
3728
.pdf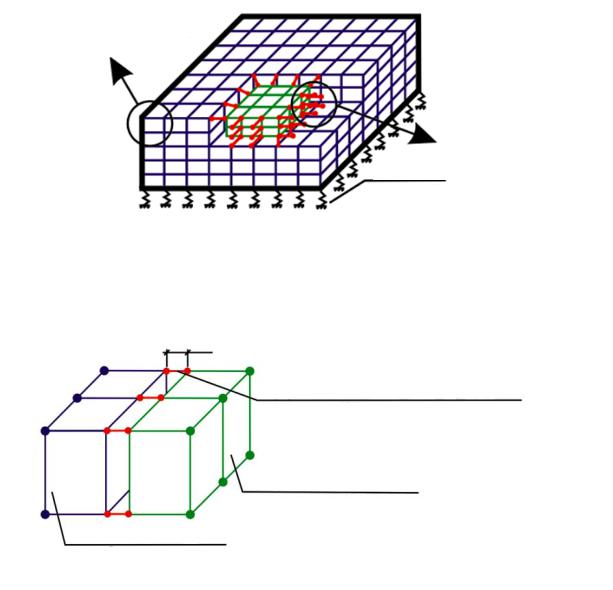
Issue № 4 (40), 2018 |
ISSN 2542-0526 |
Volumetric finite elements model the body of the reconstructed structure of the airfield surfacing. In order to optimize the calculation time without compromising the quality of the obtained result, the sizes of the volumetric elements are accepted to be no more than 10 сm, along the height 5 сm.
The use of two-node finite elements of one-sided connections with a specific effort allows a dispersion model of adhesion between the two materials to be implemented (Fig. 4). The above finite element is used to consider one-sided connections between the two nodes. In each node there are six degrees of freedom identified in relation to a local coordinate system. Therefore, the elements allows the linear as well as angular compliance of the connection to be modeled.
Volumetric finite element of the concrete plate
Two-node finite element with a one-sided connection with a specific effort
Elastic foundation
Fig. 3. Finite element scheme of the reconstructed airfield surfacing
Two-node finite element of a one-sided connection with a specific effort
1 mm
Two-node finite element of a one-sided connection with a specific effort
Finite element
of the repairing material
Volumetric finite element of the plate concrete
Fig. 4. Discrete model of adhesion of the repaired material to the plate concrete
91
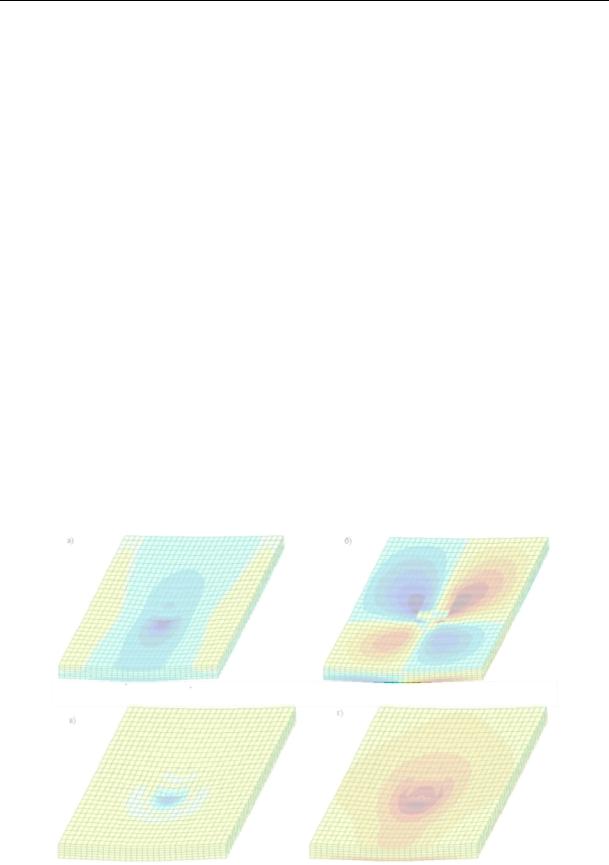
Russian Journal of Building Construction and Architecture
The use of two-node finite elements operating as one-sided connections transfers the structure into a structurally non-linear system. For such systems changes in a calculation scheme are common as a structure undergoes deformation. The rigidity and specific effort are defined only with the properties of a repairing composition. For the element being used, it is necessary that an initial and final nodes do not have identical coordinates. Therefore there is a 1 mm gap between concrete and a repairing composition.
Using a stepwise non-linear processor, the Lira software solves a linearized system of equations in each step for a current vector of node loads that is formed for a particular load.
As a result of the calculations, we get isofields that allow the assessment of redistribution of stress in the elements of the structure (Fig. 5), numerical values of the deflections, normal, main and largest tangential stresses to any point of the structure.
3. Comparative analysis of the results of analytical and numerical calculations of the stress strain of the repaired airfield surfacing. A comparative analysis of the above calculation methods will be performed using the results of a series of numerical experiments. The calculated structure is a repaired airfield plate sized 300 × 300 сm from concrete B25 with the elasticity modulus Е2= 3 × 104 МPа, thickness of 25 сm lying on a limestone foundation from a natural stone with the subbase coefficient K = 45 kg/сm3. The airfield surfacing perceives a static load of 10 kgs/сm2from the wheel of an aircraft.
a) |
|
b) |
|
|
|
|
|
d) |
|
c) |
|||
|
|
||
|
|
|
Fig. 5. Isofields of the stress of the reconstructed structure: а) normal σх; b) tangential τxy; c) main σ; d) largest tangential τmax
92
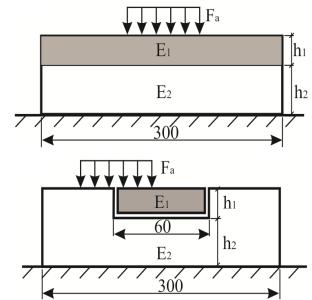
Issue № 4 (40), 2018 |
ISSN 2542-0526 |
The plate is calculated by means of an analytical method and it is reconstructed along its entire area, the thickness of the repairing layer is 3.5and 7 сm, a load is applied to the centre of the reconstructed structure of the airfield surfacing (Fig. 6а). A numerical method is used to calculate the plate in the centre of the plate of a repairing insertion with the following sizes: 3.5×60×60сm, 3.5×300×300сm, 7×60×60сm, 7×300×300сm — a load is applied at the boundaryofthematerials(Fig.6b).
a)
b)
Fig. 6. Structure of the reconstructed surfacing for numerical experiments:
а) restoration of the structure along the entire area of the plate; b) repairing insertion sized 60 × 60 сm
Depending on the depth of a defected area, a plate is repaired using the following materials: at h1=3.5 сm a polymer repairing material ХРМ-2; at h1 = 7 сm a repairing material based on the mineral binder MasterEmaco S466 [6]. The physical and mechanical parameters of the repairing materials and original data for calculations are presented in Table 2.
The values of normal stress in the repairing layer σ1 and at the boundary of the layers in the plate concrete σ2 obtained as a result of numerical experiments are presented in Table 3.
A general analysis suggests that the strength condition is met, the surfacing is reliable, the repairing material of the reconstructed structure is in the compressed area, an increase in the size of the repairing insertion in the plane causes an insignificant decrease in the compressive stress, an increase in the thickness of the repaired area leads to a decrease in the stress that depends on the physical and mechanical characteristics of the used repairing materials.
93

Russian Journal of Building Construction and Architecture
Таble 2 Geometric and physical and mechanical parameters of the layers of the reconstructed airfield surfacing
|
|
|
|
|
|
Analytical calculation method |
|
|
|
Numerical method in the Lira software |
|
||||||||||||||
|
|
|
|
|
|
|
|
|
|
|
|
|
|
|
|
|
|
|
|
|
|
|
|
||
|
|
|
|
|
|
|
|
|
Repairing material |
|
|
|
|
|
|
Characteristics |
|
||||||||
|
|
|
|
|
|
|
|
|
|
|
|
|
|
of repairing materials |
|
||||||||||
Parameter |
|
Airfield plate |
|
|
|
|
|
|
|
|
|
|
Airfield plate |
|
|
||||||||||
|
|
|
|
|
|
|
|
|
|
|
|
|
|
|
|
|
|
|
|||||||
|
|
|
|
|
(concrete В 25) |
|
|
|
|
|
Master |
|
(concrete В 25) |
|
|
|
|
Master |
|
||||||
|
|
|
|
|
|
ХРМ-2 |
|
|
|
|
ХРМ-2 |
|
Emaco |
|
|||||||||||
|
|
|
|
|
|
|
|
|
|
|
Emaco S466 |
|
|
|
|
|
|
|
|
S466 |
|
||||
|
|
|
|
|
|
|
|
|
|
|
|
|
|
|
|
|
|
|
|
|
|
|
|
||
|
|
|
|
|
|
|
|
|
|
|
|
|
|
|
|
|
|
|
|
|
|
|
|
||
Elasticity |
|
30000 |
|
27000 |
|
|
25000 |
|
|
30000 |
|
27000 |
|
|
25000 |
|
|||||||||
modulus, МPа |
|
|
|
|
|
|
|
|
|
|
|||||||||||||||
|
|
|
|
|
|
|
|
|
|
|
|
|
|
|
|
|
|
|
|
|
|||||
|
|
|
|
|
|
|
|
|
|
|
|
|
|
|
|
|
|
|
|
|
|
|
|
||
Strength |
|
|
|
|
|
|
|
|
|
|
|
|
|
|
|
|
|
|
|
|
|
||||
of adhesion with |
|
|
|
3.3 |
|
|
2.5 |
|
|
|
|
|
|
3.3 |
|
|
2.5 |
|
|||||||
concrete, МPа |
|
|
|
|
|
|
|
|
|
|
|
|
|
|
|
|
|
|
|
|
|
||||
|
|
|
|
|
|
|
|
|
|
|
|
|
|
|
|
|
|
|
|
|
|
|
|
||
Poissoncoefficient |
|
0.2 |
|
0.2 |
|
|
0.2 |
|
|
|
0.2 |
|
0.2 |
|
|
0.2 |
|
||||||||
|
|
|
|
|
|
|
|
|
|
|
|
|
|
|
|
|
|
|
|
|
|
|
|
||
Тhickness, сm |
|
25 |
|
3.5 |
|
|
7 |
|
|
|
25 |
|
3.5 |
|
|
7 |
|
||||||||
|
|
|
|
|
|
|
|
|
|
|
|
|
|
|
|
|
|
|
|
|
|
|
|
||
Compressive |
|
|
|
|
|
|
|
|
|
|
|
|
|
|
|
|
|
|
|
|
|
||||
strength limit R, |
|
32 |
|
36 |
|
|
60 |
|
|
|
32 |
|
36 |
|
|
60 |
|
||||||||
МPа |
|
|
|
|
|
|
|
|
|
|
|
|
|
|
|
|
|
|
|
|
|
|
|
||
|
|
|
|
|
|
|
|
|
|
|
|
|
|
|
|
|
|
|
|
|
|
|
|
||
Size of the |
|
№ 1 |
|
|
|
|
|
|
|
|
|
|
|
|
|
|
60 × 60 |
|
60 × 60 |
|
|||||
repairing |
|
|
|
|
|
|
300 × 300 |
|
300 × 300 |
|
|
|
|
|
|
|
|
|
|
|
|||||
|
|
|
|
|
|
|
|
|
|
|
|
|
|
|
|
|
|
||||||||
insertion, |
|
|
№ 2 |
|
|
|
|
|
|
|
|
300 × 300 |
|
300 × 300 |
|
||||||||||
|
|
|
|
|
|
|
|
|
|
|
|
|
|
|
|
|
|
||||||||
сm |
|
|
|
|
|
|
|
|
|
|
|
|
|
|
|
|
|
|
|
|
|
|
|
|
|
|
|
|
|
|
|
|
|
|
|
|
|
|
|
|
|
|
|
|
|
|
|
|
|
|
|
|
|
|
|
|
|
|
|
|
|
|
|
|
|
|
|
|
|
|
|
|
|
|
Таble 3 |
|
|
|
|
|
|
|
|
Results of the numerical experiments |
|
|
|
|
|
|
|
||||||||||||
|
|
|
|
|
|
|
|
|
|
|
|
|
|
|
|
|
|
|
|
|
|
||||
Variation |
Stress in the upper point of the repairing |
|
Stress at the boundary of the layers in the |
|
|||||||||||||||||||||
in the geometric sizes |
|
|
|||||||||||||||||||||||
of a defected area |
|
layer 1, МPа |
|
|
|
|
|
|
lower layer 2, МPа |
|
|
|
|||||||||||||
|
|
|
|
|
|
|
|
|
|
|
|
|
|
|
|
|
|
|
|
||||||
|
|
|
|
|
|
|
|
|
|
|
|
|
|
|
|
|
|
||||||||
|
|
|
a1 × b1, |
Results of the |
|
Results of the |
|
|
|
|
Results of the |
|
Results of the |
|
|
||||||||||
h1, сm |
|
|
calculation |
|
analytical |
|
∆, % |
|
calculation |
|
analytical |
|
∆, % |
|
|||||||||||
|
|
|
сm |
in the Lira |
|
|
|
in the Lira |
|
|
|
||||||||||||||
|
|
|
|
|
calculation |
|
|
|
|
|
calculation |
|
|
||||||||||||
|
|
|
|
|
|
software |
|
|
|
|
|
software |
|
|
|
||||||||||
|
|
|
|
|
|
|
|
|
|
|
|
|
|
|
|
|
|
|
|
|
|
||||
|
|
|
|
|
|
|
|
|
|
|
|
|
|
|
|
|
|
|
|
|
|||||
3.5 |
|
|
60 × 60 |
|
−2.03 |
|
−2.19 |
|
|
8 |
|
|
1.69 |
|
|
1.83 |
|
|
8 |
|
|||||
|
|
|
|
|
|
|
|
|
|
|
|
|
|
|
|
|
|
|
|
|
|
|
|
|
|
|
300 × 300 |
−1.95 |
|
−2.19 |
|
|
11 |
|
|
1.62 |
|
|
1.83 |
|
|
12 |
|
||||||||
|
|
|
|
|
|
|
|
|
|
|
|
||||||||||||||
|
|
|
|
|
|
|
|
|
|
|
|
|
|
|
|
|
|
|
|
|
|||||
7.0 |
|
|
60 × 60 |
|
−2.03 |
|
−2.01 |
|
|
1 |
|
|
1.69 |
|
|
1.08 |
|
|
36 |
|
|||||
|
|
|
|
|
|
|
|
|
|
|
|
|
|
|
|
|
|
|
|
|
|
|
|
|
|
|
300 × 300 |
−1.93 |
|
−2.01 |
|
|
4 |
|
|
1.61 |
|
|
1.08 |
|
|
33 |
|
||||||||
|
|
|
|
|
|
|
|
|
|
|
|
||||||||||||||
|
|
|
|
|
|
|
|
|
|
|
|
|
|
|
|
|
|
|
|
|
|
|
|
|
|
94
Issue № 4 (40), 2018 |
ISSN 2542-0526 |
A comparative analysis of the results of numerical and analytical calculations showed that the obtained values of the stress in the repairing material are in quite a good agreement with the difference of no more than 11 %.
Conclusions. The existing guidelines provide no methods for calculating rigid airfield surfacings reconstructed using local repairs. This is due to the complexity of the mathematical apparatus modeling the stress-strain of reconstructed plates and considering the geometric parameters of a repaired area, physical and mechanical characteristics of materials of a reconstructed structure and adhesive properties of a repairing material. In order to address this, theoretical foundations for calculating the stress-strain of a repaired rigid airfield surfacing under the impact of a static load of an aircraft were formulated analytically and by means of finite element method using a numerical modeling tool.
The above analytical calculation and numerical theoretical model can be employed for calculating repairing layers of rigid airfield surfacing using a specific condition in order to define the requirements for geometric parameters of a repaired area, physical and mechanical characteristics of a repairing material as well as to evaluate the durability of repaired areas.
The comparative analysis showed that an analytical method allows one to obtain only normal stress at the point of load application. Numerical modeling enables studies of deformations, normal, main and largest tangential stress at any point of a structure and isofields and their redistribution in a body of a structure.
Based on the results of the numerical experiments, it was found that changes in the stressstrain of a reconstructed structure are considerably impacted by the depth of a repaired area, adhesive properties of a repairing material and elasticity modulus of materials. Changes in the size of a repaired area in the plan have an insignificant impact on the stress-strain of a reconstructed structure. Thus for an approximate calculation it is acceptable to employ a calculation scheme of a locally repaired airfield plate as reconstructed along its entire area. In order to identify the optimal parameters of repairing materials, as part of studies of the stress-strain of a reconstructed airfield surfacing it is viable to use a numerical method.
References
1. Vasil'ev N. B., Boyko V. N., Usanov S. A. Sovremennykh podkhod k konstruirovaniyu aerodromnykh tsementobetonnykh pokrytiy [A modern approach to the construction of airfield concrete pavements]. Aeroporty. Progressivnye tekhnologii, 2007, no. 2, pp. 16—18.
95

Russian Journal of Building Construction and Architecture
2.Gorodetskiy A. S., Zavoritskiy V. I., Rasskazov A. O. Metod konechnykh elementov v proektirovanii transportnykh sooruzheniy [Finite element method in the design of transport facilities]. Moscow, Transport Publ., 1981. 143 p.
3.Grigolyuk E. I., Tolkachev V. M. Kontaktnye zadachi teorii plastin i obolochek [Contact problems of plate and shell theory]. Moscow, Mashinostroenie Publ., 1980. 416 p.
4.Grigolyuk E. I., Tolkachev V. M. Tsilindricheskiy izgib plastin zhestkimi shtampami Cylindrical bending of plates by rigid dies]. Moscow, Mashinostroenie Publ., 1976. 197 p.
5.Kogan B. I. Assimetrichnaya zadacha teorii uprugosti dlya mnogosloynogo poluprostranstva [Asymmetric problem of elasticity theory for multilayer half-space]. Izvestiya AN SSSR, 1958, vol. 6, pp. 57—61.
6.Kozlov G. N. Sukhie betonnye smesi «Emako» dlya remonta zhelezobetonnykh konstruktsiy transportnykh sooruzheniy [Dry concrete mixes "Emako" for repair of reinforced concrete structures of transport facilities]. Moscow, Informavtodor Publ., 2001, vol. 5, pp. 44—57.
7.Kul'chitskiy V. A., Vasil'ev N. B., Makagonov V. A. Aerodromnye pokrytiya. Sovremennyy vzglyad: fizikomatematicheskaya literatura [Airfield coverage. Modern view: physical and mathematical literature]. Moscow, Fizmatlit Publ., 2002. 528 p.
8.Kul'chitskiy V. A., Piskunov V. G., Prisyazhnyuk V. K., Vasl'ev N. B., Verizhenko V. E. Napryazhennodeformirovannoe sostoyanie sloistogo pokrytiya aerodromnoy odezhdy [Stress-strain state of layered coatings of the airfield clothing]. Izvestiya vuzov. Ser.: Stroitel'stvo i arkhitektura, 1984, no. 12, pp. 108—110.
9.Kul'chitskiy V. A., Sokolov A. N. Nekotorye resheniya zadachi ob izgibe trekhsloynoy plastiny na uprugom osnovanii [Some solutions to the problem of bending a three-layer plate on an elastic base]. Izvestiya vuzov. Ser.: Stroitel'stvo, 1994, no. 1, pp. 20—25.
10.Kul'chitskiy V. A., Chekov A. N., Muranova G. Yu. [Improvement of constructive solutions to the layers of the strengthening of airfield pavements]. Nekotorye nauchno-tekhnicheskie problemy voenno-stroitel'noy nauki: nauch.-tekhn. sb. k 50-letiyu institute [Some scientific and technical problems of military construction science]. Moscow, MADI Publ., 1996, pp. 696—707.
11.Korenev B. G., Chernigovskaya E. I. Raschet plit na uprugom osnovanii: posobie dlya proektirovshchikov
[Calculation of plates on an elastic base: a guide for designers]. Moscow, Gosstroyizdat Publ., 1962. 355 p.
12.Leshchitskaya T. P., Popov V. A. Sovremennye metody remonta aerodromnykh pokrytiy [Modern methods of airfield pavement repair]. Moscow, MADI (GTU) Publ., 1999. 131 p.
13.Mednikov I. A. [On the theory of bending of multilayer and reinforced road slabs]. Sb. nauch. tr. [Collection of proceedings]. Moscow, Soyuzdornii Publ., 1966, vol. 7, pp. 90—104.
14.Nikishin,V. S., Shapiro G. S. Prostranstvennye zadachi teorii uprugosti dlya mnogosloynykh sred [Spatial problems of elasticity theory for multilayer media]. Moscow, VTs AN SSSR Publ., 1970. 260 p.
15.Sinitsin A. P., Zhemochkin Yu. N. Prakticheskie metody rascheta fundamentnykh balok i plit na uprugom osnovanii bez gipotezy Vinklera [Practical methods of calculation of Foundation beams and plates on an elastic base without Winkler hypothesis]. Moscow, Gosstroyizdat Publ., 1962. 239 p.
16.Suladze M. D. Issledovanie deformatsiy zhestkikh pokrytiy dorog i aerodromov pri nalichii v ikh konstruktsii remontnykh vstavok [Study of deformations of hard surfaces of roads and airfields in the presence of repair inserts in their design]. Promyshlennoe i grazhdanskoe stroitel'stvo, 2015, no. 5, pp. 60—64.
96
Issue № 4 (40), 2018 |
ISSN 2542-0526 |
17.Tatarinov V. V. K voprosu rascheta zhestkikh pokrytiy aerodromov [To the question of calculation of hard surfaces of airfields]. Aeroporty. Progressivnye tekhnologii, 2012, no. 2 (55), pp. 24—27.
18.Artman D. H., Liebman J. S., Darter M. I. Optimization of lond-range major rehabilitation of airfield pavements. Transportation Research Record, 1983, no. 938, pp. 1—11.
19.Packard R. G. Design of Concrete Airport Pavement. PCA, 1973. 61p.
20.Shahin H. Y. Airfield pavement distress measurement and use in pavement management. Transport Res. Rec, 1982, no. 893, pp. 59—63.
21.Sudhakumar,J. Methods of repairing concrete structures. 26th Conference on our world in concrete and structures, 2001, pp. 63—71.
22.Tabatabaie A. M., Barenberg E.J. Structural analysis of concrete pavement systems. Мoscow, Transport Publ., 1980. 116 p.
23.Mahdy I. A., Domashevskaya E. P, Seredin P. V., Yatsenko O. B. Spectral features of Co-Ge-Te amorphous thin film. Optics and Laser Technology, 2010, vol. 43, iss. 1, pp. 20––24. doi: 10.1016/j.optlastec.2010.04.019.
97
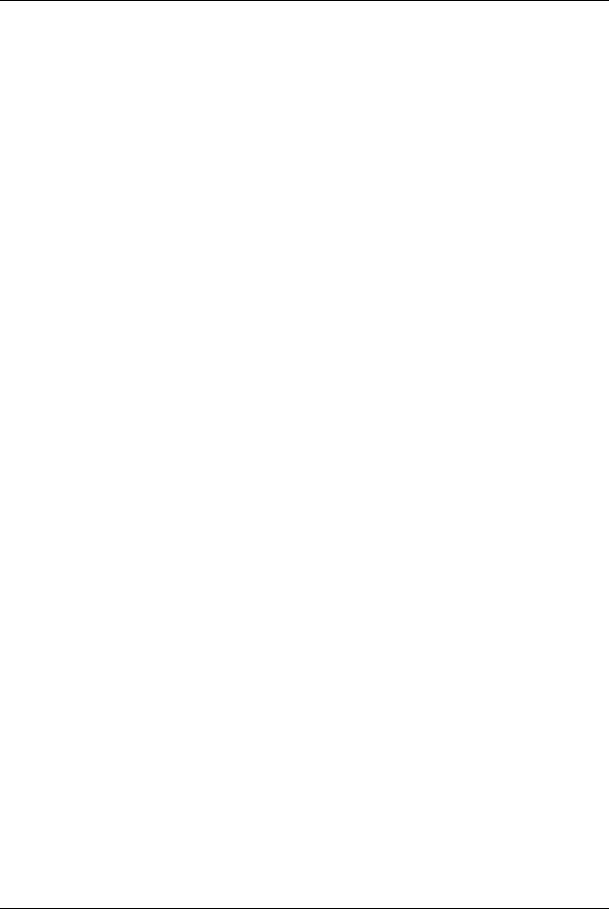
Russian Journal of Building Construction and Architecture
BUILDING MECHANICS
UDC 539.4 : 624.01
E. V. Poznyak1, S. A. Monin2
STATISTICAL MODELING OF A DYNAMIC RESPONSE
OF A STADIUM GRANDSTAND TO HUMAN LOAD
Moscow Power Engineering Institute National Research University
Russia, Moscow
1PhD in Engineering, Assoc. Prof. of the Dept. Of Robotics, Mechatronics, Dynamics and Machine Strength Named after V. V. Bolotin, tel.: +7-926-584-88-27, e-mail: PozniakYV@mpei.ru
2Master student of the Dept. Of Robotics, Mechatronics, Dynamics and Machine Strength Named after V. V. Bolotin, tel.: +7-929-627-24-52, e-mail: rfrnez@mail.ru
Statement of the problem. The aim of this paper is the estimation of the amplification factor of stadium grandstands in response to synchronous and non-synchronous activities of people. Human actions are modeled by concentrated half-sine force; nonsynchronous motions are caused by lag each person's actions and spatial distribution of people with different weights. The grandstand is modeled as a pivotally supported I-beam.
Results. Deflections of the model are determined by means of direct integration of dynamic equations. Estimating a random amplification factor and inconsistency factor are performed by means of the statistical modeling method. Resonant and non-resonant modes of the synchronous and nonsynchronous movements of people are considered.
Conclusions. The results showed that the values of the amplification factors obtained by using quasi-static approach by summing the response from each spectral component of the load, are higher than experimental values by 21—23 %, and nonsynchronous movements can significantly (up to 63 %) reduce the amplification factor.
Keywords: human-structure interaction, amplification factor, inconsistency factor, dynamic response, nonsynchronous motions.
Introduction. Consistent loads from human-structure interaction occur from synchronous movements of crowds of people that are common during sports and cultural events. Synchronous movements of spectators during a football match or a concert might cause perceivable vibrations,
© Poznyak E. V., Monin S. A., 2018
98

Issue № 4 (40), 2018 |
ISSN 2542-0526 |
disruption of operational criteria and sometimes local failures as well [5—7, 9—12, 14—21]. Stands made of suspending structures or consoles with a long are particularly sensitive to these loads with a long gibbet.
A load from spectators who are moving in synchrony is modeled with a sequence of semisinusoid impulses [13—21]:
|
|
|
t |
, |
0 t t |
|
, |
|
K |
p |
G sin |
|
p |
|
|||
F t |
|
|
|
|
|
(1) |
||
|
tp |
|
|
|
|
|||
|
|
|
|
|
tp t Tp , |
|
||
0, |
|
|
|
|
|
where G is a static load on stands from spectators (spectators’ weight); tp is time of contact; Tp is a period of impulses; Kp = π / (2α) is a shock factor where α = tp / Tp is a contact ratio; for pedestrian movement and low-rhythm aerobics α = 2/3, for rhythmic movements and high-rhythmic aerobics α = 1/2, for regular jumps α = 1/3, for high jumps α = 1/4 [17]. The frequency of impulses is fp = 1 / Tp; it was experimentally found that the frequency of impulses can range from 1 to 4 Hz [14—16, 18, 19].
The expansion of the function F (t) in a Fourier row [5] is known:
|
|
2 n |
|
2 n |
|
|
2 n |
|
||||||
F t G 1 an cos |
|
|
t bn sin |
|
|
t G 1 |
rn sin |
|
|
t n , (2) |
||||
T |
|
T |
|
|
|
|||||||||
|
n 1 |
p |
n 1 |
p |
|
|
n 1 |
T |
p |
|
|
|||
|
|
|
|
|
|
|
||||||||
|
|
|
|
|
|
|
|
|
|
|
|
where an, bn are Fourier coefficients, at 2nα = 1, an = 0, bn = π / 2, otherwise
an 0.5 |
cos 2n 1 1 |
|
cos 2n 1 1 |
, |
||||||||
|
|
|
|
|
|
|
|
|
|
|||
|
|
2n 1 |
|
|
2n 1 |
|
|
|||||
|
|
|
|
|
|
|
|
|
||||
bn 0.5 |
sin 2n 1 1 |
|
sin 2n 1 |
1 |
, |
|||||||
|
|
|
|
|
|
|
|
|||||
|
2n 1 |
|
|
2n 1 |
|
|||||||
|
|
|
|
|
|
|
|
|||||
rn |
|
an2 bn2 , |
n arctg an bn . |
|
Six or eight members of the row yield a good approximation of the function (1).
The ways of determining a dynamic reaction of a structure to consistent loads (1) were detailed in [5, 9] where two of them are described, i.e. quasistatic method in a deterministic and probabilistic context, and checked by means of direct integration of movement equations. Bear in mind that for the quasistatic method external impulse forces are applied to a structure as static loads, internal efforts do not depend on time, which makes construction calculations easier. Dynamic effects in the quasistatic method are accounted for using dynamism coefficients. At determined loading all the parameters of a calculation model and loading are con-
99

Russian Journal of Building Construction and Architecture
sidered strictly determined and dynamism coefficients are identified by means of the methods of the oscillation theory. In the probabilistic approach loading is seen as a random process and dynamism coefficients depend on spectral density of an input random process [1, 2].
The studies in [5, 9] revealed a typical feature of loading from consistent movement of spectators, i.e. extraordinarily high dynamism coefficients when a spectrum of own frequencies is in the range of possible frequencies of forced vibrations (1—4 Hz). It turned out that for high jumps a maximum dynamism coefficient is 14 at 5 % damping and 27 at 2.5 % damping. The authors of the study accounted for these dynamism coefficients with a narrow-band nature of loading when all the impulse energy is focused on certainly areas and spectral density of impact is a sequence of impulse functions at these frequencies; a detailed discussion and findings can be found in [9].
Note that high values of dynamism coefficients correspond to absolutely synchronous movements of people with the same phase, frequency, amplitude, which is obviously unlikely. It might be sensible to reduce dynamism coefficients considering inconsistency of people’s movements. In foreign methods it is suggested that the parameters of a dynamic reaction to a nonsynchronous coefficient which is 0.67 [13]. We were not able to find any references as to why an inconsistency coefficient is important in any available foreign scientific literature and regulations. It is not known how this coefficient was obtained and whether it was theoretical or experimental.
The problem might become more certain by introducing random parameters of a dynamic load such as amplitude, movement phase (it is pointed out how necessary it is to consider a random phase parameter in [12, 20]) as well as by taking into account uneven spatial distribution of impulses from each spectator on a model of a stand. Statistic modeling of a dynamic reaction will allow evaluation of coefficients of dynamism and inconsistency.
The objective of the paper is to obtain the justified coefficients of dynamism and inconsistency by means of a statistic modeling method considering a random nature of amplitude values and phase of a spatial vector on people’s consistent movements.
1. Coefficient of dynamism for a random impact. A way to determine a dynamic reaction for consistent movement of spectators is given in [5, 9]; in [3, 5] it can be found that a similar conclusion is made for formulas for a dynamism coefficient. When the problem started being addressed, it was assumed that an impact is simultaneous, synchronous and the forces have the same amplitudes, i.e. a one-dimensional impact was being dealt with. The solution of the movement equation in an established mode of forced vibrations was obtained when loading
100