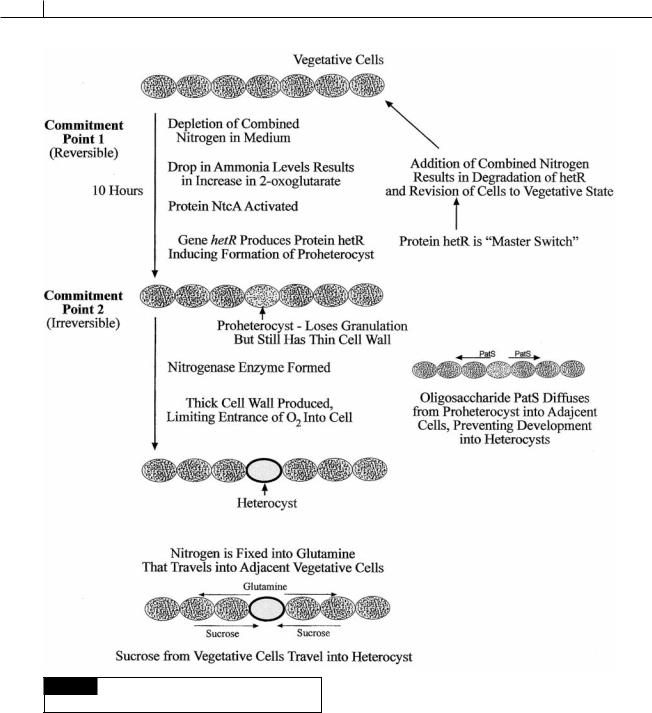
48 THE PROKARYOTIC ALGAE
Fig. 2.26 Summation of events leading to the formation of
heterocysts.
This process of heterocyst development is interesting in that it may represent one of the earliest examples of pattern formation in evolution. It is probable that akinetes were the evolutionary precursors in heterocysts (Meeks et al., 2002). Akinetes are only seen in heterocyst-forming
cyanobacteria, akinetes contain glycolipids characteristic of heterocysts, and the cell wall of heterocysts is identical to that of akinetes (and unlike that of vegetative cells). Heterocyst differentiation is a terminal event and is a basic form of programmed cell death or apotosis.
Apart from the exceptional cases of germination, heterocysts are unable to divide. Heterocysts