
Воробева Нуцлеар Реацтор Тыпес (Леарн то реад бы реадинг) 2010
.pdfoperate at high enough temperature to permit a higher plant efficiency (see Appendix C) than water-cooled reactors can achieve. Sodium, however, is highly reactive chemically. At reactor operating temperatures, it burns if exposed to air; moreover, it reacts violently with water. Stringent efforts must be made to prevent any breaks or corrosion that lead to sodium leakage.
In all LMFBR designs, the sodium that cools the core is not used to raise the steam that drives the turbogenerators. Instead, there is an intermediate sodium loop which avoids the possibility of releasing radioactive sodium during any steam generator problem. This requires use of an intermediate heat exchanger as an intermediate heat exchanger as an interface between the primary and secondary sodium loops. It has the effect of more effectively isolating the primary sodium-filled reactor from any water. It does not, however, eliminate the difficulty of designing steam generators which effectively separate sodium and water.
Two basic types of LMBR are being considered. The form most favored by other countries is the “pot” (pool) type, in which not only the core, but also a number of other components are contained in the reactor vessel. (An example is the French Phenix reactor). The vessel is filled with sodium at roughly atmospheric pressure, in which are immersed the core, refueling machines, the primary coolant pump, and the intermediate heat exchanger, so that the entire primary sodium loop is contained in the same vessel. This assembly markedly reduces the amount of external piping. The alternative scheme, called the “loop” arrangement, developed is more similar to conventional LWR systems in that individual components of the heat transfer system are connected by pipes, and the reactor vessel only contains the core and associated equipment. Such a system is employed in the Clinch River breeder reactor design. In either arrangement of the primary system, the vessels containing primary components are surrounded by guard vessels, so that any rupture of the primary system does not lead to a large loss of sodium. Finally, in either, the secondary sodium proceeds from the intermediate heat exchanger to a steam generator, which produces steam for driving the turbines.
61
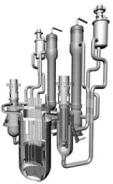
A fast breeder reactor concept (Mitsubishi Heavy Industries)
Schematic Liquid-Metal Fast
Breeder Reactor Power Plant
In an LMFBR, the core it cooled by liquid sodium. A heat exchanger transfers heat from this coolant to sodium in an intermediate loop. The sodium in this loop raises steam in a steam generator. This steam then drives the turbogenerator.
Exercises
Ex. 1. Answer the following questions.
1. What do the letters in LMFBR stand for? 2. That is the core arrangement in fast breeders? Compare it with light-water reactors. 3. What fissile and fertile material is used in the core and in the blanket? 4. What coolant has been chosen for LMFBR? Why? 5. What are advantages and disadvantages of sodium as a coolant? 6. Can you account for the necessity of a secondary sodium loop? 7. What is the function of the intermediate heat exchanger? 8. What are the two basic types of LMFBR? 9. What is the pot (pool) arrangement? 10. That is the loop arrangement? 11. Is the French Phenix reactor of the loop type? Is the Clinch River reactor of the pot (Pool) type?
Ex. 2. Translate the attributive groups.
Large fission cross sections; low neutron energies; heat transfer system; a higher plant efficiency.
Intermediate heat exchanger; sodium-filled reactor; the entire primary sodium loop breeder reactor design.
Reactor operating temperatures; blanket assemblies; sodium leakage; core assemblies; neutron escape; power generation; a team generator problem; good heat transfer properties.
62
Ex. 3. Translate. Pay attention to the underlined words.
1. The core is a compact arrangement of fuel assemblies that are similar to those used in light water reactors. 2. The cladding is stainless steel rather than zircaloy. 3. Instead of using 235U as the fissile material, the present choice is to load the core with 239Pu. 4. The sodium that cools the core is not used to raise steam. Instead, there is an intermediate sodium loop. 5. Because of its high value of eta 239Pu is much more profitably used in the breeder than in thermal reactors. 6. This nuclide (233U) is not presently being produced in any large quantity. 7. Sodium burns if exposed to air. 8. Sodium can be used at essentially atmospheric pressure, thereby making the design easier. 9. In either arrangement the vessels are surrounded by guard vessels. 10. Other fuel forms including both carbides and metal may be used in more advanced designs. 11. Conversion would occur in both regions. 12. In either case little moderation occurs prior to absorption by the fuel.
Ex. 4. Translate. Pay attention to the Infinitive Constructions.
1. Nuclear reactors are known to be capable of generating a lot of energy. 2. The control proves to be provided by the production of delayed neutrons. 3. A more sophisticated theory seems to be required. 4. The potential effects of accidents at NPPs do not appear to be larger than those at other facilities. 5. The materials of fusion devices are likely to be deuterium and tritium. 6. Ultracold neutrons are certain to be reflected from a surface no matter what the angle of incidence is. 7. One can excite the nucleus and observe it emit radiation. 8. The t° — resistant graphite core structure allows steam to be generated at the high temperature and pressures found in the modern fossil-fueled electric power plants. 9. A halflife is the time it takes for half of the nuclei in a sample to decay. 10. The doubling time is the period required for the reactor system to double the amount of fissile material. 11. For the fuel assemblies to have a longer lifetime in the reactor, the burnup must be higher, around 10 %. 12. The concentration of 235U has to be increased from its natural 0.7 % to almost 3 % in order for such a reactor to operate. 13. With a helium-cooled reactor more than an hour would be required for the t° inside the core to reach 3,000 degrees F and at that t° the coated fuel elements would not be affected.
63
Ex. 5. Condense the following sentences according to the model using the Infinitive Constructions instead of subordinate clauses.
Model: It is proved that energy consumption affects the living standard of any nation.
Energy consumption is proved to affect the living standard of any nation.
1. It is not expected that 235U yields enough neutrons for a practical breeder. 2. It was reported that 233U was not produced in any large quantity. 3. It is expected that 238U will produce more than enough 239Pu to replace that which is consumed in the core. 4. It is likely that 238U will be the basic nuclear fuel. 5. It is certain that regular reprocessing is necessary to recover the bred fissile material. 6. It is reported that the blanket assemblies have rods with the same composition throughout. 7. It turns out that the entire core is surrounded with blanket regions. 8. It is estimated that power generation in the core of a breeder will be more intense, compared with thermal reactors. 9. It proves that sodium successfully cools the very compact core. 10. However, it is proved that sodium is highly reactive chemically. 11. It is certain that the metal reacts violently with water.
Ex.6. Give a free translation.
Франция заинтересована в разработке бридеров. Разработка бридеров началась во Франции почти четверть века назад. Программа осуществлялась в три стадии, которые привели к созданию все более мощных реакторов.
Первым важным шагом во французской программе был экспериментальный реактор Rapsodie. Он имел мощность в 40 MWt и не вырабатывалэлектричества, тепловыделялосьватмосферу.
За экспериментальным реактором Rapsodie последовала демонстрационная установка Phenix: 250 MWt реактор бассейнового типа с тремя вторичными контурами.
Опыт, полученный на двух предыдущих реакторах, послужил основой для разработки полупромышленной («near-commercial)
установки Superphenix: 1200 MWt. Superphenix служит подтвер-
ждением накопленного опыта и технологии в промышленных масштабах.
64
READING 8-B
An important question for fast breeders is that of fuel lifetime. In thermal reactors, only a small percentage of the fuel atoms fission before the fuel is removed for storage or reprocessing. In fact, since the fissile load la typically less than 4 % of the fuel, and conversion ratios are small, it is clear that the “burn-up” is only 2 or 3 %. At burnups more than this, the fuel material and the cladding may suffer enough damage to prevent continued use of the fuel. But the breeder has a fissile loading of 15 % or more, and the power density (and, correspondingly, the per volume rate at which nuclei fission) is much greater than in water-cooled reactors. For the fuel assemblies to have a longer lifetime in the reactor, .the burnup that they can tolerate must be higher, around 10 %.
The United States has 15 % as the maximum burnup that fuel assemblies should stand, corresponding to a thermal energy generation of about 150,000 MWd/Te. A particular consideration with such high burnup is that the stainless steel cladding may begin to swell and distort, thus damaging the fuel rods and even blocking the coolant flow. The relatively compact LMFBR core implies a greater neutron flux through the core structure than typically occurs in thermal reactors, giving rise to the possibility of swelling and alteration of the core configuration over a period of time. Restraints are typically included in the LMFBR core design in order to prevent such changes.
Learn the terms:
1)fuel assemblies fuel bundles
2)fuel pins
fuel rods
3)coolant pump
4)primary circuit secondary circuit external circuits
-топливные сборки (с чехлом)
-топливные сборки (без чехла)
-топливные стержни
-насос для теплоносителя
-первичный контур
-вторичный контур
-внешние контуры
65
5)power generation den-
sity
6)top head of the reactor vessel
7)core barrel
8)pressurizer
9)containment building
10)steel-lined reinforced concrete
11)spray
12)leak
leakage
13) emergency
emergency core cooling system (ECCS)
14)redundant systems redundancy
15)loss-of-coolant accident (LOCA)
blowdown
16)Psi
-плотность энерговыделения
-верхняя крышка корпуса реактора
-корзина активной зоны
-компенсатор объема
-противоаварийная, герметичная оболочка(защита)
-армированный бетон
-сопло
-утечка
-аварийная ситуация
-аварийная система охлаждения активной зоны
-избыточные, дублирующие системы
-избыточность
-авария с потерей теплоносителя
-быстрая потеря теплоносителя
-pound per square inch
II. Active Vocabulary
1)to consider full-sized (scaled-down) system
2)to provide
to provide for
to have provision for control rods
3)to manipulate (handle) control rods
4)to remove radioactive materials (water droplets, etc.)
66
READING 8-C
Why is control necessary? First, because it is not possible to design a reactor so that the number of neutrons in successive generations is exactly constant. Therefore, extra fissile material is included in the fuel, and control elements remove enough neutrons to maintain a balance. This extra fissile material is needed, in any case, because over a long period of time, enough fissile material would be destroyed to turn the reactor off. Control mechanisms are needed because, in their absence, the extra fissile material at the beginning of operation would cause too great a portion of the neutrons to induce fission. As fissile material is “burned”, control is withdrawn. This control is necessary for other reasons, the most prominent of which is the buildup of neutron poisons as a result of reactor operation - many of the nuclear reactions occurring in the core, in particular fission, produce nuclei that absorb neutrons with no useful result. Enough control must exist when the reactor is turned on so that it can be reduced as this buildup of absorptive capacity occurs. A particularly important and interesting example of the need for control because of fission product poisoning is the so-called “xenon transient” to which reactors undergo during changes in power level.
One universal form of control is to provide a number of rods, loaded with neutron-absorbing elements such as boron or cadmium, which can be moved in and out of the core to select the portion of neutrons absorbed. In addition, reactors may have “burnable poisons” as part of the core - nuclei that absorb neutrons and, having done so, are neutralized. Moreover, poisons are commonly added to the cooling fluid. A number of other control mechanisms are sometimes available, particularly for use in emergencies.
67
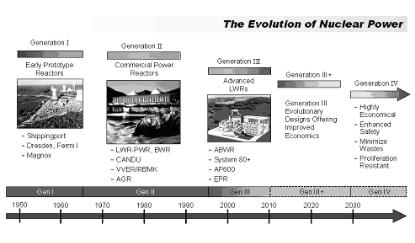
UNIT IX
ADVANCED REACTORS
READING 9-A
What is Generation IV
Today there are 441 nuclear power reactors in operation in 31 countries around the world. Generating electricity for nearly 1 billion people, they account for approximately 17 percent of worldwide electricity generation and provide half or more of the electricity in a number of industrialized countries. Another 32 are presently under construction overseas. Nuclear power has an excellent operating record and generates electricity in a reliable, environmentally safe, and affordable manner without emitting noxious gases into the atmosphere.
The Evolution of Nuclear Power
Concerns over energy resource availability, climate change, air quality, and energy security suggest an important role for nuclear power in future energy supplies. While the current Generation II and III nuclear power plant designs provide a secure and low-cost electricity supply in many markets, further advances in nuclear energy system design can broaden the opportunities for the use of nuclear energy. To explore these opportunities, the U.S. Department of Energy's Office of Nuclear Energy, Science and Technology has engaged governments, industry, and
68
the research community worldwide in a wide ranging discussion on the development of next generation nuclear energy systems known as "Generation IV".
Generation IV Nuclear Reactors
•An international task force is developing six nuclear reactor technologies for deployment between 2020 and 2030. Four are fast neutron reactors.
•All of these operate at higher temperatures than today's reactors. In particular, four are designated for hydrogen production.
•All six systems represent advances in sustainability, economics, safety, reliability and proliferation-resistance.
The Generation IV International Forum (GIF) was initiated in 2000, it is committed to joint development of the next generation of nuclear technology. USA Argentina, Brazil, Canada, France, Japan, South Korea, South Africa, Switzerland, and the UK are members of the GIF, along with the EU. Russia and China were admitted in 2006.
Late in 2002 GIF announced the selection of six reactor technologies which they believe represent the future shape of nuclear energy. These are selected on the basis of being clean, safe and cost-effective means of meeting increased energy demands on a sustainable basis, while being resistant to diversion of materials for weapons proliferation and secure from terrorist attacks. They will be the subject of further development internationally.
In addition to selecting these six concepts for deployment between 2010 and 2030, the GIF recognized a number of International NearTerm Deployment advanced reactors available before 2015.
Most of the six systems employ a closed fuel cycle to maximize the resource base and minimize high-level wastes to be sent to a repository. Three of the six are fast reactors and one can be built as a fast reactor, one is described as epithermal, and only two operate with slow neutrons like today's plants.
Only one is cooled by light water, two are helium-cooled and the others have lead-bismuth, sodium or fluoride salt coolant. The latter three operate at low pressure, with significant safety advantage. The last has the uranium fuel dissolved in the circulating coolant. Temperatures range from 510 to 1000 °C, compared with less than 330 °C for today's light water reactors, and this means that four of them can be used for thermo chemical hydrogen production.
69
READING 9-B
GIF Reactor technologies Gas-cooled fast reactors
Like other helium-cooled reactors which have operated or are under development, these will be high — temperature units — 850 °C, suitable for power generation, thermochemical hydrogen production or other process heat. For electricity, the gas will directly drive a gas turbine (Brayton cycle). Fuels would include depleted uranium and any other fissile or fertile materials. Spent fuel would be reprocessed on site and all the actinides recycled to minimise production of long-lived radioactive wastes.While General Atomics worked on the design in the 1970s (but not as fast reactor), none has so far been built.
Lead-cooled fast reactors
Liquid metal (Pb or Pb-Bi) cooling is by natural convection. Fuel is depleted uranium metal or nitride, with full actinide recycle from regional or central reprocessing plants. A wide range of unit sizes is envisaged, from factory-built "battery" with 15 — 20 year life for small grids or developing countries, to modular 300 — 400 MWe units and large single plants of 1400 MWe. Operating temperature of 550 °C is readily achievable but 800 °C is envisaged with advanced materials and this would enable thermochemical hydrogen production.
This corresponds with Russia's BREST fast reactor technology which is lead-cooled and builds on 40 years experience of lead-bismuth cooling in submarine reactors. Its fuel is U+Pu nitride. More immediately the GIF proposal appears to arise from two experimental designs: the US STAR and Japan's LSPR, these being lead and lead-bismuth cooled respectively.
Molten salt reactors
The uranium fuel is dissolved in the sodium fluoride salt coolant which circulates through graphite core channels to achieve some moderation and an epithermal neutron spectrum. Fission products are removed continuously and the actinides are fully recycled, while plutonium and other actinides can be added along with U-238. Coolant temperature is 700 °C at very low pressure, with 800 °C envisaged. A secondary coolant system is used for electricity generation, and thermo chemical hydrogen production is also feasible.
70