
Color Atlas of Physiology 2003 thieme
.pdf

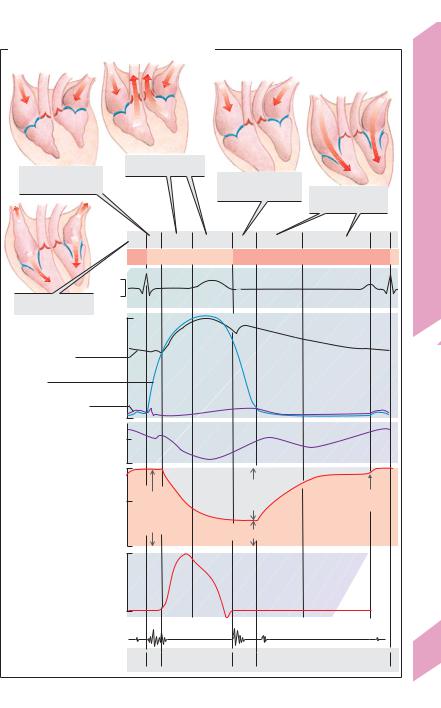

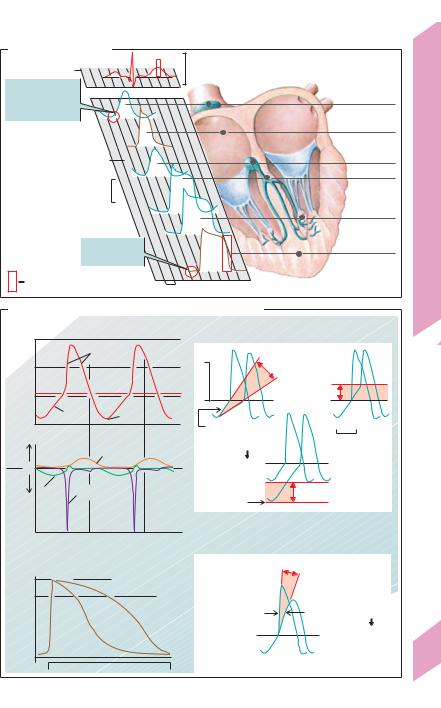



|
|
Electrocardiogram (ECG) |
the left side of the thorax in a nearly horizontal |
|
|
plane (!F). When used in combination with |
|
|
|
|
|
|
|
The ECG records potential differences (few |
the aforementioned leads in the frontal plane, |
|
|
m/V) caused by cardiac excitation. This pro- |
they provide a three-dimensional view of the |
|
|
vides information on heart position, relative |
integral vector. To make recordings with the |
|
|
chamber size, heart rhythm, impulse origin/ |
chest leads (different electrode), the three limb |
|
|
propagation and rhythm/conduction distur- |
leads are connected to form an indifferent elec- |
|
|
bances, extent and location of myocardial |
trode with high resistances (5 kΩ). The chest |
|
|
ischemia, changes in electrolyte concentra- |
leads mainly detect potential vectors directed |
|
|
tions, and drug effects on the heart. However, |
towards the back. These vectors are hardly de- |
|
|
it does not provide data on cardiac contraction |
tectable in the frontal plane. Since the mean |
|
|
or pumping function. |
QRS vector (see below) is usually directed |
System |
|
ECG potential differences arise at the inter- |
downwards and towards the left back region, |
|
face between stimulated and non-stimulated |
the QRS vectors recorded by leads V1–V3 are |
|
|
|
||
|
|
myocardium. Totally stimulated or unstimu- |
usually negative, while those detected by V5 |
Cardiovascular |
|
lated myocardial tissue does not generate any |
and V6 are positive. |
|
visible potentials The migration of the exci- |
segments, and intervals (!B and p. 195 C). By |
|
|
|
tatory front through the heart muscle gives |
Intraesophageal leads and additional leads positioned |
|
|
rise to numerous potentials that vary in mag- |
in the region of the right chest (Vr3–Vr6) and left back |
|
|
nitude and direction. |
(V7–V9) are useful in certain cases (!F2). |
|
|
An ECG depicts electrical activity as waves, |
|
|
|
These vectors can be depicted as arrows, where the |
|
|
|
|
|
8 |
|
length of the arrow represents the magnitude of the |
convention, upward deflection of the waves is |
|
potential and the direction of the arrow indicates the |
||
|
|
direction of the potential (arrowhead is +). As in a |
defined as positive (+), and downward deflec- |
|
|
force parallelogram, the integral vector (summa- |
tion as negative (!). The electrical activity as- |
|
|
tion vector) is the sum of the numerous individual |
sociated with atrial depolarization is defined |
|
|
||
|
|
vectors at that moment (!A, red arrow). |
as the P wave ("0.3 mV, "0.1 s). Repolariza- |
|
|
The magnitude and direction of the integral |
tion of the atria normally cannot be visualized |
|
|
vector change during the cardiac cycle, pro- |
on the ECG since it tends to be masked by the |
|
|
ducing the typical vector loop seen on a vector- |
QRS complex. The QRS complex ("0.1 s) con- |
|
|
cardiogram. (In A, the maximum or chief vec- |
sists of one, two or three components: Q wave |
|
|
tor is depicted by the arrow, called the “electri- |
(mV " 1/4 of R, "0.04 s), R wave and/or S wave |
|
|
cal axis” of the heart, see below). |
(R+S #0.6 mV). The potential of the mean QRS |
|
|
Limb and chest leads of the ECG make it |
vector is the sum of the amplitudes of the Q, R |
|
|
possible to visualize the course of the integral |
and S waves (taking their positive and negative |
|
|
vector over time, projected at the plane deter- |
polarities into account). The voltage of the |
|
|
mined by the leads (scalar ECG). Leads parallel |
mean QRS vector is higher (in most leads) than |
|
|
to the integral vector show full deflection (R |
that of the P wave because the muscle mass of |
|
|
wave !1–2 mV), while those perpendicular to |
the ventricles is much larger than that of the |
|
|
it show no deflection. Einthoven leads I, II, and |
atria. The R wave is defined as the first positive |
|
|
III are bipolar limb leads positioned in the fron- |
deflection of the QRS complex, which means |
|
|
tal plane. Lead I records potentials between the |
that R waves from different leads may not be |
|
|
left and right arm, lead II those between the |
synchronous. The QRS complex represents the |
|
|
right arm and left leg, and lead III those be- |
depolarization of the ventricles, and the T wave |
|
|
tween the left arm and left leg (!C1). |
represents their repolarization. Although op- |
|
|
Goldberger leads are unipolar augmented limb |
posing processes, the T wave usually points in |
|
|
leads in the frontal plane. One lead (right arm, |
the same direction as the R wave (+ in most |
|
|
aVR, left arm aVL, or left leg, aVF; !D2) acts as |
leads). This means that depolarization and re- |
|
|
the different electrode, while the other two |
polarization do not travel in the same direction |
196 |
|
limbs are connected and serve as the indiffer- |
(!p. 195 C, QRS and T: vector arrows point in |
|
ent (reference) electrode (!D1). Wilson leads |
the same direction despite reversed polarity |
|
|
|
(V1–V6) are unipolar chest leads positioned on |
during repolarization). The PQ (or PR) segment |
|
|
|
! |
Despopoulos, Color Atlas of Physiology © 2003 Thieme
All rights reserved. Usage subject to terms and conditions of license.

