
- •Phototransduction
- •Sensitivity of photoreceptors
- •Photoreceptor mechanisms
- •Photoreceptor cells
- •Adaptation: calcium acts as a negative regulator
- •Photo-excitation of rhodopsin
- •Switching off the mechanism
- •Retinal, an inverse agonist?
- •Note on phototransduction in invertebrates
- •Olfaction
- •Olfactory receptor cells
- •Olfactory receptors
- •Transduction of olfactory signals
- •References

The Regulation of Visual Transduction and Olfaction
rearrangements in rhodopsin that generate activated rhodopsin (Rh*). This catalyses the exchange of GTP for GDP on transducin and provokes the dissociation of the complex, releasing the components t.GTP and-subunits. The -subunit then activates cGMP phosphodiesterase and cGMP is hydrolysed to 5 -GMP.
In the dark-adapted eye, cGMP is present in the cytosol of rod outer segments at a high concentration (40–80 M, about 300-fold higher than in the nerve cells of brain). There are effectively 10–20 molecules of cGMP for each molecule of rhodopsin. It binds directly to the cation channels to keep them in the open state. The sequence of events following illumination ensures enormous amplification of the signal such that a single photon, causing a single photoisomerization, can lead during the next second to the hydrolysis of more than 105 molecules of cGMP, leading to the closure of 500 cation channels, so blocking the influx of as many as 107 Na ions.
The cGMP-regulated channels are ligand-gated, but they differ from the ion channels operated by neurotransmitters in that the ligand is applied from the inside of the cell and it is normally in place in the unstimulated eye in the dark. Three molecules of cGMP are required to maintain the channel in its open state, with the result that the activation curve for channel closure is very steep indeed. The consequence of this is that once it is committed to opening or closing, as a result of a sufficient change in cGMP concentration, there is very little tendency for indeterminacy. Like an electrical switch, the channel
tends to be either open or shut. The 500 or so cation channels in a rod cell that close following the transduction of a single photon represent 3% of the total number that are open in the dark. The resultant hyperpolarization (membrane potential more negative) is 1 mV and lasts 1 second. This is sufficient to depress the rate of neurotransmitter release at the synapse which impinges on the nerve cells that transmit the onward signals.
Adaptation: calcium acts as a negative regulator
Given that the incidence of a singe photon can lead to the closure of 3% of the channels, it might seem that steady illumination of even modest
intensity would cause closure of the whole lot. Indeed, if the response of the system was directly proportional to the number of photoreceptor molecules activated, then full saturation would be achieved at very low light levels.
Yet, the human eye is capable of sensing small differences in the intensity of light against high background levels. Clearly, there must be an adaptive mechanism that reduces the amplification, so that there are always some open channels remaining even when the background is very bright. Overall
the eye is responsive over an intensity range of about 11 orders of magnitude,
167
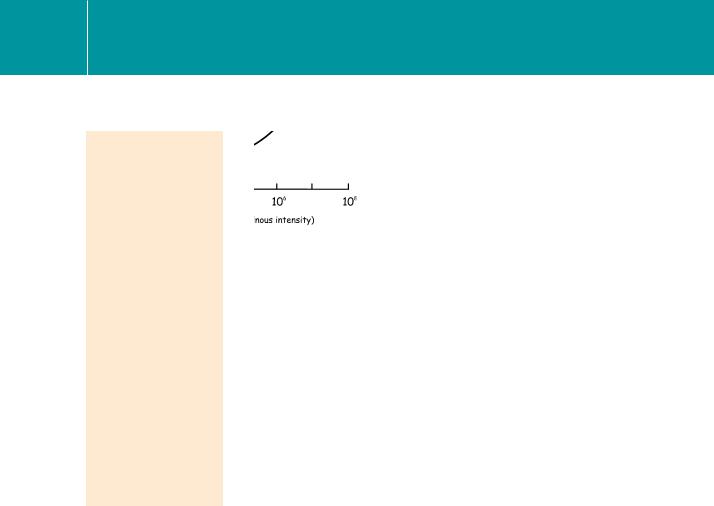
Signal Transduction
For example, this response allows us to perceive light–dark contrasts at levels
of illumination that range from that of a dark overcast night sky (~10 6 cd m 2) to the brilliance of sunlight reflected from snow fields (~107 cd m 2). The candela (cd) is an SI unit of measure of the intensity of visible light, called the luminous intensity. 1 cd is the luminous intensity, in
a given direction, of a source that emits
monochromatic radiation of frequency 540 1012 Hz (green light) and that has a radiant intensity in that direction of 1/683 W per steradian.
Fig 6.6 The shift in the flash response curve of mammalian cone photoreceptors as the background light level increases. Cone responses (instantaneous illumination plus background) measured with a bipolar electrode.
Modified from Valeton and Van Norren.13
a range that matches the extremes of the intensity of illumination at the Earth’s surface during the normal day–night cycle.12 Much of this range can be accounted for by the ability of individual photoreceptors to adapt by decreasing their sensitivity. Thus rod cells can adapt over 2 orders of magnitude and cones can adapt even more (over 7–9 orders; see Figure 6.6).
An important component of the adaptive mechanism by which the sensitivity is adjusted against ambient light levels is contributed by the cytosol concentration of free Ca2 . As the cation channels close in response to the hydrolysis of cGMP, the concentration of Ca2 declines from its dark level
of 300 nmol L 1 to 10 nmol L 1 under strong illumination. As always, the effects of Ca2 , whether stimulatory (e.g. causing the contraction of muscle) or inhibitory (as in the present case), are mediated through specific Ca2 - binding proteins. Here, the synthesis of cGMP by guanylyl cyclase is inversely and cooperatively regulated by Ca2 . This is mediated through its interaction with guanylyl cyclase activating protein (GCAP), a member of the EF-hand group of Ca2 -binding proteins (see page 233) permanently bound to its effector, guanylyl cyclase. Thus, in the dark, when the level of Ca2 in the cytosol is high, the rate of cGMP synthesis is low (Figure 6.7). This means that cGMP hydrolysed under low light conditions is not so readily replenished and the system is kept at its most sensitive. In strong light, the conversion of GTP to cGMP is accelerated due to the reduction in the concentration of free Ca2 . The free GCAP binds to the cyclase and stimulates the resynthesis of cGMP, which in turn opposes the closure of the membrane channels. The effect is to counteract the long-term effects of the light-activated hydrolysis of cGMP.
168

The Regulation of Visual Transduction and Olfaction
Fig 6.7 Photo-adaptation: a fall in intracellular Ca2 promotes cGMP synthesis, opposing the effect of cyclic GMP phosphodiesterase.
Calcium also acts as a negative signal at a number of other points in the chain of events. It controls the influx of cations and restricts the extent of membrane hyperpolarization. Although the reaction rate of the phosphodiesterase reaction appears to be unaffected, the concentration of Ca2 acts to regulate the lifetime of the activated enzyme. In the dark, through its interaction
with another EF-hand Ca2 -binding protein, recoverin (see page 233), Ca2 prolongs the signal through inhibition of rhodopsin kinase. The decline
in cytosol Ca2 under intense illumination is permissive of rhodopsin phosphorylation and this necessarily curtails the lifetime of the activated phosphodiesterase (see Figure 6.11). Other sites of action of Ca2 may include transducin (low Ca2 might accelerate the GTPase reaction and so curtail the activation signal at this level) and the cGMP-regulated ion channels. As cytosol free Ca2 declines during illumination, the affinity of the channels for cGMP increases. This must oppose the effects of the fall in the concentration of cGMP, favouring the open state.
To summarize, these highly integrated forms of automatic gain control arise in response to the generation of two signals as the membrane cation channels close in response to light. One of these is purely electrical and is conveyed
as a pulse of hyperpolarization to the synaptic body, depressing the rate of release of the transmitter, glutamate. The other signal is chemical and results from the reduction in the concentration of cytosol Ca2 . In essence,
the light signal to these cells acts as a negative stimulus. In the dark, they are partially depolarized and the rate of transmitter release at the synaptic body is maximal. In the light, they become hyperpolarized and the rate of transmitter release is depressed.
169
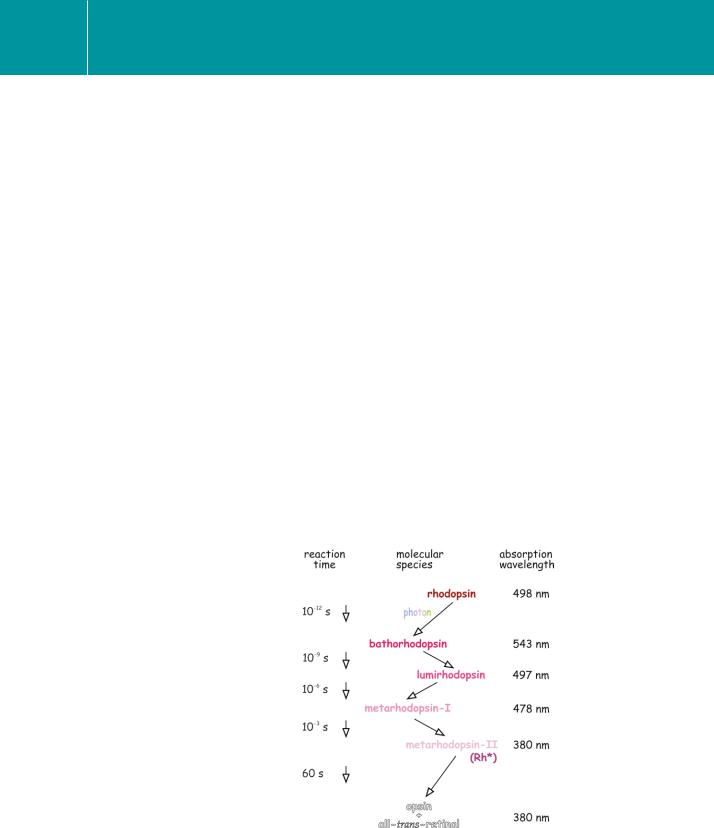
Signal Transduction
In the long term, as in the diurnal cycle, it appears that adaptation to intense illumination is also mediated by a redistribution of transducin between the outer and the inner segments of the retinal rod cells. As much as 90% of
the transducin present in the outer segments in dark-adapted conditions can translocate into other compartments of the cell on a time scale of tens of minutes.14 This is accompanied by a corresponding 10-fold reduction in amplification of the signal, allowing the eye to maintain its sensitivity to changes in light against the brighter daytime background.
Photo-excitation of rhodopsin
The initial light-induced isomerization of 11-cis-retinal to all-trans-retinal, which occurs within picoseconds, does not immediately trigger rhodopsin to catalyse the exchange of guanine nucleotides on transducin. The first rapid event is followed by a series of dark reactions. These have been characterized spectrally as the stepwise shift of the peak wavelength of light absorption of the photoreceptor protein to shorter wavelengths as the ligand and the opsin form a series of intermediates (Figure 6.8).
The early forms – bathorhodospin, lumirhodopsin, and metarhodopsin-I
– are unstable. It is metarhodopsin-II, also called photo-excited rhodopsin (Rh*), formed within milliseconds of light absorption, which initiates
the transduction cascade by inducing guanine nucleotide exchange on transducin.15–17 However, in contrast with ‘conventional’ G-protein
Fig 6.8 Steps following isomerization of retinal.
170