
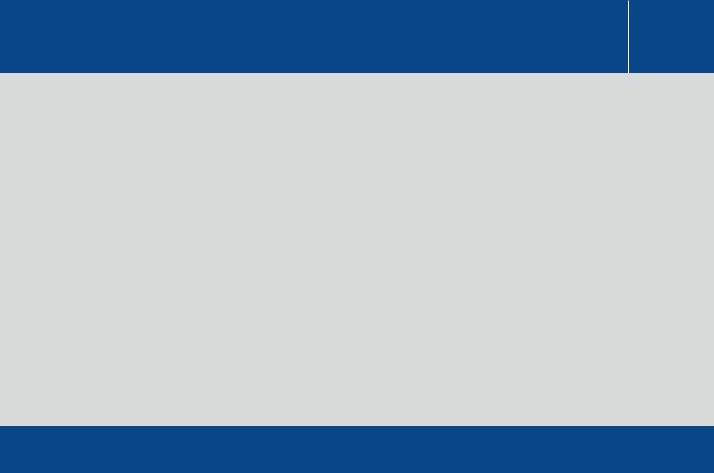
Chapter 7
Intracellular calcium
Calcium is an abundant element. It constitutes 3% of the Earth’s crust and it is prominent throughout the biosphere. It is present in fresh and sea
water at levels that range from micromolar to millimolar. Ca2 ions and Ca2 - binding proteins are essential for many biochemical processes in prokaryotic and eukaryotic cells. In this chapter and in Chapter 8, we shall see that Ca2 has special roles in the signalling mechanisms that regulate the activities of eukaryotic cells and that this depends upon the sensitivity of some proteins to the concentration of free Ca2 in their surroundings.
A new second messenger is discovered
A number of the more enduring truths of science have been discovered when a failure of vigilance is coupled with uncommon perspicacity. Sydney Ringer’s discovery of a requirement for calcium in a biological process must surely be placed in this category.1,2
Henry Dale’s account:
Ringer was a physician to University College Hospital, and, in such time as he could spare from his practice, one of the pioneers of pharmacological
185

Signal Transduction
research in this country. In his early experiments he had found that a solution containing only pure sodium chloride, common salt, in the proportion in which it is present in the serum of frog’s blood would keep the beat of the heart in action for only a short time, after which it weakened and soon stopped. And then suddenly the picture changed: apparently the same pure salt solution would now maintain the heart in vigorous activity for many hours. Ringer was puzzled, and thought for a time that the difference must be due to a change in the season of the year – until
he discovered what had really happened. Being busy with other duties, he had trusted the preparation of the solutions to his laboratory boy, one Fielder; and as Fielder himself, who I knew as an ageing man, explained to me, he didn’t see the point of spending all that time distilling water for Dr
Ringer, who wouldn’t notice any difference if the salt solution was made up with water straight out of the tap. But, as we have seen, Ringer did notice the difference; and when he discovered what had happened he did not merely become angry and insist on having distilled water for his saline solution, he took full advantage of the opportunity which accident had thus offered him and soon discovered that water from the tap, supplied then to North London by the New River Water Company, contained just the right small proportion of calcium ions to make a physiologically balanced solution with his pure sodium chloride . . .’
Hints of a wider role for calcium soon followed with Locke’s demonstration3 that removal of calcium could block the transmission of impulses at the neuromuscular junction in a frog sartorius muscle preparation. The realization that this requirement for calcium is due to its role in controlling the secretion of a chemical messenger (neurotransmitter) had to wait nearly 50 years.4,5
The direct introduction of Ca2 ions into muscle fibres to cause contraction was first reported by Kamada and Kinoshita in 1943.6 At this time Japan and the USA were at war, and as a result of the breakdown of communication, the credit for this finding has generally been ascribed to the Americans Heilbrunn and Wiercincki who reported their results 4 years later.7 Other cations such as Na , K , and Mg2 were found to be without effect. It had been Heilbrunn’s contention, at that time at least, that the effect of calcium was on the ‘general colloid properties of the protoplasm’ and that the effects of ions on isolated proteins would lack biological relevance.8 However,
Otto Loewi (see Chapter 1) who attended their presentation at the New York Academy of Sciences was heard to growl ‘Kalzium ist alles’ (calcium is
everything). So it remained, until the biochemical understanding of signalling mechanisms became more developed, taking in cyclic AMP (Chapter 5), GTPases (Chapter 4), tyrosine kinases (Chapter 11), PI 3-kinases (Chapter 13), and much more. Even with his foresight, however, had Loewi lived to the end of the century, he would surely have been astounded by the prominence of calcium in contemporary biology.
186
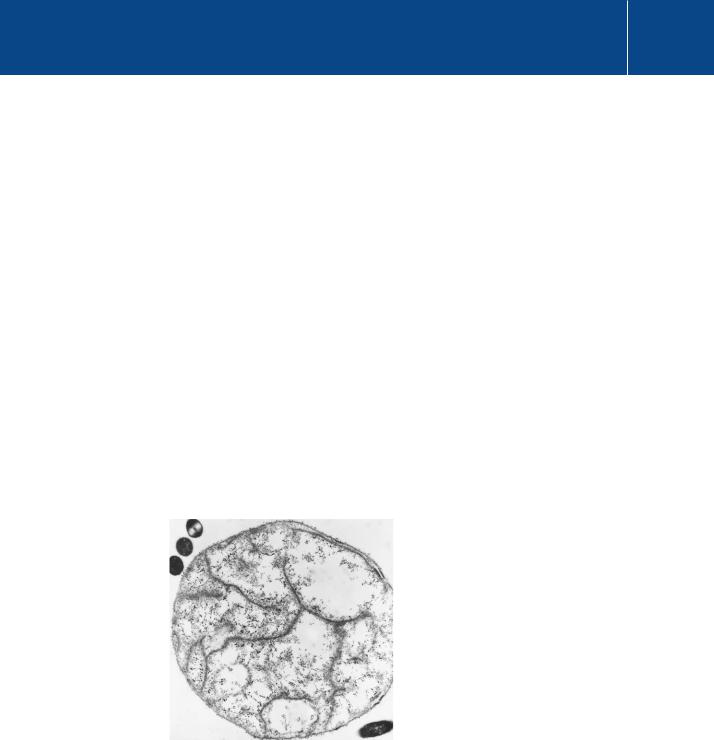
Intracellular calcium
In previous chapters we have seen how the idea of second messengers followed from the work of Sutherland and Rall,9 showing that the generation of cAMP represents the essential link between membrane events and the metabolic process in the signalling of glycogenolysis. Reflecting this history, cAMP was said to be the first second messenger and Ca2 the second. In fact, elevation of cytosol Ca2 is more ubiquitous than elevation of cAMP and it regulates a very diverse range of activities, including secretion, muscle contraction, fertilization, gene transcription, and cell proliferation.
Calcium and evolution
The evolution of the multicompartment structure of eukaryotic cells from their prokaryotic forerunners is thought to have occurred in stages, involving successive invaginations and internalizations of the cell membrane. Some modern bacteria provide evidence for this (Figure 7.1). The internalization
of elements of the plasma membrane had the effect of segregating specific functions on to specialized intracellular structures, such as the nuclear envelope, the smooth and rough endoplasmic reticulum, the Golgi membranes, lysosomes, etc. A probable early outcome of this process was the formation of a reticular compartment responsible for protein synthesis
– a ‘protoendoplasmic’ reticulum. The concentration of free Ca2 within prokaryotic cells is kept very low by powerful ion translocation mechanisms that expel Ca2 ions. The early endoplasmic reticulum (and other organelles)
Fig 7.1 Thiovulum, a sulfur-oxidizing bacterium possessing endoplasmic membrane structures.
This exceptionally large bacterium (diameter 6 m) possesses an intracellular membrane system, possibly including a rudimentary rough endoplasmic reticulum. The black dots inside the cell are probably ribosomes. The dark blobs at the top left are other bacteria.
By courtesy of Tom Fenchel, Marine Biological Laboratory Helsingør, Denmark.
187
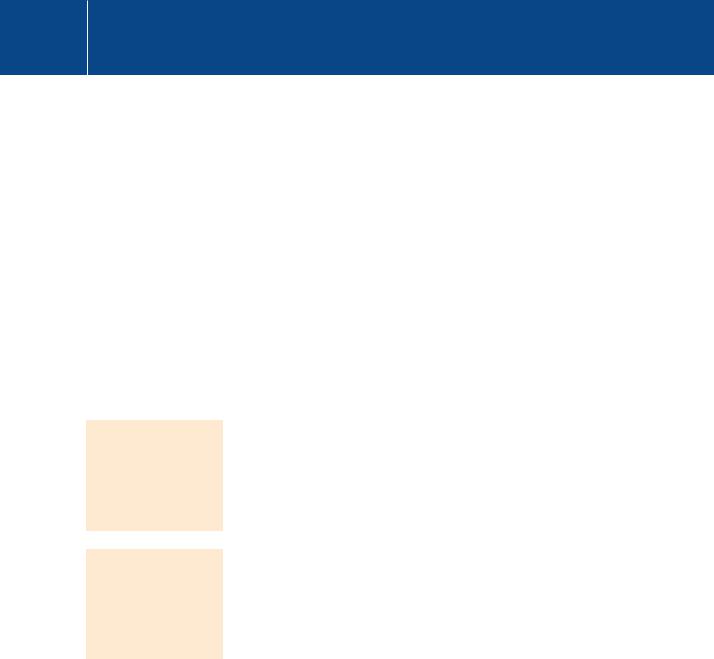
Signal Transduction
The term ligand was first coined by chemists to describe an electrondonating group that forms coordination complexes with metal ions.
The stability constant
K is the reciprocal of the dissociation constant KD, which represents the concentration of free cation M at which the ligand is half saturated.
inherited these mechanisms allowing it to draw the ion into its lumen from the cytosol, to provide an intracellular Ca2 store.
The formation of specialized membranes and organelles imposed a need for eukaryotic cells to elaborate the means of communication between their various internal compartments and the external world. Furthermore, the later evolution of complex metazoan organisms having differentiated cells with individual specialized functions necessitated increasingly complex signalling systems. These would enable the coupling of extracellular signals to intracellular signalling pathways and thence to specific downstream effectors. Calcium is well suited for the role of an intracellular messenger, for two main reasons. One is that its immediate proximity provides the opportunity for it to enter the cytosol directly, through membrane channels, either from the extracellular environment or from the internal reticular compartment; the other is provided by calcium’s distinctive coordination chemistry.
Distinguishing Ca2 and Mg2
While nature seems to have taken advantage of the presence of Ca2 , its suitability for signalling depends upon its ability to form stable complexes with particular biological molecules. The propensity of metal ions such as Ca2 and Mg2 to form coordination complexes with anionic or polar ligands can be described by equilibria of the form
M L K ML
in which ML represents the complex of the metal ion M with the ligand L. The stability (equilibrium) constant K is a measure of the stability of the complex. All ions in aqueous solution are surrounded by a hydration shell, so in order for coordination to occur, water molecules must be displaced from both the metal ion and the ligand. The factors that determine the stability therefore include not just the electrostatic attraction between cation and ligand, but also attractive and repulsive interactions between adjacent coordinating ligands. Importantly, water is itself a ligand that stabilizes the ionized state. Not all of the water molecules present in the hydration shell need necessarily be removed to form a complex. The stability of the complex will therefore depend upon the energy of association of M and L, set against the energies of association of M and L separately with water.
Ca2 is remarkably different from Mg2 in these respects.10 This is principally because Ca2 is larger and has less difficulty accommodating ligand atoms around its surface. The number of atoms that bind to it in a complex (the
188
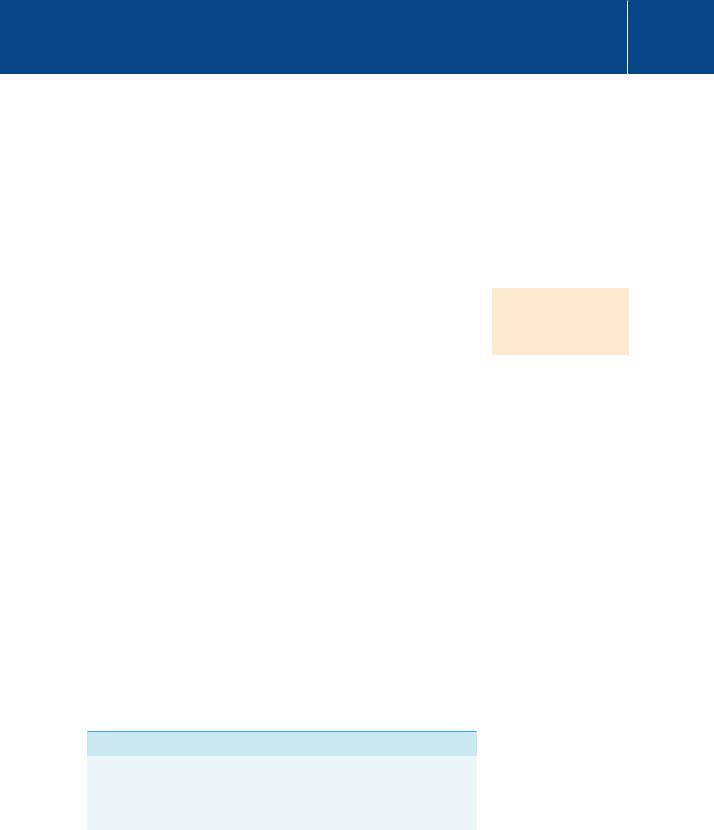
Intracellular calcium
coordination number) is variable, in the range 6–12, and the geometry of the arrangement around the coordination sphere is rather flexible. Conversely, Mg2 forms complexes in which the ligand atoms are nearly always arranged in an octahedral formation (a coordination number of 6). For small anions that can be accommodated in such a structure, the strength of association with Mg2 is greater than with Ca2 . For bulky and irregularly shaped anions, for which the octahedral requirement cannot be satisfied, the preference is reversed.
Chelating agents are synthetic compounds that consist of an array or cage of several ligand atoms. Such multidentate (‘many-toothed’) assemblies, particularly those that form an irregular structure, can be very selective
for Ca2 over Mg2 , because of the ability of Ca2 to tolerate an irregular geometry. One of the most specific Ca2 chelators is EGTA (Figure 7.2). This molecule possesses four carboxyl groups and two ether oxygens and exhibits a 105-fold selectivity for Ca2 over Mg2 . (Note that Ca2 overwhelmingly prefers oxygen atom donors.) EGTA and similar compounds are used in
the laboratory as Ca2 buffers to control the concentration of free Ca2 in experiments.
Free, bound, and trapped Ca2
In the biological world, Ca2 may be considered to exist in three main forms: free, bound, and trapped. In vertebrates, calcified tissues such as bones and teeth account for the major proportion of body calcium. Within these tissues calcium is trapped in mineral form (with phosphate) as hydroxyapatite.
Apart from its structural function, bone provides a reservoir of slowly exchangeable calcium that can be mobilized, when needed, to maintain a steady extracellular concentration (Table 7.1). The total amount of calcium in extracellular fluid, in comparison with the mineralized tissues, is very small and of this, only about half can be regarded as free. The remainder is bound mostly to proteins in the extracellular milieu. Within cells, the concentration of free Ca2 inside the cytosolic compartment is still lower, by about four orders of magnitude. A considerable proportion of intracellular calcium is also bound
Fig 7.2 EGTA (ethylene-bis(2- aminoethylether)-N,N,N9,N9- tetraacetic acid).
The word chelate is derived from the Greek chela, meaning the claw of a crab or lobster.
Table 7.1 Approximate levels of free Ca2 and Mg2
|
[Ca2 ] |
|
[Mg2 ] |
Plasma, extracellular fluid |
1–2 mM |
pCa 3 |
1 mM |
|
|
|
|
Intracellular cytosolic (resting cells) |
50–100 nM |
pCa 7 |
0.5–1 mM |
|
|
|
|
Intracellular lumenal (ER) |
30–300 M |
pCa 5–4 |
|
|
|
|
|
189

Signal Transduction
Electrochemical potential gradient: the combined effect of the concentration and voltage gradients.
to proteins and these exhibit a wide range of affinities. More importantly, many are able to bind Ca2 ions in the presence of a huge excess of Mg2 . This is because the arrangement of the amino acid side chains that bear the coordinating atoms tends to create sites of irregular geometry. There is also structural flexibility. This has important consequences for the ‘on–off’ kinetics of binding.
In the extracellular environment, free Ca2 and Mg2 are both present at millimolar concentrations (Table 7.1). Low affinity Ca2 -binding proteins provide a short-term local buffer, while in vertebrates at least, long-term (homeostatic) control of free extracellular Ca2 is maintained by a hormonal mechanism
that utilizes the mineralized tissue reservoirs. Failure to maintain a stable level of extracellular Ca2 can have serious consequences. Excess circulating Ca2 (hypercalcaemia) reduces neuromuscular transmission and causes myocardial dysfunction and lethargy. Hypocalcaemia affects the excitability of membranes and if left untreated leads to tetany, seizures, and death.
Cytosol Ca2 is kept low
Ca2 -binding proteins within prokaryotic and eukaryotic cells fulfil a wide range of functions (see Chapter 8). Some, such as the membrane pumps and ion exchanger, are employed to keep cytosol [Ca2 ] low. Others act as Ca2 buffers. As already mentioned, a low Ca2 level in the cytosol of eukaryotic cells is maintained by ATP-dependent transporters in the plasma membrane that eject Ca2 from the cell, while others transport the ion into the lumen of the endoplasmic reticulum (ER) or in muscle cells the sarcoplasmic reticulum (a specialized type of ER). The pumps are transmembrane proteins that move Ca2 ions across membranes against their electrochemical potential gradients. They are called Ca2 -ATPases and they provide primary active transport. Those in the membrane of the ER and SR are called sarcoplasmic/ endoplasmic reticulum Ca2 ATPases (SERCAs). Extrusion of Ca2 from the cytosol by plasma membrane pumps is supported by secondary active transport through Na Ca2 exchangers: transmembrane proteins that use the inward Na -electrochemical gradient to transport Ca2 ions out of the cell.
Changes in cytosol Ca2 concentration are also resisted by resident, highaffinity Ca2 -binding proteins, some of which may be confined to particular locations within the cytosolic compartment. All of them may act as buffers that tend to restrict local changes of Ca2 , although their capacity is not high and the speed at which they take effect will depend on the binding kinetics. Within the ER, low-affinity Ca2 -binding proteins (KD 2 mmol L 1) such
as calreticulin, can retain up to 20 mol of Ca2 per mol of protein, greatly increasing the capacity of this intracellular store. Estimates of ER [Ca2 ] vary between 100 mol L 1 and 800 mol L 1.
190