
Кочубей СПЕКТРОСКОПИЯ РАССЕИВАЮЩИХ СРЕД
.pdf2 , |
(214) |
где - случайное число в интервале между 0 и 1. В случае изотропного рассеяния угол определяется как:
|
|
cos 2 1. |
|
|
|
(215) |
|||
Для случая анизотропного рассеяния, при использовании фазовой функции |
|||||||||
Хеньи-Гринштейна, угол может быть определен как: |
|
|
|
||||||
1 |
|
2 |
|
1 g |
2 |
|
2 |
|
|
|
|
|
|
||||||
cos |
|
1 g |
|
|
|
|
|
|
|
2g |
|
|
|
|
|||||
|
|
|
1 g 2g |
|
(216) |
||||
|
|
|
|
|
|
|
|
. |
Когда направление движения и длина пробега фотона известны, определяется, остается ли фотон в результате данного перемещения в том же слое или попадает в другой. Если фотон остается в том же слое, то он перемещается в рассчитанную точку. Если фотон попадает в другой слой, то по формулам Френеля разыгрывается событие, состоящее в отражении фотона. Вероятность того, что фотон отразится от границы раздела двух сред, определяется коэффициентом отражения Френеля. Число , случайным образом распределенное между 0 и 1, определяет, отразится фотон от границы раздела или пересечет ее. Если R i , то фотон отразится от границы раздела, в
противном случае фотон пересечет ее. Если фотон пересекает границу, то по формуле Снеллиуса вычисляется его новое направление, а оставшаяся часть длины пробега l изменяется: l' l *ni /ni 1 . Здесь l' - новое значение оставшейся части длины свободного пробега фотона, при перемещении его из слоя i в слой i+1. Когда фотон пересекает границу раздела двух сред с различными показателями преломления, то ему приписывается новый вес, равный W 1 R i
. В случае отражения фотона, ему приписывается вес, равный W R i . После каждого шага перемещения, за исключением случая, когда фотон выходит за границы среды, часть веса фотона, равная 1 a W , записывается в ячейку массива Qij, определяющего распределение поглощенной в среде энергии. Здесь W - текущий вес фотона, а a – альбедо единичного акта рассеяния. Подобная техника моделирования подразумевает, что под каждым фотоном надо понимать пакет фотонов, распространяющийся в среде. При этом размер пакета определяется весовым коэффициентом, в начальный момент времени равным W0. Значения индексов (i, j) вычисляются по текущим координатам фотона. Затем генерируются новые значения угла рассеяния, и длины свободного пробега фотона, и процедура повторяется. Если вес фотона становится меньше некоторого изначально заданного значения, то разыгрывается процедура называемая "русской рулеткой". В случае, если на очередном шаге моделирования фотон попадает за пределы исследуемой среды, то часть его веса, пропорциональная коэффициенту пропускания границы, записывается в ячейку массива QTj (пропускание) или QRj (отражение). Значение индекса i определяется текущими
79
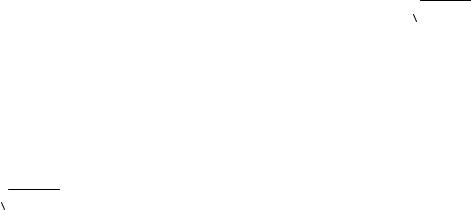
координатами фотона, а именно значением x2 y2 . После выполнения описанной процедуры для достаточно большого числа фотонов Nphs вычисляются функции Грина отклика среды:
Gij |
Qij |
/ NphsVij aijW0 , |
(217) |
Gli |
Qli |
/ NphsSiW0 , |
(218) |
где Vij - объем ячейки |
(i,j), aij - коэффициент поглощения слоя, |
которому |
принадлежит ячейка (i, j), Si - площадь кольца, соответствующая расстоянию
x2 y2 .
И, наконец, по заданному профилю падающего пучка, с помощью формул (208) и (209), вычисляется распределение освещенности внутри среды, а также распределения прошедшего и отраженного излучения. Суммируя элементы массивов QTj (пропускание) или QRj (отражение), и выполняя нормировку полученных величин на число фотонов, используемых для моделирования, мы получаем значения коэффициентов диффузного отражения и полного пропускания.
Применение метода Монте-Карло базируется на использовании макроскопических оптических свойств среды, которые предполагаются однородными в пределах небольших объемов образца. Известные алгоритмы позволяют учесть прохождение света через сложные образцы, состоящие, например, из нескольких слоев с различными оптическими свойствами, при этом надо учитывать также конечный размер падающего пучка, отражение света от границ раздела слоев. При высокой точности и универсальности главным недостатком метода Монте-Карло является большие затраты машинного времени. Хотя развитие аппаратных и программных средств вычислительной техники уменьшает роль фактора времени, необходимо создание эффективных сравнительно простых и надежных алгоритмов метода Монте-Карло. Например, метод конденсированного Монте-Карло позволяет получить решение для любого значения альбедо(Λ=μs/μt) на основе моделирования для одного конкретного значения альбедо, что существенно ускоряет расчеты.
80
ЛИТЕРАТУРА
1.Richards–Kortum R., Sevick–Muraca E. Quantitative optical spectroscopy for diagnostics. // Annu. Rev. Phys. Chem, 1996. – Vol.47. – P.555-606.
2.Optical Tomography, Photon Migration and Model Media: Theory, Human Studies, and Instrumentation. // Ed. by B.Chance, R.Alfano. – Proc.: SPIE 2389, 1995.
3.Schuitmaker J.J., Baas P., van Leengoed H.L., van der Meulen F.W., Star W.M., van Zandwijk N. Photodynamic therapy: a promising new modality for the treatment of cancer. // J. Photochem. Photobiol. B, 1996. – Vol.34. – P.3-12.
4.Laser–induced Interstitial Thermotherapy. // Ed. by Muller G., Roggan A. – SPIE Press, PM25, 1995
5.Тучин В.В. Лазеры и волоконная оптика в биомедицинских исследованиях. – Саратов: Изд-во Сарат. ун-та, 1998.
6.Das B.B., Liu F., Alfano R.R. Time–resolved fluoresence and photon migration studies in biomedical and random media. // Rep.Prog.Phys., 1997. – Vol.60. – P.227-292.
7.Bolin F.P., Preuss L.E., Taylor R.C., Ference J. Refractive index of some mammalian tissues using a fiber optic cladding method. // Appl.Opt, 1989. - Vol.28. – P.2297-2305
8.Flock S.T., Wilson B.C., Patterson M.S. Total attenuation coefficient and scattering phase function of tissues and phantom materials at 633 nm. // Med.Phys., 1987. - Vol.14. – P.835-841.
9.Jacques S.L., Alter C.A., Prahl S.A. Angular dependance of HeNe laser light scattering by human dermis. // Lasers in Life Sciences, 1987. – Vol.1. – P.309-
333.
10.Marchesini R., Bertoni A., Andreola S., Melloni E., Sihirollo A.E. Extinction and absorption coefficients and scattering phase functions of human tissues in vitro. // Appl.Opt., 1989. – Vol.28. – P.2318-2324.
11.Peters V.G., Wyman D.R., Patterson M.S., Frank G.L. Optical properties of normal and deseased human tissues in the visible and near–infrared. // Phys.Med.Biol., 1990. – Vol.35. – P.1317-1334.
12.Van der Zee P., Essenpreis M., Delpy D.T. Optical properties of brain tissue. // Proc. SPIE, 1993. – Vol.1888. – P.454-465.
13.Staveren H.J., Moas J.M., van Marle J., Prahl S.A., van Gemert J.C. Light scattering in Intralipid–10% in the wavelength range of 400 –1100 nm. // Appl.Opt., 1991. – Vol.30. – P.4507-4514.
14.Taddeucci A., Martelli F., Barilli M., Ferrari M., Zaccanti G. Optical properties of brain tissue. // J.Biomed. Opt., 1996. – Vol.1. – P.117-123.
81
15.Zdrojkowski R.J., Longini R.L. Optical transmission through whole blood illuminated with high collimated light. // J.Opt.Soc.Am., 1969. – Vol.59(8). – P.898-903.
16.Fecht I., Johnson M. Non–contact, scattering–independent water absorption measurement using a falling stream and integrating sphere. // Meas.Sci.Technol., 1999. – Vol.10(7). – P.612-618.
17.Milburn D.I., Hollands K.G.T., Kehl O. On measurement techniques for the spectral absorptance of glazing materials in the solar range. // Solar Energy, 1998. – Vol.62(3). –163-168.
18.Zhang Z., Modest M.F. Temperature–dependent absorptances of ceramics for Nd:YAG and CO2 laser processing applications. // J.Heat.Transfer, 1998, – Vol.120(2). – P.322-327.
19.Nostell P., Roos A., Ronnow D. Single–beam integrating sphere spectrophotometer for reflectance and transmittance measurements versus angle of incidence in the solar wavelength range on diffuse and specular samples. // Review of Scientific Instruments, 1999. – Vol.70(5). – P.2481-2494.
20.Ulbricht R. Die Bestimmung der mittleren räumlichen Lichtintensität durch nur eine Messung. // Electrotech.Z., 1900. – Vol.21. – P.595-597.
21.Taylor A.H. The measurement of diffuse reflection factors and a new absolute reflectometer. // J.Opt.Soc.Am., 1920. – Vol.4. – P.9-23.
22.Jacquez J.A., Kuppenheim H.F. Theory of the integrating sphere. // J.Opt.Soc.Am., 1955. – Vol.45. – P.460-470.
23.Clare J.F. Comparison of four analytic methods for the calculation of irradiance in integrating spheres, J.O.S.A. (A). // Optics,Image,Science, Vision, 1998. – Vol.15 (12). – P.3086-3096.
24.Pickering J.W., Moes C.J.M., Sterenborg H.J.C.M, Prahl S.A., van Gemert M.J.C. Two integrating spheres with an intervening scattering sample. // J.Opt.Soc.Am., 1992. – Vol.A 9. – P.621-631.
25.Pickering J.W., Prahl S.A., van Wieringen N., Beek J.F., Sterenborg H.J.C.M., van Gemert M.J.C. Double–integrating–sphere system for measuring the optical properties of tissue. // Appl.Opt., 1993. – Vol.32. – P.399-410.
26.Çilesiz I.F., Welch A.J. Optical properties of human aorta: are they affected by cryopreservation? // Las.Surg.Med., 1994. – Vol.14. – P.396-402.
27.Yaroslavsky A.N., Yaroslavsky I.V., Goldbach T., Schwarzmaier H.-J. Optical properties of blood in the near-infrared spectral range. // Proc. SPIE, 1996. – Vol.2678. – P.314-324.
28.Yaroslavsky A.N., Yaroslavsky I.V., Goldbach T., Schwarzmaier H.-J. Different phase-function approximations to determine optical properties of blood: a comparison. // Proc. SPIE, 1997. –Vol.2982. – P.324-330.
82
29.Yaroslavsky A.N., Vervoorts A., Priezzhev A.V., Yaroslavsky I.V., Moser J.G., Schwarzmaier H.-J. Can Tumor Cell Suspension Serve as an Optical Model of Tumor Tissue in situ? // Proc. SPIE, 1999. – Vol.3565. – P.165-173.
30.H.J. Schwarzmaier, Yaroslavsky A.N., Yaroslavsky I.V., Goldbach Th., Kahn Th., Ulrich F., Schober R. The optical properties of native and coagulated human brain structures. // Proc.SPIE, 1997. – Vol.2970. – P.492-499.
31.Wan S., Anderson R.R., Parrish J.A. Analytical modeling for the optical properties of the skin with in vitro and in vivo applications. // Photochem. Photobiol., 1981.
– Vol.43. – P.493-499.
32.Van Gemert M.J.C., Berenbaum M.C., Gijsbers G.H.M. Wavelength and light– dose dependence in tumor phototherapy with hematoporphyrin–derivative. // Brit.J.Cancer, 1985. – Vol.52. – P.43-49.
33.Kubelka P. New contribution to the optics of intensively light–scattering materials. Part I. // J. Opt. Soc. Am., 1948. – Vol. 38(5). – P. 448-457.
34.Tuchin V.V., Utz S.R., Yaroslavsky I.V. Tissue optics, light distribution and spectroscopy. // Opt.Eng., 1994. – Vol.33. – P.3178-3188.
35.Wilson B.C., Patterson M.S., Flock S.T. Indirect versus direct techniques for the measurement of the optical properties of tissues. // Photochem.Photobiol., 1987. – Vol.46. – P.601-608.
36.Jacques S.L., Prahl S.A. Modeling optical and thermal distributions in tissue during laser irradiation. // Las.Surg.Med., 1987. – Vol.6. – P.494-503.
37.Prahl S.A. Light Transport in Tissue: PhD dissertation. – The University of Texas at Austin, Austin, Texas, 1988.
38.Ishimaru A. Wave Propagation and Scattering in Random Media. – New York: Academic Press, 1978. – Vol.1.
39.Cheong W.–F., Prahl S.A., Welch A.J. A review of the optical properties of biological tissues. // IEEE J.Quantum Electron., 1990. – Vol.26. – P.2166-2185.
40.Prahl S.A., van Gemert M.J.C., Welch A.J. Determining the optical properties of turbid media by using the adding–doubling method. // Appl.Opt., 1993. – Vol.32.
– P.559-568.
41.Graaf R., Koelink M.H., de Mul F.F.M., Zijlstra W.G., Dassel A.C.M. Condensed Monte Carlo simulations for the description of light transport. // Appl.Opt., 1993. – Vol.32. – P.426-434.
42.Van der Zee P. Methods for measuring the optical properties of tissue samples in the visible and near infrared wavelength range, in Medical Optical Tomography: Functional Imaging and Monitoring. // Chance B., Müller G.et al., eds. – Soc.Photo–Opt.Instrum.Eng. Institute Series, 1993. – Vol.11. – P.166-192.
43.Torres J.H., Welch A.J., Çilesiz I., Motamedi M. Tissue optical property measurements: overestimation of absorption coefficient with spectrophotometric techniques. // Las.Surg.Med., 1994. – Vol.14. – P.249-257.
83
44.Khlebtsov N.G. Role of multiple scattering in turbidimetric investigations of dispersed systems. // J.Appl.Spectrosc., 1984. – Vol.40. – P.243-247.
45.Wang L.H., Jacques S.L. Error estimation of measuring total interaction coefficients of turbid media using collimated light transmission. // Phys.Med.Biol., 1994. – Vol.39. – P.2349-2354.
46.Yaroslavsky I.V., Yaroslavsky A.N., Goldbach T., Schwarzmaier H.-J. Inverse hybrid technique for the determination of the optical properties of turbid media. // Appl.Opt., 1996. – Vol.35. – P.6797-6809.
47.Roggan A., Minet O., Shröder C., Müller G.J. Measurements of optical tissue properties using integrating sphere technique, in Medical Optical Tomography: Functional Imaging and Monitoring. // Chance B., Müller G. et al., eds. – Soc.Photo-Opt.Instrum.Eng. Institute Series, 1993. – Vol.11. – P.149-165.
48.Firbank M., Hiraoka M., Essenpreis M., Delpy D.T. Measurement of the optical properties of the scull in the wavelength range 650-950 nm. // Phys.Med.Biol, 1993. – V38. – P.503-510.
49.Verkruysse W., Nilsson A.M., Milner T.E., Beek J.F., Lucassen G.W., van Gemert M.J.C. Optical absorption of blood depends on temperature during a 5 ms laser pulse at 586 nm. // Photochem.Photobiol., 1998. – Vol.67(3). – P.276-281.
50.Glasser Z., Yaroshevsky A., Barak B., Granot E., Sternklar S. Effect of measurement on the ballistic-diffusive transition in turbid media // J. Biomed. Opt., 2013. - Vol. 18(10). – 106006.
51.W. F. Cheong, S. A. Prahl, and A. J. Welch, "A review of the optical properties of biological tissues" // IEEE Journal of Quantum Electronics, vol. 26, pp. 2166– 2185, 1990.
52.M. S. Patterson, B. C. Wilson, and D. R. Wyman, "The propagation of optical radiation in tissue II. Optical properties of tissues and resulting fluence distributions" // Lasers in Medical Science, vol. 6, pp. 379–390, 1991.
53.S. T. Flock, B. C. Wilson, and M. S. Patterson, "Total attenuation coefficients and scattering phase functions of tissues and phantom materials at 633 nm" // Medical Physics, vol. 14, pp. 835–841, 1987.
54.P. Kubelka, "New contributions to the optics of intensely light-scattering materials. Part I" // Journal of the Optical Society of America, vol. 38, pp. 448– 457, 1948.
55.P. Kubelka, "New contributions to the optics of intensely light-scattering materials. Part II: Nonhomogeneous layers" // Journal of the Optical Society of America, vol. 44, pp. 330–335, 1954.
56.W. G. Egan, T. W. Hilgeman, and J. Reichman, "Determination of absorption and scattering coefficients for nonhomogeneous media. 2: Experiment" // Applied Optics, vol. 12, pp. 1816–1823, 1973.
84
57.S. A. Prahl, I. A. Vitkin, B. C. Wilson, and R. R. Anderson, “Determination of optical properties of turbid media using pulsed photothermal radiometry,” Physics in Medicine and Biology, vol. 37, pp. 1203–1217, 1992.
58.H. C. van de Hulst, "A new look at multiple scattering" // Tech. rep., NASA Institute for Space Studies, New York, 1962.
59.Чандрасекар C. Перенос лучистой энергии. М.: ИЛ 1953г. 431 с. 60.Гермогенова Т.А. Локальные свойства решений уравнения переноса. – М.:
Наука, 1986.
61.Reynolds L.O., McCormick N.J. Approximate two–parameter phase function for light scattering. // J.Opt.Soc.Am., 1980. – Vol.70. – P.1206-1212.
62.Reynolds L., Johnson C., Ishimaru A. Diffuse reflectance from a finite blood medium: applications to the modeling of fiber optic catheters. // Appl.Opt., 1976. – Vol.15. – P.2059-2067.
63.Yaroslavsky A.N., Yaroslavsky I.V., Goldbach T., Schwarzmaier H.-J. Influence of the Scattering Phase Function Approximation on the Optical Properties of Blood Determined from the Integrating Sphere Measurements. // JBO, 1999. – Vol.4(01). – P.47-53.
64.Van de Hulst H.C. Multiple Light Scattering. – N.Y.: Academic Press, 1980. – Vol.2.
65.Van Gemert M.J.C., Schets G.A.C., Bishop M.S. et al. Optics of tissue in a multislab geometry //Laser Life Sci. .19881. N 2. P. 1-18.
66.Yoon G., Welch A.J., Motamedi M. et al. Development and Application of ThreeDimensional Light Distribution Model for Laser Irradiated Tissue.-IEEE Journal of Quantum Electronics // IEEE J. Quantum Electron. 1987. QE-23. N 10. P. 17211733.
67.Kubelka P., Munk F. Ein Beitrag zur Optik der Farbanstriche. - Zeitschrift fuer Technische Optik II a, 1931. – P.593-601.
68.К.М. Гираев, Н.А. Ашурбеков, О.В. Кобзев, Оптические исследования биотканей: определение показателей поглощения и рассеяния // Письма в ЖТФ, 2003, том 29, вып. 21. С. 48-54
69.Maitland D.J., Walsh J.T., Prystowsky J.B. Optical properties of human gallbladder tissue and bile // Appl. Optics. 1993. V. 32. N 4. P. 586-591.
70.Groenhuis R.A.J., Ferverda H.A., Ten Bosch J.J. Scattering and absorption of turbid materials determined from reflection measurements. 1: Theory // Appl. Optics. 1983. V. 22. N 16. P. 2456-2462.
71.Splinter R., Svenson R.H., Littmann L., Tuntelder J.R., Huang C.H., Tatsis G.P., Thompson M. Optical properties of normal, diseased, and laser photocoagulated myocardium at the Nd:YAG wavelength. // Las.Surg.Med. 1991. – Vol.11. – P.117-124.
85
72.Воронков А.В. и др., Технический справочник железнодорожника // т.1, – М.: Государственное транспортное железнодорожное издательство, 1951. – 254 с.
73.G. N. Plass, G. W. Kattawar, and F. E. Catchings, "Matrix operator theory of radiative transfer. 1: Rayleigh scattering" // Applied Optics, vol. 12, pp. 314–329, 1973.
74.S. Chandrasekhar, "Radiative Transfer" // New York: Dover, 1960.
75.S. E. Orchard, "Reflection and transmission of light by diffusing suspensions" // Journal of the Optical Society of America, vol. 59, pp. 1584–1597, 1969.
76.B. C. Wilson and G. Adam, "A Monte Carlo model for the absorption and flux distributions of light in tissue" // Medical Physics, vol. 10, pp. 824–830, 1983.
77.S. A. Prahl, M. Keijzer, S. L. Jacques, and A. J. Welch, "A Monte Carlo model of light propagation in tissue" // in Dosimetry of Laser Radiation in Medicine and Biology (G. J. Muller and D. H. Sliney, eds.), vol. IS 5, (Bellingham, WA), pp. 102– 111, SPIE Optical Engineering Press, 1989.
78.S. T. Flock, M. S. Patterson, B. C. Wilson, and D. R. Wyman, "Monte Carlo modeling of light propagation in high scattering tissue—I: Model predictions and comparison with diffusion theory" // IEEE Transactions of Biomedical Engineering, vol. BME-36, pp. 1162–1168, 1989.
79.Keijzer M., Jacques S.L., Prahl S.A., Welch A.J. Light distribution in artery tissue: Monte Carlo simulations for finite diameter laser beams. // Las.Surg.Med., 1989.
– Vol.9. – P.148-154.
80.Yaroslavsky I.V., Tuchin V.V. Light propagation in multilayer scattering media: modeling by the Monte Carlo method. // Opt. Spectrosc., 1992. – Vol.72. – P.505-509.
86

Учебное издание
Вячеслав Иванович Кочубей, Алексей Николаевич Башкатов
СПЕКТРОСКОПИЯ РАССЕИВАЮЩИХ СРЕД
Учебное пособие
Подписано в печать ____________. Формат 60х841/16 Бумага офсетная. Гарнитура Calibri. Печать офсетная. Усл. печ. л. ______
Тираж 100 экз. Заказ ______
Отпечатано в типографии «Новый ветер» 410012, Саратов, ул. Астраханская, 79