
Инновационные процессы в исследовательской и образовательной деятел
..pdf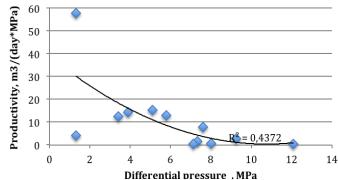
I.S. Poplygina
Perm National Research Polytechnic University
ENHANCING OIL RECOVERY OF PERM REGION OIL FIELDS
Regulatory issues in the development of oil fields with the use of methods of intensification of oil production and enhanced oil recovery. It is viewed The influence of power parameters of oil-containing reservoir on the effectiveness of the methods of stimulation of oil. Noted that, in order to enhance the effectiveness of the development to maintain reservoir pressure at the level of primary and operate wells down hole pressure not less than rational.
Key words: enhanced oil recovery, hydraulic fracturing, acidizing, bottomhole pressure, well yield.
At present, many of the oil deposits in the Perm region are in the stage of depletion. To boost oil production and develop residual stocks different methods of enhancing oil well production and increasing oil recovery are used.
Chemical methods are based on acidizing the bottom-hole using modified solutions of various composition. Physical methods comprise hydraulic fracturing, hydro sandblasting perforation, drilling additional wells and other. The hydrodynamic methods are based on cyclic water injection into the reservoir.
Wells with formation pressure above the saturation pressure are characterized by the highest well yield growth and the most durable effect of oil recovery enhancing measures [8]. Dynamics of the productivity rates of wells resulting from the geological and technical measures (GTM) depends on the bottom hole pressure (BHP) and the differential pressure on the seam (Fig. 1, 2) [4, 5, 7]. Initially the wells with low formation and bottomhole pressure have growth lower of productivity rates and well yield than wells with stratal pressure and bottom hole production pressure above the saturation pressure. With decreasing BHP and increasing productivity ratios on seam differential pressure wells after the intervention reduced [1, 2, 3, 6], production wells on the fluid will increase slightly.
Fig. 1. Effect of fracturing stimulation for differential pressure on well productivity
111

Fig. 2. Effect of acidizing for differential pressure on well productivity
The data available [3, 4] prove that the decrease in bottom-hole pressure to the value of gas saturation pressure leads to a 50…60 % decrease in well productivity factor; these data were obtained as a result of oil recovery enhancing measures at the Perm region. Then the effect, which degassing oil in place and changing the oil reservoir permeability due to its deformation had on the actual value of well productivity, was determined. Then the assessment was made to what extent degassing oil in place affect the actual value of well productivity. It was found that, when the bottom hole pressure is 0.3 of the saturation pressure, gas escaping from oil has the main impact on well productivity factor; these data were obtained on the production wells of Shershnevskoe oilfield.
The data considered in the paper allow to make the following conclusion. The reservoir energy is critical when it comes to applying different methods with the view to enhancing oil recovery. This is particularly true in case of oil formations characterized by high gas saturation of oil. It is essential to carry out Further in – depth research to determine the values of the rational bottom hole pressure and differential pressure for the oil fields in Perm region. Also, closer attention should given to the maintenance of formation pressure.
References
1.Поплыгин В.В., Головизина А.А. Прогнозирование отборов нефти при разработке нефтяных месторождений с учетом изменения продуктивности скважин // Нефть, газ и бизнес. – 2011. – № 8. – С. 24–26.
2.Поплыгин В.В., Галкин С.В., Давыдова И.С. К вопросу оптимизации систем разработки в условиях эксплуатационных объектов нефтяных месторождений предуральского краевого прогиба // Геология, геофизика и разработка нефтяных и газовых месторождений. – 2010. – № 12. – С. 54–58.
112
3.Поплыгин В.В. Прогнозирование продуктивности скважин и темпов нефтеизвлечения при высокой газонасыщенности пластовой нефти (на примере месторождений Верхнего Прикамья): автореф. дис. … канд. техн. наук. – СПб., 2011.
4.Мордвинов В.А., Поплыгин В.В., Ерофеев А.А. Влияние газа и деформаций коллектора на показатели работы скважин после гидроразрыва пласта // Нефтяное хозяйство. – 2012. – № 10. – С. 102–103.
5.Результаты обработок составом ДН-9010 призабойных зон пластов бш нефтяных месторождений района ВКМКС / В.В. Поплыгин, И.С. Давыдова, И.В. Кузнецов, С.В. Галкин // Вестник Пермского национального исследовательского политехнического университета. Геология. Нефтегазовое и горное дело. – 2010. – № 5. – С. 70–74.
6.Воеводкин В.Л., Галкин С.В., Поплыгин В.В. Прогнозирование дебитов нефти при технико-экономическом обосновании проектов освоения
ипоисков месторождений территории ВКМКС // Нефтепромысловое дело. – 2010. – № 7. – С. 45–48.
7.Мордвинов В.А., Поплыгин В.В., Сидоренко Д.Д.,
Шаймарданов А.Р. Продуктивность скважин после кислотных гидроразрывов пласта на Гагаринском и Озерном месторождениях // Нефтяное хозяйство. – 2013. – № 4. – С. 44–45.
113
L.A. Rybinskaya, V.I. Kychkin
Perm National Research Polytechnic University
MODELLING AND CALCULATION
OF THE LAYERED ROAD CONSTRUCTION UNDER
STRENGTH AND TEMPERATURE LOADS
Calculation of a road model is carried out by the finite element method in the software package. The results of the stress and displacement dependence, which occur in the upper layer of the road clothes, on the environment temperature are presented. Assessment criteria of the distorted layer state caused by temperature are determined.
Key words: stresses, displacements, finite element method, thermal load, distorted
layer.
The increase in endurance of road constructions that work in different climatic conditions and traffic loads has become a difficult-to-solve problem at present [1]. Its complexity is explained by the following:
–an automobile road construction is a complex system composed of materials which have a large variation of physical and mechanical properties;
–these properties depend substantially on time, operating conditions, fabrication methods and traffic load conditions.
It makes the assessment of endurance and construction durability more difficult. Thus, there is a necessity to create available means for an efficient analysis of the thermal and strength stress-strain state of non-rigid road clothes.
Automatic design software packages being road clothes calculationoriented, for example CREDO RADON, are known. However, these software packages do not solve joint thermo-elasticity problems on the basis of road constructions.
In the paper given the method of road construction state assessment under the complex influence of the traffic load and the temperature factor is considered in the following variations:
–the layer-by-layer temperature calculation at fixed thermo-physical parameters (thermal conductivity, thermal capacity, etc.);
–the displacement and stress calculation under the wheel load only;
–the temperature stress and displacement calculation with no account taken of the wheel load;
–the joint thermo-elasticity problem calculation by layers;
–the comparison of modelling results by stress and displacement parameters under the joint and total solution of a thermo-elastic road deformation problem.
114
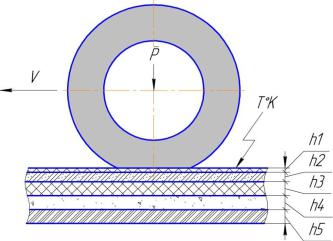
The third category road, that is a non-rigid road clothes construction composed of five layers was chosen for calculating. The layer thickness values were assumed to be fixed and equal to: h1 = 50 mm (upper layer), h2 = 70 mm, h3 = 200 mm, h4 = 300 mm, h5 was the basement soil thickness, according to [2]. The single vehicle wheel load, equal to 585 kPa (Fig. 1) was applied to the center of the picked space.
Fig. 1. The design diagram of the road clothes, load and temperature interaction
The calculation was carried out by using the SolidWorks Simulation software package. This software package includes the joint thermo-elastic problem statement and its solution. In spite of the fact that the software package is metal construction modelling and calculation-oriented, the experiment results showed that the road model could be designed by using the SolidWorks Simulation provided the material data base with the detailed property description for each material (such as, elastic module, Poisson's ratio, density, etc.) was applied to road bed layers.
The boundary conditions of road clothes [3], ambient temperature on the surface of the upper layer that contacts with the environment, as well as the natural convective heat exchange parameters were set in the temperature analysis. The convective heat exchange was set by the coefficient of air convective heat exchange (W/m² × K) and the mass ambient temperature (°C). Side borders were taken as non-heat-conducting.
The elastic module of the upper layer material was assumed to be variable depending on the soil temperature. The stress-strain state was investigated at different temperature conditions of the environment for winter and summer seasons from –30 °C to +50 °C, respectively.
115
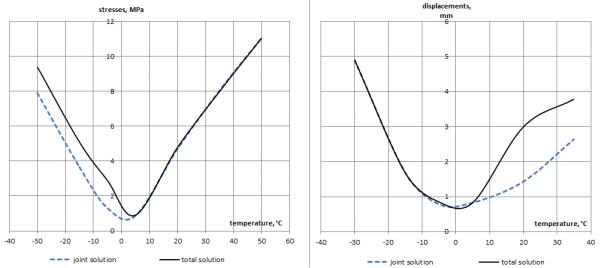
The finite element method was chosen as a calculating method. A high quality mesh with 56,311 tetrahedral elements and 80,994 nodes was built. The size of one element was equal to 111.104 mm. The level of zero temperature deformations was assumed to be about +5 °C.
The software package made it possible to determine the deflection cup parameters and calculate the corresponding stress-strain state factors of layer materials. The equivalent Mises stress was used as a criterion for strength assessment of the road layers.
|
|
1 |
|
|
|
von Mises |
|
|
x y |
2 |
y |
2 |
|
||||
|
|
|
|
|
|
|
|
|
|
2 |
2 |
2 |
0,5 |
Z |
2 |
Z x |
2 |
6 |
xy |
yZ |
xZ . |
|
|
|
|||||||
|
|
|
|
|
|
|
|
|
The calculated stress and displacement results are shown in Figure 2.
The similar calculating results for stresses are observed in the positive temperature area and those for displacement in the negative temperature area.
Fig. 2. Two variants of the equivalent Mises stress and displacements in the calculation point
The modeling results showed the significant dependence of displacements and stresses on the environment temperature at the assumed parameters of layer materials and thickness. It is clear from the analysis of the joint and total problem solution, that the calculated values for stresses in the negative temperature area can differ by 30 %, whereas the difference is insignificant in the positive temperature area. The displacement results practically coincide in the negative temperature area, but they differ by up to 48 % in the positive temperature area.
Therefore, the level of stresses is considered to be the criterion of the distorted layer condition assessment in the negative temperature area, whereas the level of displacements is the assessment criterion in the positive temperature area.
116
References
1.Апестин В.К. Что мешает приведению федеральных дорог в нормативное состояние? // Наука и техника в дорожной отрасли. – 2014. –
№2. – С. 25.
2.Торская Е.В., Лушников Н.А., Лушников П.А. Анализ напряженнодеформированного состояния многослойных дорожных одежд // Трение и износ. – 2008. – Т. 29, № 2. – С. 204–210.
3.Разработка и исследование энергосберегающих и подземных строительных конструкций и элементов / С.Р. Леви [и др.]. – Пермь: Издво Перм. гос. техн. ун-та, 2006. – 230 с.
117
A.V. Ryzhkov
Perm National Research Polytechnic University
COARSE-GRAINED MOLECULAR DYNAMICS MODEL
OF MICROFERROGEL SPECIMEN
Coarse-grained molecular dynamics method was applied for simulation of chain-like aggregates formation in small ferrogel objects, the so-called microferrogels. They are isolated polymer samples with embedded magnetic nanoparticles that can be used in biomedicine and diagnostics. Many-particle model was implemented in ESPResSo software. Structural changes in ferrogel during magnetization process were described by radial distribution function under variation of intensity of interparticle interactions.
Key words: microferrogel, magnetic nanoparticles, computer simulation, coarsegrained molecular dynamics, radial distribution function.
Magnetically sensitive composites based on soft polymer matrix and magnetoactive filler have broad prospects in actively growing spheres of nanomedicine and theranostics [1; 2; 3]. Interesting examples of such intelligent materials are the so-called microferrogels. They consist of submicron-sized polymer specimen as a carrier and magnetized nanosized particles as an active filler. This combination allows remote control and tuning of physical and mechanical properties of the sample (e.g. shape, density, stiffness, viscosity and elastic moduli) by applying external magnetic fields. So, it can be used for drug delivery, hyperthermia cancer treatment and many other innovative applications.
In spite of the fact that there is an extensive experimental experience in ferrogel study, it is still relevant to develop an adequate theoretical description of magneto-mechanical behavior for effective practical implementation of magnetoactive composites. In this sense computer simulation is an integral part of any comprehensive study. It is assumed that discrete numerical methods are able to describe and predict behavior of such complex systems as microferrogels through direct considering of interaction between elements. Current simulation was carried out using extension of molecular dynamics method, the so-called coarse-grained molecular dynamics. In a nutshell, an idea of molecular dynamics is the following: the system under consideration is represented as a set of large number of interacting particles via some interparticle potentials. Potentials are functions depending on interparticle distance and other parameters. They characterize the value of potential energy between the particles. Evolution of this set is calculated by integration of Newton's equations of motion until the stationary state is reached. To deal with microferrogels the coarse-grained modification of molecular dynamics (CGMD) is required. In CGMD some groups of particles (molecules or atoms) are treated as a single bead. It allows to
118
describe systems in different size scales. This approach has already been applied for simulation of magnetopolymer system response [4] and proved its power.
In our simulation we consider the 3D sample which is initially generated in the form of cubic lattice with 343 nodes (7×7×7). The nodes are filled with magnetic grains which are connected by polymer chains. Each magnetic particle has a dipolar moment and easy magnetization axis. They interact with each other due to dipolar-dipolar interaction which depends on distance and orientations of the moments. Chains consist of non-magnetic particles-"monomers" interacting via non-bonded repulsive hard-sphere potential and bonded potential. Bonded potential looks like non-linear elastic spring imitating chemical bonds between monomers. The entire composite "polymer chains + nanoparticles" system is placed into a constant temperature bath (Langevin thermostat model is used [5]). After thermalisation the external magnetic field switches on and induces transformation of internal structure and chain-formation. The simulation was carried out by open-source software ESPResSo [6]. It provides wide possibilities for extension via script writing for pre/postprocessing and source code modification for user-defined issues.
Calculation is interrupted when the stationary state is reached. The indicator of equilibration is approximately a constant value of mean-square displacement of magnetic beads relative to the initial state. Field-induced structures were analyzed by using radial distribution function (RDF), which describes how density varies as a function of distance from a reference particle. First of all we compared simulations without dipolar interaction and with it. It is clear that without attractive forces particles save their random character of arrangement after the process of thermal equilibration. On RDF plots we can see peaks in short distances, and it proves the presence of aggregates. Then we simulated another system with different strength of dipolar interaction (in fact different values of average magnetic moment of particles) and found how it influenced on RDF curve. With the increase of the dipolar forces aggregates manifest themselves in enhancement of peaks. Finally, in simulations at high temperatures chain-like structures were not observed visually and it also was confirmed by the shape of the RDF curve.
As a result in our model we tried to apply CGMD for simulation of structural changes in small ferrogels. It is a new and actively developing approach in the theory of smart materials. We also used RDF analysis that has similarities with experimental techniques to detect particle aggregates. Although it is important to emphasize that RDF is not an absolutely appropriate instrument for analyzing anisotropic structures, but for some typical configurations it is sufficiently valid. Subsequently we are introducing new methods of analysis.
119
References
1.Magnetic hydrogels and their potential biomedical applications / Y. Li, G. Huang, X. Zhang, B. Li, Y. Chen, T. Lu, T.J. Lu, F. Xu // Advanced Functional Materials. – 2013. – 23 (6). – P. 660–672.
2.Ma, X., Zhao, Y., Liang, X.-J. Theranostic nanoparticles engineered for clinic and pharmaceutics // Accounts of Chemical Research. – 2011. – 44 (10) – P. 1114–1122.
3.Thevenot, J., Oliveira, H., Sandre, O., Lecommandoux, S. Magnetic responsive polymer composite materials // Chem. Soc. Rev. – 2013. – 42. – P. 7099– 7116.
4.Weeber, R., Kantorovich, S., Holm, C. Deformation mechanisms in 2d magnetic gels studied by computer simulations // Soft Matter. – 2012. – 8. – P. 9923–9932.
5.Molecular dynamics with coupling to an external bath / H.J.C. Berendsen, J.P.M. Postma, W.F. van Gunsteren, A. DiNola, J.R. Haak // The Journal of Chemical Physics. – 1984. – 81 (8).
6.Espresso 3.1: Molecular dynamics software for coarse-grained models /
A. Arnold, O. Lenz, S. Kesselheim, R. Weeber, F. Fahrenberger, D. Roehm, P. Košovan, C. Holm // Griebel M., Schweitzer M.A. (Eds.). Mesh-free methods for partial differential Equations VI. – Vol. 89 of lecture notes in computational science and engineering. – Springer Berlin Heidelberg. – P. 1–23.
120