
Воробева Нуцлеар Реацтор Тыпес (Леарн то реад бы реадинг) 2010
.pdfREADING 11- B
Safety issues and more
Traditional reactor safety systems are 'active' in the sense that they involve electrical or mechanical operation on command. Some engineered systems operate passively, e.g. pressure relief valves. Both require parallel redundant systems. Inherent or full passive safety depends only on physical phenomena such as convection, gravity or resistance to high temperatures, not on functioning of engineered components.
Some are conceived for areas away from transmission grids and with small loads, others are designed to operate in clusters in competition with large units. US Congress is now funding research on both small modular nuclear power plants (assembled on site from factory-produced modules) and advanced gas-cooled designs (which are modular in the sense that up to ten or more units are progressively built to comprise a major power station).
Already operating in a remote corner of Siberia are four small units at the Bilibino co-generation plant. These four 62 MWt (thermal) units are an unusual graphite-moderated boiling water design with water/steam channels through the moderator. They produce steam for district heating and 11 MWe (net) electricity each. They have performed well since 1976, much more cheaply than fossil fuel alternatives in the Arctic region.
Light Water Reactors
US experience has been of very small military power plants. There was also an Army program for small reactor development and some successful small reactors from the main national program commenced in the 1950s. One was the Big Rock Point BWR of 67 MWe which operated for 35 years to 1997. The others mostly have the steam supply system inside the reactor pressure vessel ('integral' PWR design). All have enhanced safety features relative to current PWRs.
The Russian KLT-40S is a reactor well proven in icebreakers and now proposed for wider use in desalination and, on barges, for remote area power supply. These are designed to run 3 — 4 years between refueling and it is envisaged that they will be operated in pairs to allow for outages (70 % capacity factor), with on-board refueling capability and spent fuel storage. At the end of a 12-year operating cycle the whole plant is taken to a central facility for overhaul and storage of spent fuel. Two units will be mounted on a 20 000 tonne barge.
81
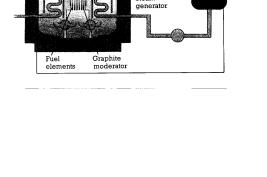
READING-11-C
Advanced Gas Cooled Reactor Thermal Reactor-Graphite Moderated
Indicative data for а reactor of 600 MW(е) size:
Uranium enrichment 2,3 % U235. Coolant outlet temperature 650 °С. Coolant pressure 600 psi. Steam cycle efficiency 42 %. Core dimensions 9,1 m dia × 8,5 m high.
AGRs form the second phase in Britain’s own nuclear development programme. Seven twin reactor stations are involved with а total capacity of 9000 MW(e)
Fuel: Uranium dioxide in -stainless steel cladding. Moderator: Graphite.
Core layout: Clusters of fuel elementa are joined together end-to- end in a stringer, рlасеd in vertical holes in the graphite.
Heat extraction: Carbon dioxide gas is heated by passing over the fuel in the core. It transfers its heat to water in а steam generator; the steam drives а turbme coupled to an electric generator.
Thermal Reactor Graphite Moderated Indicative data for a reactor of 600 MW(e) size:
Uranium enrichment 0.7 % U.235 (natural) Coolant outlet temperature 400 °C • Coolant pressure 300 psi Steam cycle efficiency 31 % Core dimensions 14m dia × 8m high.
82
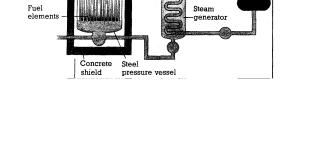
The first nuclear power station Calder Hall, is a 4 × 50 MW(e) Magnox type. Besides Calder and its sister station Chapelcross, Britain has eight twin reactor stations of this type. Two further stations were built overseas, one in Italy and one in Japan. Total Magnox capacity in the UK is over 3000 MW(e).
Fuel: Uranium metal. To conserve neutrons and allow natural uranium to be used, the fuel is clad in a magnesium alloy (Magnox) with low neutron absorption. Moderator: Graphite.
Heat extraction: Carbon dioxide gas is heated by passing over the fuel in the core. It transfers its heat to water in a steam generator, the steam drives a turbine coupled to an electric generator.
The long-delayed South African Pebble-Bed Modular Reactor (PBMR) Demonstration Power Plant is now expected to start construction at Koeberg in 2009 and achieve criticality in 2013 — possibly a few years behind the Chinese version. It will be for electricity production.
However, in 2006 the PBMR Board formalised the concept of a higher-temperature PBMR Process Heat Plant (PHP) with reactor output temperature of 950 °C. The first plants are envisaged for 2016 and the applications will be oil sands production and petrochemical industry (process steam), steam methane reforming for hydrogen and eventually thermochemical hydrogen production. This design is being submitted to US Department of Energy as a candidate Next-Generation Nuclear Plant.
Each 600 MWt module would produce about 200 tonnes of hydrogen per day.
83
Molten Salt Reactors
During the 1960s the USA developed the molten salt breeder reactor concept as the primary back-up option for the fast breeder reactor (cooled by liquid metal) and a small prototype MSR Experiment (8 MW) operated at Oak Ridge over four years. There is now renewed interest in the concept in Japan, Russia, France and the USA, and one of the six generation IV designs selected for further development is the MSR.
In the Molten Salt Reactor (MSR) the fuel is a molten mixture of lithium and beryllium fluoride salts with dissolved enriched uranium, thorium or U-233 fluorides. The core consists of unclad graphite moderator arranged to allow the flow of salt at some 700 °C and at low pressure. Heat is transferred to a secondary salt circuit and thence to steam. It is not a fast reactor, but with some moderation by the graphite is epithermal (intermediate neutron speed). The fission products dissolve in the salt and are removed continuously in an on-line reprocessing loop and replaced with Th-232 or U-238. Actinides remain in the reactor until they fission or are converted to higher actinides which do so. A fullsize 1000 MWe MSR breeder reactor was designed but not built. In 2002 a Thorium MSR was designed in France with a fissile zone where most power would be produced and a surrounding fertile zone where most conversion of Th-232 to U-233 would occur.
New reactor needed for medical imaging - why cyclotrons cannot do the job
It is claimed by opponents of the nuclear industry that Australia's demand for medical radioisotopes can be met by cyclotrons. The truth is that any number of cyclotrons will never replace Australia's need for a reactor.
Australia has two cyclotrons, which use high voltages and electrical fields to accelerate hydrogen atoms through a vacuum chamber. When they collide with a target substance they produce radioactivity.
As a general rule, it is more difficult to make a radioisotope in a cyclotron than in a reactor. Cyclotron reactions are less productive and less predictable than nuclear reactions performed in a reactor.
The cyclotron produces neutron-deficient radioisotopes whereas the reactor produces neutron-rich radioisotopes. Thus the reactor and the cyclotron complement each other in satisfying society's need for a full range of radioisotopes; rarely one acts as a substitute for the other.
84
A few radioisotopes are exceptions to this rule and can be produced by either facility. One is technetium-99m, currently used in 85 % of medical applications. The discovery that technetium-99m can be produced in a cyclotron does not imply that the need for a reactor is disappearing.
Economic factors would also militate against cyclotron-produced technetium-99m. The raw materials for reactor production are cheap (a few dollars per kilogram) and readily available, whereas the starting material for the cyclotron-method is a rare form of molybdenum that must be enriched to high levels of isotopic purity (>99 %), is not commercially available and would cost millions of dollars per kilogram.
The frontiers of nuclear medicine now extend beyond the diagnosis of disease with technetium-99m. Other short-lived radioisotopes are being introduced into nuclear medicine with the capability of reducing the pain associated with cancer. Australia must have its own reactor if its community is to have access to these radioisotopes and reap the benefits of the latest advances.
UNIT XII
FUEL
INTRODUCTION
This UNIT explains how nuclear fuel is made and about the different sorts of reactors it is produced for. They also explain what happens to the fuel once it has been used.
READING 12-A
Manufacturing Nuclear Fuel
•Fuel for nuclear reactors (power stations) is made at specially designed plants. These also produce Mixed Oxide (MOX) fuel (a mixture of uranium and plutonium). They have the ability to make fuel for all major designs of nuclear reactors.
•Fuel for nuclear reactors is made from uranium ore or a mixture of uranium and plutonium that is recycled from used nuclear fuel. The fuel is used in nuclear reactors to generate electricity. In 1999, on average,
85
around 16 % of the world's electricity supply was generated by nuclear power.
•Nuclear energy makes a significant contribution towards reducing greenhouse gas emissions. Globally, electricity supplied by nuclear power stations avoids the emission of around 2 billion tones of carbon dioxide annually.
•The raw material used to make nuclear fuel is uranium. Rock is taken from mainly open-cast mines all over the world. The ore that is taken contains around 1.5 % uranium. To concentrate the uranium content, the ore is ground, treated and purified using chemical and physical processes. This results in a solid uranium ore concentrate which contains around 80 % uranium.
•When the uranium ore arrives at a fuel manufacturing plant from mines around the world, it passes through a number of chemical processes that change it to uranium tetra fluoride (UF4). It then follows different processes depending on the type of fuel which is being produced.
Thorium-plutonium fuel
Since the early 1990s Russia has had a program to develop a tho- rium-uranium fuel, which more recently has moved to have a particular emphasis on utilisation of weapons-grade plutonium in a thoriumplutonium fuel.
The program is based at Moscow's Kurchatov Institute and involves the US company Thorium Power and US government funding to design fuel for Russian VVER-1000 reactors. Whereas normal fuel uses enriched uranium oxide, the new design has a demountable centre portion and blanket arrangement, with the plutonium in the centre and the thorium (with uranium) around it. The Th-232 becomes U-233, which is fissile — as is the core Pu-239. Blanket material remains in the reactor for 9 years but the centre portion is burned for only three years (as in a normal VVER). Design of the seed fuel rods in the centre portion draws on extensive experience of Russian navy reactors.
The thorium-plutonium fuel claims four advantages over MOX: proliferation resistance, compatibility with existing reactors — which will need minimal modification to be able to burn it, and the fuel can be made in existing plants in Russia — hence it could be used from 2006. In addition, a lot more plutonium can be put into a single fuel assembly than with MOX, so that three times as much can be disposed of as when using MOX. The spent fuel amounts to about half the volume of MOX
86
and is even less likely to allow recovery of weapons-useable material than spent MOX fuel, since less fissile plutonium remains in it. With an estimated 150 tonnes of weapons Pu in Russia, the thorium-plutonium project would not necessarily cut across existing plans to make MOX fuel.
READING 12-B
FUEL FOR:
Light Water Reactors
• Light Water Reactors (LWRs) are used throughout the world. Pressurized Water Reactors are a type of Light Water Reactor. They
are the most modern type of nuclear reactor to be used in the UK.
•The fuel for Light Water Reactors is a type of oxide fuel. The fuel elements from these reactors are made up of uranium dioxide fuel pellets stacked inside zirconium alloy fuel tubes. We then group these tubes together to form a fuel assembly. For example, a Sizewell B fuel assembly is made up of 264 zirconium alloy tubes, each containing about 300 pellets.
Advanced Gas-cooled Reactors
•The Advanced Gas-cooled Reactor (AGR) is unique to the UK and is the second type of nuclear reactor to be run in the UK. In all, 15 AGR reactors have been built and run in the UK since 1963. (All of the UK's AGR reactors are operated by a company called British Energy.)
•AGR fuel is also a type of oxide fuel and is made from uranium dioxide powder. An AGR fuel element is made up of uranium oxide pellets stacked inside stainless steel tubes. These tubes are then grouped together in a graphite 'sleeve' to form a 'fuel assembly'. An AGR assembly is made up of 36 steel tubes, each containing 64 pellets.
•AGR fuel uses the same manufacturing process as for LWR fuel. The fuel pellets (which are about the size of a thimble) are stacked inside a fuel tube.
•Once the tubes are sealed and pressurised, they are put together in the graphite 'sleeve' to form the AGR fuel assembly. After it is thoroughly inspected to check the quality of the fuel, we pack it ready to send to an AGR reactor.
Magnox reactors
•The name Magnox comes from the magnesium alloy casing which surrounds the fuel rod. Magnox reactors are the UK's pioneering nuclear
87
reactors and in all, 26 Magnox reactors have been built and run solely in the UK since 1956.
Calder Hall at Sellafield site was the world's first industrial-scale nuclear reactor.
A Magnox fuel element is made up of a uranium metal fuel rod which has a magnesium alloy can around it.
READING 12-C
Mixed Oxide (MOX) fuel
•Mixed Oxide (MOX) fuel is made up of plutonium (around 5 %) and uranium (around 95 %) both of which we can be recovered from reprocessing. This type of fuel manufacture produces fuel which can be burned in conventional nuclear reactors. About 400 tonnes of MOX fuel has been safely loaded into reactors around the world since 1963. More than 30 reactors in Europe are licensed to use MOX fuel and many reactors in the world plan to use this fuel, particularly in Japan.
•Using MOX fuel helps to manage the plutonium stockpile which would otherwise grow if we only used conventional uranium fuel in reactors. Typical MOX fuel might contain between about 50 and 70 kilograms of plutonium for each tonne of fuel entering the reactor. After four years of generating electricity, the amount of plutonium in the fuel would have been reduced by about 18 kilograms in each tonne of fuel.
Recycling nuclear fuel
•When the fuel rods in a nuclear reactor become less efficient they are removed and replaced with new fuel. A chemical operation called reprocessing which separates out around 96 % uranium and 1 % plutonium from the 3 % waste in the fuel is carried out.
•Reprocessing used nuclear fuel means that we can recycle the uranium and plutonium to make new fuel.
•Recycling uranium means that it is not necessary to mine as much new uranium. By recycling nuclear fuel we are making sure that this valuable energy source does not go to waste.
Summary
•We can make fuel for nuclear power stations from uranium and plutonium.
•The world's nuclear electricity supply avoids around 2 billion tonnes of CO2 being released into the air annually.
88
• Fuel for various reactor types like LWR, Magnox and AGR reactors as well as intermediate products are made.
•Dealing with notes
•Have fun and develop the notes on “Sex and Plutonium
SEX AND PLUTONIUM
Presentation for University Conference June 23, 1995 Philadelphia Alan E. Waltar, President American Nuclear Society
I) INTRODUCTION
- In thinking about this, I decided (after a good bit of hesitation) that I should entitle this talk, "Sex and Plutonium";
•Pu and sex both part of our everyday vocabulary;
•Both very much a powerful part of our life — inescapable
part;
•Both are emotional topics, subject to improper use, and potentially dangerous;
•In both cases, everybody considers themselves an expert;
-volumes of information available;
-too much either slanted, commercialized, or just plain incorrect;
•Both require a thoughtful education for wise use;
•Finally, and most importantly, both have a great deal to do with our quality of life, indeed the survival of life, in the 21st century;
-"Dean Henry and I are determined to stop kissing at campus".
II) JOYS AND PROBLEMS WITH SEX
А) Joys:
1)One of the most rewarding activities in life (but only if done under proper conditions);
2)Life-long enjoyment.
-NOTE: А1 Weinberg's 80th birthday party:
Father remarried but then divorced his wife for sexual incompatibility....at age 93!
89
В) Problems (most are emotional failures to accept reality):
1)Short-Term:
•Teenage Pregnancy;
•Unwanted pregnancy in or out of marriage;
•Basis for divorce; second only to money;
•Ruined careers, relationships, etc.;
•Venereal disease.
2)Life Threatening:
•AIDS;
•Jealousy.
3)Long-Term (subsequent generations):
•Children (from broken marriages);
•Sexual abuse.
С) Ban Sex?
•Given а11 these problems, WHY NOT BAN SEX? - Avoid а11 the above problems
•ANSWER:
1)Very rewarding and completely ok when practiced within given accepted rules (e.g. marriage);
2)Essential for survival of the species.
III) JOYS AND PROBLEMS WITH PLUTONIUM
А) Joys:
1)We love electricity.
-Name any major activity that does not rely, in some fashion, on electricity.
•Over one-third of the energy used in the United states is now in the form of electricity (and this % is constantly growing);
•America — more power from nuclear now than at time Eisenhower sighed Atoms for Peace.
90