
- •Introduction
- •Materials and methods
- •Animals
- •Anatomical model
- •Histology
- •Measurements of pupil dynamics
- •Focal length measurements in the lower lens-eye
- •Method 1: point source imaging by isolated lenses
- •Optical investigation of the upper lens-eye
- •Results
- •Lower lens-eye morphology
- •Optics of the lower lens-eye
- •Upper lens-eye morphology
- •Optics of the upper lens-eye
- •Pit and slit eyes
- •Discussion
- •References
560 |
J Comp Physiol A (2009) 195:557–569 |
|
|
160–217 lm) and a simple linear regression was performed to confirm a linear relationship between the two, such that the focal length could be normalised to a single lens diameter. The average focal length was then calculated for a lens of 210 lm diameter, which was the average in fully grown animals. Measurements were performed on five fresh lenses from five large animals.
Method 2: image magnification behind isolated lenses
Using the same preparation as used in method 1, a grating replaced the pinhole used in method 1. At the position of the lens, one grating cycle subtended 2.46L. The focus was then adjusted to find the position where the grating was best focused. Image magnification was then used to calculate the focal length, f as f = x/tan 2.46, where x is the cycle width of the grating measured in the image plane. The same linear regression and transformation as described in method 1 was performed to get an average focal length for a lens with a diameter of 210 lm.
Method 3: image magnification of the lenses in situ
Long pigment cells containing white pigment are clearly visible through the lens of the lower eye in intact, fresh rhopalia. With the lens in place, the retina is magnified to a degree that depends on the focal length of the lens, and the distance from the lens centre (principal plane) to the observed retinal structures. The image magnification can be determined by comparing the distance between long pigment cells measured with and without the lens present. The white pigment extends from the base of the retina to about halfway towards the back of the lens, which, according to our geometrical model, corresponds to a distance of 118– 136 lm behind the lens centre. Assuming a distant observer, a relationship between the focal length, f, the distance between lens centre and visible objects in the retina, z, and image magnification is given by the geometry: f ¼ zdL=ðdL dN Þ, where dN is the actual separation of white pigmented cells and dL is the apparent separation observed through the lens. Estimation of lens magnification and the corresponding focal length was performed on four different preparations.
Optical investigation of the upper lens-eye
The lens of the upper lens-eye was too soft to be removed undamaged from the eye. Because the lens is almost without focusing power, the optics of the eye was investigated by observations of retinal illumination patterns, seen through the lens aperture, when the eye was illuminated by a point source (end of 2 mm light guide at 200 mm distance) moved to different positions in the visual field.
Results
Like other cubomedusa, the visual system of C. bronzie consists of four morphologically different eye types: two camera-type eyes (the upper lens-eye and lower lens-eye) and two pairs of pigment pit eyes (the pit and slit eyes) (Fig. 2a). The eyes are organised on sensory clubs known as rhopalia. Each rhopalium lies in a niche within the exumbrella, mid-way between pedalia and is attached to the subumbrella via a flexible stalk. Each medusa has four rhopalia, and on each rhopalium there are six eyes (one upper lens-eye, one lower lens-eye, two pit eyes and two slit eyes) making a total of 24 eyes. Both lens eyes are located on the midline of the rhopalium, whereas the pairs of pit and slit eyes are located symmetrically on either side of the rhopalium midline (Fig. 2a). All eyes of C. bronzie are everse and have ciliary photoreceptors. The rhopalia of C. bronzie are approximately 650–750 lm in length, which is much larger than the rhopalia of T. cystophora (Fig. 1). The gastric cavity enters the rhopalia via the stalk and occupies a significant portion of the centre of the rhopalium (Fig. 1a).
Lower lens-eye morphology
The lower lens-eye is the largest of the eye types with an average diameter of 360 lm in fully grown medusae. The components of the lower lens-eye include those typical for a camera-type eye: a cornea, a lens, a pigment layer, an adjustable iris, and a retina (Fig. 1). The cornea is a thin layer of epithelial cells that covers the lens and is continuous with the rhopalial epithelium (Fig. 1a). The lens of the lower lens-eye in C. bronzie is not spherical like that of T. cystophora, but flattened such that its depth is about three-fourth of its width (Figs. 1, 2b, c). The lens is cellular and the centre of the lens is consistently stained darker than the periphery, suggesting that the lens has a graded refractive index (Garm et al. 2008). The lens cells have their nuclei in columnar segments at the posterior periphery of the lens (Fig. 3d–g).
The retina lies close to the lens, separated only by a thin, approximately 8 lm, acellular, vitreous space. The retina contains two morphologically distinct cell types: ciliary photoreceptors and long pigment cells. Both cell types have three distinct segments, giving rise to three retinal layers: a thick layer of receptor-cell cilia and cone-shaped projections from the long pigment cells, here termed the ciliary layer; a thin pigment layer where the receptor cells are densely pigmented; and proximally, a neural layer containing nucleated cell bodies of the receptor cells and the long pigment cells (Fig. 3d–g). The neural layer contains synapses between receptor cells and presumably also with second order interneurons.
123

J Comp Physiol A (2009) 195:557–569 |
561 |
|
|
Fig. 3 The lower lens-eye of Chiropsella bronize. a Tangential c section of the lower lens-eye retina showing long pigment cells interspersed between the ciliary trunks of receptor cells. Scale bar 10 lm. b Transmission electron micrograph of the sagittal plane, through the pigment layer, showing long pigment cells penetrating the pigment layer where the receptor cells are densely pigmented. The white pigment particles are shaped like crystals, whereas the dark pigment is contained in spherical granules. Scale bar 5 lm. c Darkadapted fresh eye where the retina and its array of long pigment cells can be seen through the lens. Scale bar 100 lm. Inset is a magnification demonstrating that white pigment surrounds a dark core. d Bright-field microscopy of a sagittal section of a light-adapted retina, showing that the dark pigment extends into the ciliary layer, and that the long pigmented cells extend to the vitreous space. e Same section as d but viewed with polarised microscopy to reveal the location of the white pigment. f Bright-field microscopy of a darkadapted retina, demonstrated the migration of the dark pigment from the ciliary layer to the neural layer. g Same section as f but viewed with polarised light microscopy, showing that the white pigment remains in approximately the same positions irrespective of adaptational state. Scale bar d–g 10 lm. CL ciliary layer, PL pigment layer, NL neural layer, LP long pigment cell, CT ciliary trunk of receptor cell, M microvilli from receptor-cell cilium, WP white pigment, DP dark pigment, VS vitreous space
At the retinal margin towards the pupil, there is a gradual transition from pigmented receptor cells to pure pigment cells lacking the ciliary segment. The outermost of these pigment cells form the mobile iris. Under bright white light, the iris can constrict to an average of 77 lm (Fig. 4a). In the dark, the iris gradually dilates. After 15 min of dark adaptation, the pupil diameter increased to about 130 lm (Fig. 4b). When the eye was left in the dark for over an hour, the pupil diameter grew to approximately 270 lm (Fig. 4c). When the pupil was fully dilated, the width of the iris was larger than the lens diameter by approximately 25%. For optical calculations, we take the closed pupil to be 77 lm, and the open pupil to fit the lens diameter of 210 lm.
The ciliary layer is dominated by the ciliary segments of the receptor cells. The cilia extend from the pigment layer to the vitreous space. As seen in both light and electron microscopy, the ciliary trunks appear swollen at numerous places throughout the ciliary layer (Fig. 3a, d–g). From the ciliary membrane, microvilli extend, partly as bundles of parallel microvilli and partly as a disorganised tangle. The microvilli make up the majority of the volume of the ciliary layer. Scattered among the receptor cells are the long pigment cells. In the ciliary layer these pigment cells form cone-shaped segments and are filled with screening pigment (Fig. 3). These pigmented cones run parallel to the ciliary trunks of the sensory cells (Fig. 3d–g). Transmission electron micrographs could not identify any ciliary or microvillar projections from the long pigment cells, and therefore we assume that they have no photoreceptive function. Just above the pigment layer, the pigment cones
have a diameter of 6–10 lm (Fig. 3b), tapering to about a third of this width at the distal retinal surface.
Interestingly, the long pigment cells of C. bronzie contain two different screening pigments, a dark pigment, similar to that of the receptor cells, and a white pigment (Fig. 3), which has not been described before in cubozoans.
123
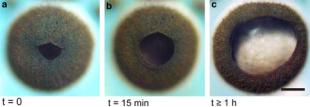
562 |
J Comp Physiol A (2009) 195:557–569 |
|
|
Fig. 4 Constriction and dilation of the pupil of the lower lens-eye of Chiropsella bronzie under different light adaptation states. a A fully light-adapted eye with constricted pupil, b after 15 min of dark adaptation and c after C1 h in total darkness. Scale bar 100 lm
This unusual pigmentation is noticeable in fresh eyes with a dilated pupil, where an irregular mosaic of white pegs with a dark centre can be seen throughout the retina (Fig. 3c). The long pigment cells do not contribute as much to the pigmentation of the pigment layer. The pigment layer is primarily a result of dense pigmentation in the receptor cells (Fig. 3b). In the neural layer, the long pigment cells have their cell bodies with nuclei, and here they are also filled with both dark and white pigment. The white pigment extends more proximally in the cells, making the back of the eye white. Seen from the front, this white pigmentation makes a sharp border where the dark pigment layer becomes the iris (Fig. 2a).
With bright-field light microscopy, dark pigment is easily seen, but the white pigment is hard to detect and may be mistaken as dark pigment (Fig. 3d, f). However, in polarisation microscopy, the white pigment becomes extremely obvious because it is the only structure that alters the plane of polarisation (Fig. 3e, g). Using this technique, the white pigment can be seen in the ciliary layer as well as in the neural layer (Fig. 3e, g). Light microscopy and TEM confirms that both pigment types occur together in the long pigment cells (Fig. 3b). In TEM the dark and white pigment granules are clearly different. The dark pigment granules appear electron dense, rounded, and vary only slightly in size, whereas the white pigment granules appear empty and have irregular, crystal-like shapes (Fig. 3b). In the ciliary layer, much of the white pigment occupies positions close to the cell membrane of the long pigment cells, such that it largely conceals the content of dark pigment in this part of the cells (Fig. 3b).
Sections of light adapted (Fig. 3d, e) and dark adapted (Fig. 3f, g) retinas, revealed that pigment migration occurs in the long pigment cells under different light conditions. This migration, however, is limited to the dark pigment. In a light-adapted retina, both dark and white pigment is seen in the ciliary layer. However, in a dark-adapted retina, dark pigment migrates to the neural layer and is almost absent from the ciliary layer, while the white pigment remains in both layers at all times (Fig. 3f, g).
Optics of the lower lens-eye
The focal length of the lens was measured in two different ways on fresh excised lenses, and compared to in situ measurements in fresh intact rhopalia. The isolated fresh lenses clearly had the ability to focus images of distant objects. Using a point source, and determining the distance to the narrowest focus, the average focal length was found to be 470 ± 5 lm when normalised to a lens diameter of 210 lm. The normalised focal length was thus about 4.5 lens radii. By using a stripe grating, and measuring the image magnification when focusing at the plane of highest
image contrast, the focal length |
was |
calculated to |
be |
462 ± 12 lm, again normalised |
to a |
lens diameter |
of |
210 lm. The contrast of the 2.46L cycle gratings at the focal length was low, approximately 0.9%, indicating that the 2.46L cycles is very close to the cut-off frequency of the lens. This in turn indicates that the lens suffers from significant aberrations.
Because the array of long pigment cells was clearly visible through a dilated pupil in an intact fresh rhopalium, there is a possibility to calculate the focal length by comparing the separation of long pigment cells seen through the lens in the intact rhopalium, with the same separation measured after the lens has been removed. With this principle, focal lengths ranging from 406 to 615 lm were calculated from different preparations. The wide range is a result of inaccuracies, which arise from difficulties in identifying the same location on the retina when the lens is present and absent. Another shortcoming of this technique is that the long pigment cells extend throughout much of the depth of the ciliary layer, and not in a single plane. Despite these shortcomings, the method has advantages because measurements are made on perfectly intact lenses. Thus, measurements with this technique confirm the results on excised lenses, although the accuracy cannot compete with that obtained from excised lenses.
Irrespective of the method used, all the measured focal lengths fall well beyond the retina in the lower lens-eye (Fig. 5a). The degree to which the lower lens-eye is underfocused can be directly determined by sending parallel light into an isolated lens (distant point source), and measuring the light distribution under the lens, at the levels occupied by the retina in the intact eye (Fig. 5c). Such experiments reveal that the originally parallel beam converges in a non-linear manner, where convergence is steeper close to the lens (Fig. 5a). This non-linear variation of the beam profile is inconsistent with aberration-free imaging, but is an expected consequence of positive spherical aberration (peripheral rays have comparatively shorter focal lengths).
Even though aberrations are substantial, they will not have a negative impact on the eye’s spatial resolution,
123
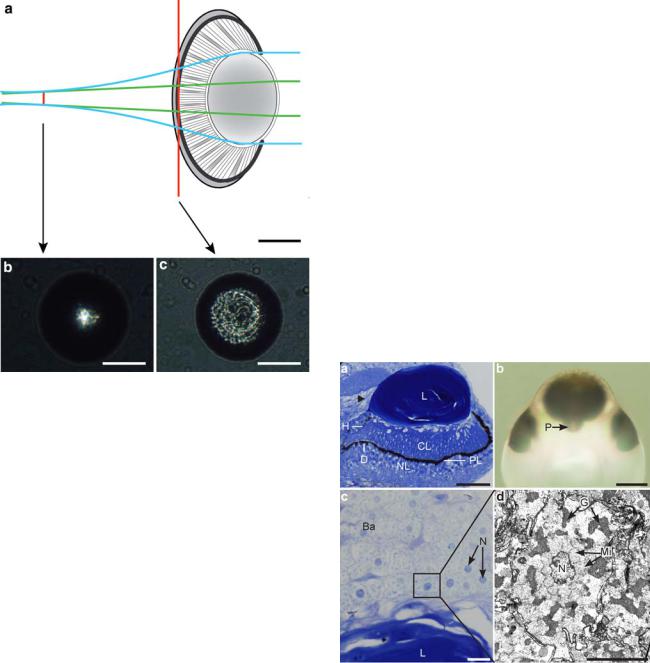
J Comp Physiol A (2009) 195:557–569 |
563 |
|
|
still a very large angle, which effectively removes all finer detail in the retinal image.
Upper lens-eye morphology
The upper lens-eye consists of the same basic components as those of the lower lens-eye. However, the geometry and structural details of the upper lens-eye differ significantly from the lower lens-eye. The upper lens-eye is smaller than the lower lens-eye and has an outer diameter of approximately 225 lm (Fig. 2a).
The lens of the upper lens-eye is an ellipsoid where all three diameters are different. Looking into the eye along the optical axis, the lens is 200 lm 9 130 lm in mature animals and the thickness is approximately 100 lm (Fig. 6a, b). This lens shape is similar to that seen in the species Tamoya bursaria (Yamasu and Yoshida 1976) but differs from the spherical lens of T. cystophora (Fig. 1). A cataract-like inclusion was observed in the lens core of the upper lens-eye (Fig. 7f). This scattering structure was
Fig. 5 The severely under-focused optics of the lower lens-eye of Chiropsella bronzie. a A model of the eye showing the beam width (at 50% level) when parallel light enters the eye through a dilated pupil (blue beam width) and a constricted pupil (green beam width). The beam converges behind the lens, and reaches a minimum width at about 300 lm behind the back of the retina. The non-linear reduction of beam width is due to positive spherical aberration. Beam widths were measured by recording the light distribution behind isolated lenses illuminated by parallel light of 500 nm wave length. Examples of recorded beam profiles are given for the plane of the pigment layer (c), and for the plane of best focus (b). The lens does not bring light to a perfect focus at any distance, as indicated by the broad blur-spot at the plane of best focus (b). Scale bars 100 lm
because the best focus is very far behind the retina. Instead, it is this under-focusing that will make the spatial resolution extremely poor. In order to estimate the spatial resolution of the eye, we used the measured beam widths recorded at midretinal depth behind isolated lenses. The angle that this beam width subtends at a point in the centre of the pupil plane was taken as an approximate half-width of the angular sensitivity function for an eye with a dilated pupil. This angle is 52L, implying a spatial resolution sufficient only for detection of very large structures at close range. With a constricted pupil the peripheral rays with short focal lengths are blocked, leaving only central rays, and these will have a much longer focal length, at least as long as the distance producing the minimum beam width (470 lm). With this assumption, the beam width at mid-retinal level for a constricted pupil is given by simple geometry (Fig. 5a), and the angle it subtends at the nodal point (pupil centre) works out to be 20L. This is
Fig. 6 The upper lens-eye of Chiropsella bronzie. a A sagittal section shows the different parts of the eye: the lens (L), the retina with a ciliary layer (CL), a pigment layer (PL) and a neural layer (NL). At the posterior side of the rhopalium (left in a) the pigment layer makes a dip (D) leaving a large unshielded hole (H) at the side of the retina. Above this hole, the gastric epithelium contacts the side of the lens (arrowhead). Scale bar 50 lm. b Viewing the upper lenseye from above in transmitted light, a protrusion (P) is seen in the retina at the posterior side of the rhopalium. This protrusion corresponds to the dip seen in sagittal sections. Scale bar 100 lm. c The balloon cells (Ba) just outside the lens (L), are roughly circular, have large nuclei (N), and a cytoplasm with granular content Scale bar 10 lm. The box in c corresponds to d, which is an electron micrograph of a balloon cell with its nucleus (N), mitochondria (Mi) and irregular granules (G). Scale bar 5 lm
123