
- •Why CFD is Important for Modeling
- •How the CFD Module Helps Improve Your Modeling
- •Model Builder Options for Physics Feature Node Settings Windows
- •Where Do I Access the Documentation and Model Library?
- •Typographical Conventions
- •Quick Start Guide
- •Modeling Strategy
- •Geometrical Complexities
- •Material Properties
- •Defining the Physics
- •Meshing
- •The Choice of Solver and Solver Settings
- •Coupling to Other Physics Interfaces
- •Adding a Chemical Species Transport Interface
- •Equation
- •Discretization
- •Transport Feature
- •Migration in Electric Field
- •Reactions
- •Reactions
- •Initial Values
- •Initial Values
- •Boundary Conditions for the Transport of Concentrated Species Interface
- •Mass Fraction
- •Mass Fraction
- •Flux
- •Inflow
- •Inflow
- •No Flux
- •Outflow
- •Flux Discontinuity
- •Flux Discontinuity
- •Symmetry
- •Open Boundary
- •Physical Model
- •Transport Properties
- •Model Inputs
- •Fluid Properties
- •Diffusion
- •Migration in Electric Field
- •Diffusion
- •Model Inputs
- •Density
- •Diffusion
- •Porous Matrix Properties
- •Porous Matrix Properties
- •Initial Values
- •Initial Values
- •Domain Features for the Reacting Flow, Concentrated Species Interface
- •Boundary Conditions for the Reacting Flow, Concentrated Species Interface
- •Reacting Boundary
- •Inward Flux
- •Physical Model
- •Transport Properties
- •Fluid Properties
- •Migration in Electric Field
- •Porous Matrix Properties
- •Initial Values
- •Domain Features for the Reacting Flow, Diluted Species Interface
- •Boundary Conditions for the Reacting Flow, Diluted Species Interface
- •Pair and Point Conditions for the Reacting Flow, Diluted Species Interface
- •Multicomponent Mass Transport
- •Multicomponent Diffusion: Mixture-Average Approximation
- •Multispecies Diffusion: Fick’s Law Approximation
- •Multicomponent Thermal Diffusion
- •References for the Transport of Concentrated Species Interface
- •Domain Equations
- •Combined Boundary Conditions
- •Effective Mass Transport Parameters in Porous Media
- •Selecting the Right Interface
- •The Single-Phase Flow Interface Options
- •Laminar Flow
- •Coupling to Other Physics Interfaces
- •The Laminar Flow Interface
- •Discretization
- •The Creeping Flow Interface
- •Discretization
- •Fluid Properties
- •Fluid Properties
- •Mixing Length Limit
- •Volume Force
- •Volume Force
- •Initial Values
- •Initial Values
- •The Turbulent Flow, Spalart-Allmaras Interface
- •The Rotating Machinery, Laminar Flow Interface
- •Rotating Domain
- •Rotating Domain
- •Initial Values
- •Initial Values
- •Rotating Wall
- •Wall
- •Boundary Condition
- •Interior Wall
- •Boundary Condition
- •Inlet
- •Boundary Condition
- •Velocity
- •Pressure, No Viscous Stress
- •Normal Stress
- •Outlet
- •Boundary Condition
- •Pressure
- •Laminar Outflow
- •No Viscous Stress
- •Vacuum Pump
- •Symmetry
- •Open Boundary
- •Boundary Stress
- •Boundary Condition
- •Periodic Flow Condition
- •Flow Continuity
- •Pressure Point Constraint
- •Non-Newtonian Flow—The Power Law and the Carreau Model
- •Theory for the Pressure, No Viscous Stress Boundary Condition
- •Theory for the Laminar Inflow Condition
- •Theory for the Laminar Outflow Condition
- •Theory for the Slip Velocity Wall Boundary Condition
- •Theory for the Vacuum Pump Outlet Condition
- •Theory for the No Viscous Stress Condition
- •Theory for the Mass Flow Inlet Condition
- •Turbulence Modeling
- •Eddy Viscosity
- •Wall Functions
- •Initial Values
- •Wall Distance
- •Inlet Values for the Turbulence Length Scale and Intensity
- •Initial Values
- •The Spalart-Allmaras Turbulence Model
- •Inlet Values for the Turbulence Length Scale and Intensity
- •Pseudo Time Stepping for Turbulent Flow Models
- •References for the Single-Phase Flow, Turbulent Flow Interfaces
- •Selecting the Right Interface
- •Coupling to Other Physics Interfaces
- •Discretization
- •Fluid-Film Properties
- •Initial Values
- •Initial Values
- •Inlet
- •Outlet
- •Wall
- •Symmetry
- •Discretization
- •Initial Values
- •Initial Values
- •Fluid-Film Properties
- •Border
- •Inlet
- •Outlet
- •Conditions for Film Damping
- •The Reynolds Equation
- •Structural Loads
- •Gas Outflow Conditions
- •Rarefaction and Slip Effects
- •Geometry Orientations
- •References for the Thin-Film Flow Interfaces
- •Selecting the Right Interface
- •The Multiphase Flow Interface Options
- •The Relationship Between the Interfaces
- •Bubbly Flow
- •Coupling to Other Physics Interfaces
- •The Laminar Two-Phase Flow, Level Set Interface
- •Discretization
- •The Laminar Two-Phase Flow, Phase Field Interface
- •Domain Level Settings for the Level Set and Phase Field Interfaces
- •Fluid Properties
- •Mixing Length Limit
- •Initial Values
- •Initial Values
- •Volume Force
- •Volume Force
- •Gravity
- •Boundary Conditions for the Level Set and Phase Field Interfaces
- •Wall
- •Boundary Condition
- •Initial Interface
- •The Turbulent Flow, Two-Phase Flow, Level Set Interface
- •The Turbulent Two-Phase Flow, Phase Field Interface
- •Wall Distance Interface and the Distance Equation
- •Level Set and Phase Field Equations
- •Conservative and Non-Conservative Formulations
- •Phase Initialization
- •Numerical Stabilization
- •References for the Level Set and Phase Field Interfaces
- •Stabilization
- •Discretization
- •Level Set Model
- •Initial Values
- •Initial Values
- •Boundary Conditions for the Level Set Function
- •Inlet
- •Initial Interface
- •No Flow
- •Outlet
- •Symmetry
- •Discretization
- •Initial Values
- •Initial Values
- •Phase Field Model
- •Boundary Conditions for the Phase Field Function
- •Initial Interface
- •Inlet
- •Wetted Wall
- •Wetted Wall
- •Outlet
- •The Level Set Method
- •Conservative and Non-Conservative Form
- •Initializing the Level Set Function
- •Variables For Geometric Properties of the Interface
- •Reference for the Level Set Interface
- •About the Phase Field Method
- •The Equations for the Phase Field Method
- •Conservative and Non-Conservative Forms
- •Additional Sources of Free Energy
- •Variables and Expressions
- •Reference For the Phase Field Interface
- •The Laminar Bubbly Flow Interface
- •Reference Pressure
- •Discretization
- •The Turbulent Bubbly Flow Interface
- •Reference Pressure
- •Discretization
- •Fluid Properties
- •Slip Model
- •Initial Values
- •Initial Values
- •Volume Force
- •Volume Force
- •Gravity
- •Gravity
- •Mass Transfer
- •Mass Transfer
- •Boundary Conditions for the Bubbly Flow Interfaces
- •Wall
- •Liquid Boundary Condition
- •Gas Boundary Condition
- •Inlet
- •Liquid Boundary Condition
- •Gas Boundary Condition
- •Outlet
- •Liquid Boundary Condition
- •Gas Boundary Condition
- •Symmetry
- •Gas Boundary Conditions Equations
- •The Mixture Model, Laminar Flow Interface
- •Stabilization
- •Discretization
- •The Mixture Model, Turbulent Flow Interface
- •Stabilization
- •Mixture Properties
- •Mass Transfer
- •Mass Transfer
- •Initial Values
- •Initial Values
- •Volume Force
- •Volume Force
- •Gravity
- •Gravity
- •Boundary Conditions for the Mixture Model Interfaces
- •Wall
- •Mixture Boundary Condition
- •Dispersed Phase Boundary Condition
- •Inlet
- •Mixture Boundary Condition
- •Dispersed Phase Boundary Condition
- •Outlet
- •Mixture Boundary Condition
- •Symmetry
- •The Bubbly Flow Equations
- •Turbulence Modeling in Bubbly Flow Applications
- •References for the Bubbly Flow Interfaces
- •The Mixture Model Equations
- •Dispersed Phase Boundary Conditions Equations
- •Turbulence Modeling in Mixture Models
- •Slip Velocity Models
- •References for the Mixture Model Interfaces
- •Dispersed Phase
- •Discretization
- •Domain Conditions for the Euler-Euler Model, Laminar Flow Interface
- •Phase Properties
- •Solid Viscosity Model
- •Drag Model
- •Solid Pressure Model
- •Initial Values
- •Boundary, Point, and Pair Conditions for the Euler-Euler Model, Laminar Flow Interface
- •Wall
- •Dispersed Phase Boundary Condition
- •Inlet
- •Two-Phase Inlet Type
- •Continuous Phase
- •Dispersed Phase
- •Outlet
- •Mixture Boundary Condition
- •The Euler-Euler Model Equations
- •References for the Euler-Euler Model, Laminar Flow Interface
- •Selecting the Right Interface
- •The Porous Media Flow Interface Options
- •Coupling to Other Physics Interfaces
- •Discretization
- •Fluid and Matrix Properties
- •Mass Source
- •Mass Source
- •Initial Values
- •Initial Values
- •Boundary Conditions for the Darcy’s Law Interface
- •Pressure
- •Pressure
- •Mass Flux
- •Mass Flux
- •Inflow Boundary
- •Inflow Boundary
- •Symmetry
- •No Flow
- •Discretization
- •Fluid and Matrix Properties
- •Volume Force
- •Volume Force
- •Forchheimer Drag
- •Forchheimer Drag
- •Initial Values
- •Initial Values
- •Mass Source
- •Boundary Conditions for the Brinkman Equations Interface
- •Discretization
- •Fluid Properties
- •Porous Matrix Properties
- •Porous Matrix Properties
- •Forchheimer Drag
- •Forchheimer Drag
- •Volume Force
- •Volume Force
- •Initial Values
- •Initial Values
- •Boundary Conditions for the Free and Porous Media Flow Interface
- •Microfluidic Wall Conditions
- •Boundary Condition
- •Discretization
- •Domain, Boundary, and Pair Conditions for the Two-Phase Darcy’s Law Interface
- •Fluid and Matrix Properties
- •Initial Values
- •Initial Values
- •No Flux
- •Pressure and Saturation
- •Pressure and Saturation
- •Mass Flux
- •Inflow Boundary
- •Inflow Boundary
- •Outflow
- •Pressure
- •Darcy’s Law—Equation Formulation
- •About the Brinkman Equations
- •Brinkman Equations Theory
- •References for the Brinkman Equations Interface
- •Reference for the Free and Porous Media Flow Interface
- •Darcy’s Law—Equation Formulation
- •The High Mach Number Flow, Laminar Flow Interface
- •Surface-to-Surface Radiation
- •Discretization
- •Initial Values
- •Initial Values
- •Shared Interface Features
- •Fluid
- •Dynamic Viscosity
- •Inlet
- •Outlet
- •Consistent Inlet and Outlet Conditions
- •Pseudo Time Stepping for High Mach Number Flow Models
- •References for the High Mach Number Flow Interfaces
- •Selecting the Right Interface
- •The Non-Isothermal Flow Interface Options
- •Coupling to Other Physics Interfaces
- •The Non-Isothermal Flow, Laminar Flow Interface
- •Discretization
- •The Conjugate Heat Transfer, Laminar Flow Interface
- •The Turbulent Flow, Spalart-Allmaras Interface
- •Fluid
- •Dynamic Viscosity
- •Wall
- •Boundary Condition
- •Initial Values
- •Pressure Work
- •Viscous Heating
- •Dynamic Viscosity
- •Turbulent Non-Isothermal Flow Theory
- •References for the Non-Isothermal Flow and Conjugate Heat Transfer Interfaces
- •Selecting the Right Interface
- •The Heat Transfer Interface Options
- •Conjugate Heat Transfer, Laminar Flow
- •Conjugate Heat Transfer, Turbulent Flow
- •Coupling to Other Physics Interfaces
- •Accessing the Heat Transfer Interfaces via the Model Wizard
- •Discretization
- •Heat Transfer in Solids
- •Translational Motion
- •Translational Motion
- •Pressure Work
- •Heat Transfer in Fluids
- •Viscous Heating
- •Dynamic Viscosity
- •Heat Source
- •Heat Source
- •Initial Values
- •Initial Values
- •Boundary Conditions for the Heat Transfer Interfaces
- •Temperature
- •Temperature
- •Thermal Insulation
- •Outflow
- •Symmetry
- •Heat Flux
- •Heat Flux
- •Inflow Heat Flux
- •Inflow Heat Flux
- •Open Boundary
- •Periodic Heat Condition
- •Surface-to-Ambient Radiation
- •Boundary Heat Source
- •Boundary Heat Source
- •Heat Continuity
- •Pair Thin Thermally Resistive Layer
- •Pair Thin Thermally Resistive Layer
- •Thin Thermally Resistive Layer
- •Thin Thermally Resistive Layer
- •Line Heat Source
- •Line Heat Source
- •Point Heat Source
- •Convective Cooling
- •Out-of-Plane Convective Cooling
- •Upside Heat Flux
- •Out-of-Plane Radiation
- •Upside Parameters
- •Out-of-Plane Heat Flux
- •Domain Selection
- •Upside Inward Heat Flux
- •Change Thickness
- •Change Thickness
- •Porous Matrix
- •Heat Transfer in Fluids
- •Thermal Dispersion
- •Dispersivities
- •Heat Source
- •Equation Formulation
- •Activating Out-of-Plane Heat Transfer and Thickness
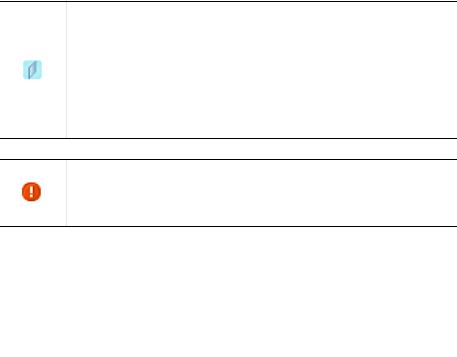
If Standard pressure and temperature is selected, enter:
-The Standard flow rate Qsv (SI unit: m3/s) to specify the standard volumetric flow rate through the boundary. The default value is 10 6 m3/s.
-The Standard pressure Pst (SI unit: Pa). The default value is 1 atm (101,325 Pa).
-The Standard temperature Tst (SI unit: K). The default value is 273.15 K (0 degrees Celsius).
-The Mean molar mass Mn (SI unit: kg/mol). This can be selected as a model input from another physics interface or set to a user-defined value using the associated
list and field. The default is that User defined is selected from the list with a value of 0.032 kg/mol.
For 2D models, enter a Channel thickness dbc (SI unit: m). The default value is 1 m.
The Channel thickness is used only in defining the area across which the mass flow occurs—it is not a setting applied to the whole model. Line
2D integrals of mass flux over the boundary in post-processing or coupling variables still produces results per unit thickness and needs to be scaled appropriately for comparison with the specified mass flow.
Remember to enter the settings as described in Additional Boundary
Condition Settings for Turbulent Flow Interfaces.
Important
C O N S T R A I N T S E T T I N G S
To display this section, click the Show button () and select Advanced Physics Interface Options. Select a Constraint type—Bidirectional, symmetric or Unidirectional. Select the Use weak constraints check box as required.
Outlet
The Outlet feature includes a set of boundary conditions describing fluid flow conditions at an outlet. The Pressure, no viscous stress boundary condition is the default.
B O U N D A R Y C O N D I T I O N S F O R T H E S I N G L E - P H A S E F L O W I N T E R F A C E S | 121
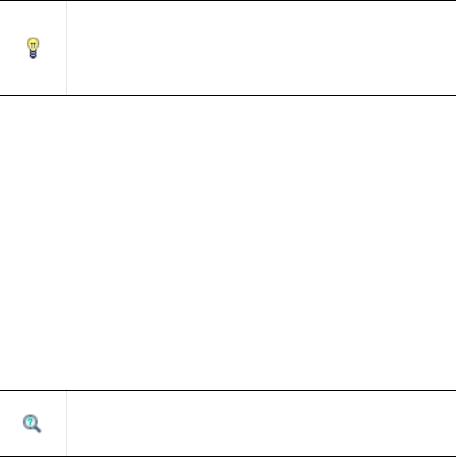
Selecting appropriate outlet conditions for the Navier-Stokes equations is not a trivial task. Generally, if there is something interesting happening at an outflow boundary, extend the computational domain to include this phenomenon.
All of the formulations for the Outlet type are also available, possibly slightly modified, in other boundary types as well. This means that there is nothing in the mathematical formulations to prevent a fluid from
Tip entering the domain through boundaries where the Outlet boundary type is specified.
•Velocity
•Pressure, No Viscous Stress
•Normal Stress
•Vacuum Pump
•Laminar Outflow
•Pressure
•No Viscous Stress
B O U N D A R Y S E L E C T I O N
From the Selection list, choose the boundaries that represent outlets.
B O U N D A R Y C O N D I T I O N
Select a Boundary condition for the outlet. Pressure, no viscous stress is the default. See Table 4-6 and Table 4-7 for available boundary conditions by interface.
See Pressure, No Viscous Stress, Velocity and Normal Stress boundary
conditions as described for the Inlet feature.
See Also
122 | C H A P T E R 4 : S I N G L E - P H A S E F L O W B R A N C H
TABLE 4-6: SINGLE-PHASE FLOW, LAMINAR OUTLET BOUNDARY CONDITIONS
|
|
BOUNDARY CONDITION |
|
|
|
|
|
|
|
|
|
|||||
|
INTERFACE |
|
|
|
|
|
|
|
|
|
|
|
|
|
|
|
|
|
|
|
|
|
|
|
|
|
|
|
|
|
|
|
|
|
|
VELOCITY |
|
PRESSURE, NO VISCOUS STRESS |
|
|
NORMAL STRESS |
|
LAMINAR |
OUTFLOW |
PRESSURE |
|
NO VISCOUS |
STRESS |
||
|
Laminar Flow |
|
|
|
|
|
|
|
|
|
|
|
|
|
|
|
|
|
|
|
|
|
|
|
|
|
|
|
|
|
|
|
|
|
Creeping Flow |
|
|
|
|
|
|
|
|
|
|
|
|
|
|
|
|
|
|
|
|
|
|
|
|
|
|
|
|
|
|
|
|
|
Rotating Machinery, |
|
|
|
|
|
|
|
|
|
|
|
|
|
|
|
|
Laminar Flow |
|
|
|
|
|
|
|
|
|
|
|
|
|
|
|
|
|
|
|
|
|
|
|
|
|
|
|
|
|
|
|
|
TABLE 4-7: SINGLE-PHASE FLOW, TURBULENT OUTLET BOUNDARY CONDITIONS |
|
|
|
|
|
|
||||||||||
|
|
|
|
|
|
|
|
|
|
|
|
|
|
|||
|
|
|
BOUNDARY CONDITION |
|
|
|
|
|
|
|
|
|
||||
INTERFACE |
|
VELOCITY |
|
PRESSURE, |
|
NORMAL |
|
PRESSURE |
|
NO |
||||||
|
|
|
|
|
||||||||||||
|
|
|
|
|
|
NO |
|
STRESS |
|
|
|
|
VISCOUS |
|||
|
|
|
|
|
|
VISCOUS |
|
|
|
|
|
|
|
STRESS |
||
|
|
|
|
|
|
STRESS |
|
|
|
|
|
|
|
|
|
|
Turbulent Flow, k- |
|
|
|
|
|
|
|
|
|
|
|
|||||
|
|
|
|
|
|
|
|
|
|
|
|
|||||
Turbulent Flow, k- |
|
|
|
|
|
|
|
|
|
|
|
|||||
|
|
|
|
|
|
|
|
|
|
|
|
|||||
Turbulent Flow, Low k- |
|
|
|
|
|
|
|
|
|
|
|
|||||
|
|
|
|
|
|
|
|
|
|
|
||||||
Turbulent Flow, Spalart-Allmaras |
|
|
|
|
|
|
|
|
|
|
||||||
|
|
|
|
|
|
|
|
|
|
|
||||||
Rotating Machinery, Turbulent |
|
|
|
|
|
|
|
|
|
|
||||||
Flow |
|
|
|
|
|
|
|
|
|
|
|
|
|
|
|
|
|
|
|
|
|
|
|
|
|
|
|
|
|
|
|
|
|
P R E S S U R E
This boundary condition prescribes only a Dirichlet condition for the pressure p = p0.
Enter the Pressure p0 (SI unit: Pa) at the boundary. While this boundary condition is flexible and seldom produces artifacts on the boundary (compared to Pressure, No Viscous Stress), it can be numerically unstable. Theoretically, the stability is guaranteed
by using streamline diffusion for a flow with a cell Reynolds number Rec u h 2 1 (h is the local mesh element size). It does however work well in
most other situations as well.
B O U N D A R Y C O N D I T I O N S F O R T H E S I N G L E - P H A S E F L O W I N T E R F A C E S | 123
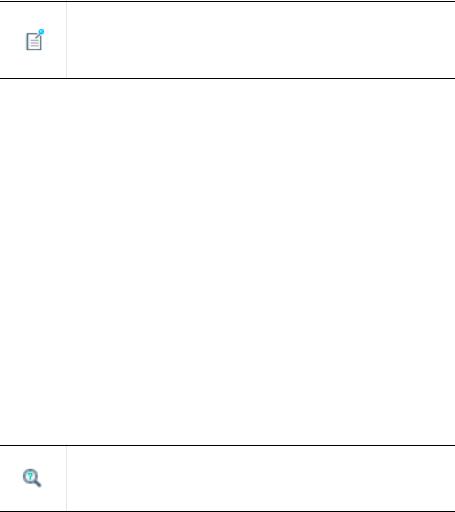
L A M I N A R O U T F L O W
This section displays when Laminar Outflow is selected as the Boundary condition for a Laminar Flow (or Creeping Flow) interface. However, it is
not available when the Use memory-efficient form check box is selected
Note
from Advanced Settings on the Laminar Flow Settings window.
Select a flow quantity to specify for the inlet:
•If Average velocity is selected, enter an Average velocity Uav (SI unit: m/s).
•If Flow rate is selected, enter the Flow rate V0 (SI unit: m3/s).
•If Exit pressure is selected, enter the Exit pressure pexit (SI unit: Pa) at the end of the fictitious channel following the outlet.
Then specify these parameters:
•Enter the Exit length Lexit (SI unit: m) to define the length of the fictitious channel after the model domain. This value must be large enough so that the flow can reach
a laminar profile. For a laminar flow, Lexit should be significantly greater than 0.06ReD, where Re is the Reynolds number and D is the outlet length scale (this
formula is exact if D is the diameter of a cylindrical pipe and approximate for other geometries).
•Select the Constrain endpoints to zero check box to force the laminar profile to go to zero at the bounding points or edges of the inlet channel. Otherwise the velocity is defined by the boundary condition of the adjacent boundary in the model.
For example, if one end of a boundary with a Laminar inflow condition connects to a Slip boundary condition, then the laminar profile has a maximum at that end.
• Theory for the Laminar Outflow Condition
See Also
N O V I S C O U S S T R E S S
The No Viscous Stress condition specifies vanishing viscous stress on the outlet. This condition does not provide sufficient information to fully specify the flow at the outlet and must be combined with pressure constraints on adjacent points.
124 | C H A P T E R 4 : S I N G L E - P H A S E F L O W B R A N C H