
- •Introduction
- •Increasing Demand for Wireless QoS
- •Technical Approach
- •Outline
- •The Indoor Radio Channel
- •Time Variations of Channel Characteristics
- •Orthogonal Frequency Division Multiplexing
- •The 5 GHz Band
- •Interference Calculation
- •Error Probability Analysis
- •Results and Discussion
- •IEEE 802.11
- •IEEE 802.11 Reference Model
- •IEEE 802.11 Architecture and Services
- •Architecture
- •Services
- •802.11a Frame Format
- •Medium Access Control
- •Distributed Coordination Function
- •Collision Avoidance
- •Post-Backoff
- •Recovery Procedure and Retransmissions
- •Fragmentation
- •Hidden Stations and RTS/CTS
- •Synchronization and Beacons
- •Point Coordination Function
- •Contention Free Period and Superframes
- •QoS Support with PCF
- •The 802.11 Standards
- •IEEE 802.11
- •IEEE 802.11a
- •IEEE 802.11b
- •IEEE 802.11c
- •IEEE 802.11d
- •IEEE 802.11e
- •IEEE 802.11f
- •IEEE 802.11g
- •IEEE 802.11h
- •IEEE 802.11i
- •Overview and Introduction
- •Naming Conventions
- •Enhancements of the Legacy 802.11 MAC Protocol
- •Transmission Opportunity
- •Beacon Protection
- •Direct Link
- •Fragmentation
- •Traffic Differentiation, Access Categories, and Priorities
- •EDCF Parameter Sets per AC
- •Minimum Contention Window as Parameter per Access Category
- •Maximum TXOP Duration as Parameter per Access Category
- •Collisions of Frames
- •Other EDCF Parameters per AC that are not Part of 802.11e
- •Retry Counters as Parameter per Access Category
- •Persistence Factor as Parameter per Access Category
- •Traffic Streams
- •Default EDCF Parameter Set per Draft 4.0, Table 20.1
- •Hybrid Coordination Function, Controlled Channel Access
- •Controlled Access Period
- •Improved Efficiency
- •Throughput Improvement: Contention Free Bursts
- •Throughput Improvement: Block Acknowledgement
- •Delay Improvement: Controlled Contention
- •Maximum Achievable Throughput
- •System Saturation Throughput
- •Modifications of Bianchi’s Legacy 802.11 Model
- •Throughput Evaluation for Different EDCF Parameter Sets
- •Lower Priority AC Saturation Throughput
- •Higher Priority AC Saturation Throughput
- •Share of Capacity per Access Category
- •Calculation of Access Priorities from the EDCF Parameters
- •Markov Chain Analysis
- •The Priority Vector
- •Results and Discussion
- •QoS Support with EDCF Contending with Legacy DCF
- •1 EDCF Backoff Entity Against 1 DCF Station
- •Discussion
- •Summary
- •1 EDCF Backoff Entity Against 8 DCF Stations
- •Discussion
- •Summary
- •8 EDCF Backoff Entities Against 8 DCF Stations
- •Discussion
- •Summary
- •Contention Free Bursts
- •Contention Free Bursts and Link Adaptation
- •Simulation Scenario: two Overlapping QBSSs
- •Throughput Results with CFBs
- •Throughput Results with Static PHY mode 1
- •Delay Results with CFBs
- •Conclusion
- •Radio Resource Capture
- •Radio Resource Capture by Hidden Stations
- •Solution
- •Mutual Synchronization across QBSSs and Slotting
- •Evaluation
- •Simulation Results and Discussion
- •Conclusion
- •Prioritized Channel Access in Coexistence Scenarios
- •Saturation Throughput in Coexistence Scenarios
- •MSDU Delivery Delay in Coexistence Scenarios
- •Scenario
- •Simulation Results and Discussion
- •Conclusions about the HCF Controlled Channel Access
- •Summary and Conclusion
- •ETSI BRAN HiperLAN/2
- •Reference Model (Service Model)
- •System Architecture
- •Medium Access Control
- •Interworking Control of ETSI BRAN HiperLAN/2 and IEEE 802.11
- •CCHC Medium Access Control
- •CCHC Scenario
- •CCHC and Legacy 802.11
- •CCHC Working Principle
- •CCHC Frame Structure
- •Requirements for QoS Support
- •Coexistence Control of ETSI BRAN HiperLAN/2 and IEEE 802.11
- •Conventional Solutions to Support Coexistence of WLANs
- •Coexistence as a Game Problem
- •The Game Model
- •Overview
- •The Single Stage Game (SSG) Competition Model
- •The Superframe as SSG
- •Action, Action Space A, Requirements vs. Demands
- •Abstract Representation of QoS
- •Utility
- •Preference and Behavior
- •Payoff, Response and Equilibrium
- •The Multi Stage Game (MSG) Competition Model
- •Estimating the Demands of the Opponent Player
- •Description of the Estimation Method
- •Evaluation
- •Application and Improvements
- •Concluding Remark
- •The Superframe as Single Stage Game
- •The Markov Chain P
- •Illustration and Transition Probabilities
- •Definition of Corresponding States and Transitions
- •Solution of P
- •Collisions of Resource Allocation Attempts
- •Transition Probabilities Expressed with the QoS Demands
- •Average State Durations Expressed with the QoS Demands
- •Result
- •Evaluation
- •Conclusion
- •Definition and Objective of the Nash Equilibrium
- •Bargaining Domain
- •Core Behaviors
- •Available Behaviors
- •Strategies in MSGs
- •Payoff Calculation in the MSGs, Discounting and Patience
- •Static Strategies
- •Definition of Static Resource Allocation Strategies
- •Experimental Results
- •Scenario
- •Discussion
- •Persistent Behavior
- •Rational Behavior
- •Cooperative Behavior
- •Conclusion
- •Dynamic Strategies
- •Cooperation and Punishment
- •Condition for Cooperation
- •Experimental Results
- •Conclusion
- •Conclusions
- •Problem and Selected Method
- •Summary of Results
- •Contributions of this Thesis
- •Further Development and Motivation
- •IEEE 802.11a/e Simulation Tool “WARP2”
- •Model of Offered Traffic and Requirements
- •Table of Symbols
- •List of Figures
- •List of Tables
- •Abbreviations
- •Bibliography
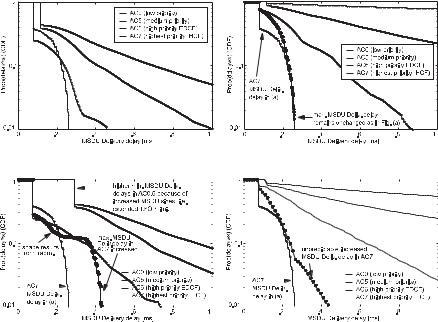
5.5 Summary and Conclusion
(a) isolated QBSS
115 |
(b) isolated QBSS with higher offered traffic
|
|
|
|
|
|
|
|
|
|
|
|
|
|
|
|
|
|
|
|
|
|
|
|
|
|
|
|
|
|
|
|
|
|
|
|
|
|
|
|
|
|
|
|
|
|
|
|
|
|
|
|
|
|
|
|
|
|
|
|
|
|
|
|
|
|
|
|
|
|
|
|
|
|
|
|
|
|
|
|
|
|
|
|
|
|
|
|
|
|
|
|
|
|
|
|
|
|
|
|
|
|
|
|
|
|
|
|
|
|
|
|
|
|
|
|
|
|
|
|
|
|
|
|
|
|
|
|
|
|
|
|
|
|
|
|
|
|
|
|
|
|
|
|
|
|
|
|
|
|
|
|
|
|
|
|
|
|
|
|
|
|
|
|
|
|
|
|
|
|
|
|
|
|
|
|
|
|
|
|
|
|
|
|
|
|
|
|
|
|
|
|
|
|
|
|
|
|
|
|
|
|
|
|
|
|
|
|
|
|
|
|
|
|
|
|
|
|
|
|
|
|
|
|
|
|
|
|
|
|
|
|
|
|
|
|
|
|
|
|
|
|
|
|
|
|
|
|
|
|
|
|
|
|
|
|
|
|
|
|
|
|
|
|
|
|
|
|
|
|
|
|
|
|
|
|
|
|
|
|
|
|
|
|
|
|
|
|
|
|
|
|
|
|
|
|
|
|
|
|
|
|
|
|
|
|
|
|
|
|
|
|
|
|
|
|
|
|
|
|
|
|
|
|
|
|
|
|
|
|
|
|
|
|
|
|
|
|
|
|
|
|
|
|
|
|
|
|
|
|
|
|
|
|
|
|
|
|
|
|
|
|
|
|
|
|
|
|
|
|
|
|
|
|
|
|
|
|
|
|
|
|
|
|
|
|
|
|
|
|
|
|
|
|
|
|
|
|
|
|
|
|
|
|
|
|
|
|
|
|
|
|
|
|
|
|
|
|
|
|
|
|
|
|
|
|
|
|
|
|
|
|
|
|
|
|
|
|
|
|
|
|
|
|
|
|
|
|
|
|
|
|
|
|
|
|
|
|
|
|
|
|
|
|
|
|
|
|
|
|
|
|
|
|
|
|
|
|
|
|
|
|
|
|
|
|
|
|
|
|
|
|
|
|
|
|
|
|
|
|
|
|
|
|
|
|
|
|
|
|
|
|
|
|
|
|
|
|
|
|
|
|
|
|
|
|
|
|
|
|
|
|
|
|
|
|
|
|
|
|
|
|
|
|
|
|
|
|
|
|
|
|
|
|
|
|
|
|
|
|
|
|
|
|
|
|
|
|
|
|
(c) isolated QBSS with longer EDCF-TXOPs |
|
|
|
|
|
|
|
(d) overlapping QBSSs |
Figure 5.40: (Mangold et al., 2002a) MSDU Delivery delay for the four ACs in isolated and overlapping scenarios.
5.4.4Conclusions about the HCF Controlled Channel Access
The controlled channel access of the HCF allows allocating resources with highest priority, and to guarantee a maximum MSDU Delivery delay only if the QBSS operates in isolation. If backoff entities of overlapping QBSSs allocate TXOPs that are longer than the specified TXOPlimit, the MSDU Delivery delay of the controlled channel access of the HCF increases unpredictably.
Further, if HCs of overlapping BSSs allocate CAPs as well, allocation attempts may often fail due to colliding frames, and MSDU Delivery delays may increase dramatically. As a result, QoS guarantees are not possible then.
5.5Summary and Conclusion
The upcoming 802.11e standard is an efficient means for QoS support in wireless LANs for a wide variety of applications, although open problems such as the overlapping QBSSs remain to be solved. This chapter provides an in-depth analy-
116 |
5. Evaluation of IEEE 802.11e with the IEEE 802.11a Physical Layer |
sis of potential 802.11e enhancements. The maximum achievable throughput as well as the saturation throughput for the HCF contention-based channel access (EDCF) have been calculated analytically, and validated by simulation, see Section 5.1.2.
A new model to approximate the resulting share of capacity per AC, i.e., the relative priority between different ACs when backoff entities operate with different EDCF parameters, has been developed and validated by simulation in Section 5.1.3. The model sufficiently approximates the simulation results for nearly all studied load scenarios.
The specific problem of providing EDCF backoff entities a higher priority in channel access over the legacy DCF stations has been discussed, and means based on the Persistence Factor and the EIFS to support EDCF in congestion with DCF were proposed and evaluated, see Section 5.1.4.
The importance of applying contention free bursts together with radio resource control schemes in coexistence scenarios have been highlighted in Section 5.2. Radio resource capture scenarios have been investigated in Section 5.3 and have been shown to be sensitive in that they might affect the ability of 802.11e to guarantee QoS support.
The controlled channel access of the HCF has been analyzed in Section 5.4. It provides in an isolated QBSS the means for delivering time-bounded traffic, and requires all backoff entities within the range of the HC to follow its coordination. QoS support appears to be problematic when multiple QBSSs overlap.

Chapter 6
COEXISTENCE AND INTERWORKING BETWEEN
802.11 AND HIPERLAN/2
6.1 ETSI BRAN HiperLAN/2................................................ |
118 |
6.2Interworking Control of ETSI BRAN HiperLAN/2
and IEEE 802.11................................................................. |
122 |
6.3 Coexistence ........................................................................ |
128 |
WIRELESS networks are able to coexist if they operate with the same radio resources at the same time and location, i.e., in an overlapping scenario (Mangold et al., 2001h). Wireless networks that coexist with
each other operate in the same coverage area without harmful interference. With successful coexistence, individual wireless networks that overlap with each other generally see a reduced capacity of the radio resources but still have full access to the available radio resources. Without a successful coexistence strategy, wireless networks are not able to successfully control their access to radio resources. It is worth mentioning that coexisting wireless networks not necessarily must follow the same standard. Further, the capability to exchange information between overlapping wireless networks is not a required characteristic for establishing successful coexistence, as will be shown in this chapter. Wireless networks are able to interwork rather than to coexist if they are able to exchange information across the different networks. When interworking, wireless networks are able to coordinate the usage of radio resources. This is achieved by exchanging spectrum coordination information. To be capable of exchanging information, it is required that overlapping wireless networks operate with the same transmission scheme through a protocol that is common between these wireless networks.