
Воробева Нуцлеар Реацтор Тыпес (Леарн то реад бы реадинг) 2010
.pdf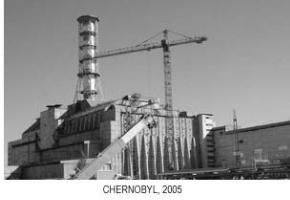
Annex 7
Chernobyl — Myths and Reality
The 1986 nuclear disaster at Chernobyl in Soviet Ukraine — the only nuclear power accident ever to harm the public — spawned widespread fears about the safety of nuclear power. But the Chernobyl reactor had an acutely flawed design — one which would never have been allowed to be built outside the Soviet Union. It also had weak safety features that failed to guard against human error.
In contrast, the U.S. Three Mile Island accident, which harmed no one, was confined by the extensive protective systems that are now the worldwide industry standard. Reactors with Chernobyl's severe shortcomings have been eliminated or improved — and will never be built again.
Using the world's top experts, the UN has conducted exhaustive studies of the health effects of Chernobyl — beyond the original death toll of 31. Of around 4000 thyroid cancer cases attributed to the accident, nearly all were successfully treated. Beyond this — after 20 years — there is no scientific evidence of any increase in cancer incidence at locations near or far. Theoretical projections of Chernobyl's possible longterm effects predict 4000 late-in-life cancer deaths. Any such increase would be too small to confirm statistically.
The UN's authorative findings do not minimise the gravity of what happened at Chernobyl. But they do refute many sensationalized reports and help to place that singular event in perspective. Coal-mining acci-
181
dents and gas explosions account for thousands of fatalities each year. Ironically, these deaths are so common that they generally go unreported. For example, a single mining accident killing scores of people may occur with little note, even while causing more fatalities in a day than have occurred in the full history of nuclear power.
The greatest health impact from over-use of fossil fuel comes from air pollution. The World Health Organisation (WHO) estimates that such pollution causes nearly three million deaths each year. Medical scientists predict that the fossil fuel mortality rate will triple by the year 2025. These devastating health effects — which equate to 600 'pollution Chernobyls' each day in the near future — overwhelm even the most distorted myths about nuclear power.
Annex 8
RBMK Reactors
•The RBMK is an unusual reactor design, one of two to emerge in the Soviet Union.
•The design has core characteristics which make it prone to power surges.
•It has no full containment structure.
•Major modifications have been made to RBMK reactors still operating.
The Soviet designed RBMK (high-power channel reactor — reactor bolshoy moshchnosty kanalny) is a pressurised water reactor with individual fuel channels and using ordinary water as its coolant and graphite as its moderator. It is very different from most other power reactor designs as it derived from a design principally for plutonium production and was intended and used in Russia for both plutonium and power production.
The combination of graphite moderator and water coolant is found in no other power reactors. The design characteristics of the reactor mean that it is unstable at low power levels, and this was shown in the Chernobyl accident. The instability was due primarily to control rod design and a positive void coefficient. A number of significant design changes have now been made to address these problems.
182
Features of the RBMK
Fuel: Pellets of slightly-enriched uranium oxide are enclosed in a zircaloy tube 3.65 m long, forming a fuel rod. A set of 18 fuel rods is arranged cylindrically in a carriage to form a fuel assembly. Two of these end on end occupy each pressure tube.
Pressure tubes: Within the reactor each fuel assembly is positioned in its own vertical pressure tube or channel about 7 m long. Each channel is individually cooled by pressurised water which is allowed to boil in the tube and emerges at about 290 В °C.
Refuelling: When fuel channels are isolated, these fuel assemblies can be lifted into and out of the reactor, allowing fuel replenishment while the reactor is in operation.
Graphite moderator: A series of graphite blocks surround, and hence separate, the pressure tubes. They act as a moderator to slow down the neutrons released during fission so that a continuous fission chain reaction can be maintained. Conductance of heat between the blocks is enhanced by a mixture of helium and nitrogen gas.
Control rods: Boron carbide control rods absorb neutrons to control the rate of fission. A few short rods, inserted upwards from the bottom of the core, even the distribution of power across the reactor. The main control rods are inserted from the top down and provide automatic, manual or emergency control. The automatic rods are regulated by feedback from in-core detectors. If there is a deviation from normal operating parameters (e.g. increased reactor power level), the rods can be dropped into the core to reduce or stop reactor activity. A number of rods normally remain in the core during operation.
Coolant: Two separate water coolant systems each with four pumps circulate water through the pressure tubes to remove most of the heat from fission. There is also an emergency core cooling system which will come into operation if either coolant circuit is interrupted.
Steam separator: Steam from the heated coolant is fed to turbines to produce electricity in the generator. The steam is then condensed and fed back into the circulating coolant.
Containment: There is no secure containment in the sense accepted in the West. The reactor core is located in a concrete lined cavity that acts as a radiation shield. The upper shield or pile cap above the core, is made of steel and supports the fuel assemblies. The steam separators of the coolant systems are housed in their own concrete shields.
183
Immediate Safety Changes
After the accident at Chernobyl unit 4, the primary concern was to reduce the positive void coefficient. All operating RBMK reactors, in the former Soviet Union therefore, had the following changes implemented to improve operating safety:
•To improve the operational reactivity margin, the effective number of manual control rods was increased from 30 to 45.
•The installation of 80 additional absorbers in the core to inhibit operation at low power.
•An increase in fuel enrichment from 2 to 2.4 % to maintain fuel burnup with an increase in neutron absorption (ie less reliance on cooling water for this).
These factors have reduced the positive void coefficient from +4.5 beta to +0.7 beta, eliminating the possibility of power excursion. Beta is the delayed neutron fraction, which is neutrons emitted from each fission with a measurable time delay.
The next consideration was to reduce the time taken to shut the reactor down and eliminate the positive void reactivity. Improvements include:
•Scram (rapid shut down) rod insertion time cut from 18 to 12
seconds.
•The redesign of control rods.
•The installation of a fast scram system.
•Precautions against unauthorised access to emergency safety systems.
Many — 179 of 211 — control rods are inserted into the core from the top. To improve their effectiveness, they are equipped with "riders" fixed to their bottom end but with a gap between the rider and the bottom tip of the control rod. Approximately 1.0 m water columns remained under and above it. When the control rod is in its uppermost position, the rider is in the control rod cooling tube within the fuelled region of the core. The rider being made substantially of graphite, is almost transparent to neutrons, while water, which would occupy the tube otherwise, plays as an absorber. When the reactor is "poisoned" with xenon and with partially inserted control rods, the major part of the power is produced within the lower region of the core. This means that when the rod started to move down from its uppermost position, the rider removed water from the lower part, causing an increase in reactivity and hence in power.
184
Longer-term modifications
In addition to the safety changes, RBMKs were modified more fundamentally. Chernobyl unit 1 was relicensed for operation in October 1995, following extensive maintenance which included the removal of some fuel channels to evaluate the metal and some backfitting as follows. The modification process consisted of:
•Replacement of the fuel channels at all units except Smolensk-
3.
•Replacement of the group distribution headers and addition of check valves.
•Improvements to the emergency core cooling systems.
•Improvements of the reactor cavity over-pressure protection systems.
•Replacement of the process computer, SKALA.
Positive void coefficient
Positive void coefficient is a term often associated with the RBMK reactors, the type involved in the Chernobyl disaster. Reactors that have a positive void coefficient can be unstable at low power and are prone to experiencing a rapid, uncontrollable power increase. While some reactors other than the RBMK type have positive void coefficients, they incorporate design features to prevent such instability from occurring.
In a water cooled reactor, steam may accumulate to form pockets, known as voids. If excess steam is produced, creating more voids than normal, the operation of the reactor is disturbed, because
•the water is a more efficient coolant than steam
•the water acts as a moderator (to enhance the fission reaction) and neutron absorber (hindering it) while steam barely does so.
A reactor is said to have a positive void coefficient if excess steam voids lead to increased power generation, and a negative void coefficient if excess steam voids leads to a decrease in power. The quantum of the coefficient is simply a measure of the speed of change of state of the reactor.
When the void coefficient is strongly positive, the power can increase very rapidly because any power increment that occurs leads to increased steam generation, which in turn leads to a further increase in power. Such positive feedback processes are, therefore, very difficult to control.
185
When the void coefficient is negative, excess steam generation will tend to shut down the reactor. This is, of course, is a safety feature.
Most of the world's operating power reactors have negative void coefficients. In those reactors where same water circuit acts as both moderator and coolant, excess steam generation reduces the slowing of neutrons necessary to sustain the nuclear chain reaction. This leads to a reduction in power.
In some reactor designs however, the moderator and coolant are in separate circuits, or are of different materials. In these reactors, excess steam reduces the cooling of the reactor, but as the moderator remains intact the nuclear chain reaction continues.
In some of these reactors, most notably the RBMK, the neutron absorbing properties of the cooling water are a significant factor in the operating characteristics. In such cases, the reduction in neutron absorption as a result of steam production, and the consequent presence of extra free neutrons, enhances the chain reaction. This increases power production which causes additional heating. The additional heat raises the temperature in the cooling circuit and more steam is produced. More steam means less cooling and less neutron absorption, and the problem gets worse.
This positive feedback can occur very rapidly, potentially leading to a serious accident such as at Chernobyl unit 4 in 1986 where the power peaked at several hundred times the normal full rating. The coolant vapourised and reacted with the zirconium cladding to produce hydrogen which then exploded and destroyed the core, dispersing about 5 % of it to the outside environment.
In order to avoid problems with positive void coefficient there are two approaches. Either the reactor characteristics can be altered to reduce the positive void coefficient, or systems can be provided that will shut the reactor down very quickly if an increase in power is detected. Since the Chernobyl disaster, the RBMK reactor design has been altered and units have been equipped to protect them against the runaway effects of the positive void coefficient.
There are currently 12 operating RBMKs in the world, in Russia and Lithuania. One more is under construction in Russia (Kursk-5), the completion of which is unlikely. All operating RBMKs began operation between 1973 (Leningrad-1) and 1990 (Smolensk-3). There are currently
186
three distinct generations of reactors having significant differences with respect to their safety design features:
•The four first-generation units are Leningrad-1 and -2, and Kursk-1 and -2. They were designed and brought on line in the early-to- mid 1970s, before new standards on the design and construction of nuclear power plants (OPB-82) were introduced in the Soviet Union.
•Second-generation RBMKs, brought on line since the late 1970s and early 1980s include Leningrad-3 and -4; Kursk-3, and -4; Ignalina-1 (now closed); and Smolensk-1 and -2. Ignalina-2 contains safety features beyond those of other second generation units. These units conform to updated standards issued in 1982.
•After the Chernobyl accident, Soviet safety standards were revised again (OPB-88). One RBMK (Smolensk-3) has been built to these third-generation standards. Additional design changes were being incorporated in the construction of Kursk-5.
The 'operating till' dates in the following table are the scheduled shut down for these plants, with 15 year life extensions in some cases. Lithuania, on the other hand, closed Ignalina-1 early as a condition for entry into the European Union and will close unit 2 in 2009.
Russia's long-term plans earlier included the possibility of replacing the Leningrad units, at the end of their extended service life, by new MKER-1000 units. These are a modification of the RBMK design. The main differences are in the spacing of the graphite lattice in the core and the incorporation of passive safety systems.
In 2006 Rosatom said it was considering lifetime extensions and uprating of its eleven operating RBMK reactors. Following significant design modifications made after the Chernobyl accident, as well as extensive refurbishment including replacement of fuel channels, a 45-year lifetime is seen as realistic for the 1000 MWe units. In 2005 they provided 48 % of Russia's nuclear-generated electricity. The R&D Institute of Power Engineering is preparing plans for 5 % uprating of them.
187
Location |
Unit |
Commercial |
Unit net capacity |
|
Operation |
(MWe) |
|||
|
|
|||
Lithuania |
В |
В |
В |
|
Ignalina |
1 |
1984 |
1250 |
|
|
2 |
1987 |
1250 |
|
Russia |
|
|
|
|
Kursk |
1 |
1977 |
700 |
|
|
2 |
1979 |
700 |
|
|
3 |
1984 |
950 |
|
|
4 |
1986 |
950 |
|
|
5 |
- |
950 |
|
Leningrad |
1 |
1974 |
950 |
|
|
2 |
1976 |
950 |
|
|
3 |
1980 |
950 |
|
|
4 |
1981 |
950 |
|
Smolensk |
1 |
1983 |
950 |
|
|
2 |
1990 |
950 |
|
|
3 |
1990 |
950 |
Status
В
Closed
Operating till 2009
В
Operating till 2022 Operating to 2024
Operating till March 2014
Operating till February 2016
Construction suspended
Operating till 2019 Operating till 2021
Operating till June 2010
Operating till August 2011
Operating till December 2013
Operating till July 2015
Operating till July 2023
Annex 9
Hiroshima, Nagasaki, and Subsequent Weapons Testing
•Two atomic bombs made by the allied powers (USA and UK) from uranium-235 and plutonium-239 were dropped on Hiroshima and Nagasaki respectively early in August 1945. These brought the long Second World War to a sudden end.
•The immense and previously unimaginable power of the atom had been demonstrated. In the following years several governments
188
joined the arms race, while internationally, efforts were focussed on constraining the threat of nuclear weapons proliferation.
•But from the 1950s the power of the atom was harnessed increasingly for peaceful uses, notably electricity generation and medicine. Nowhere is the transition from weapons of destruction to power for good better displayed than Hiroshima and Nagasaki in Japan which depend substantially on electricity from nuclear energy.
•Today, as the main nuclear arsenals are being dismantled and a comprehensive test ban treaty is in sight, commercial nuclear power provides 16 percent of the world's electricity. Several factors suggest that despite its sometimes controversial image, nuclear power has a much larger role to play in supplying the world's future energy needs.
The First two Atom Bombs in 1945
The Hiroshima bomb was made from highly-enriched uranium-235. This was prepared by diffusion enrichment techniques using the very small differences in mass of the two main isotopes: U-235 (originally
0.7% in the uranium) and U-238, the majority. As UF 6, there is about a one percent difference in mass between the molecules, and this enables concentration of the less common isotope. About 60 kilograms of highlyenriched uranium was used in the bomb which was released over Hiroshima, Japan's seventh largest city, on 6 August 1945. Some 90 % of the city was destroyed.
The explosive charge for the bomb detonated over Nagasaki three days later was provided by about of 8 kilograms of plutonium-239 (> 90 % Pu239), and its preparation depended on the operation of special nuclear reactors. During 1942, under conditions of wartime secrecy, the first human-designed reactor was constructed, in a squash court at the University of Chicago. It used highly purified graphite to slow the neutrons released in fission to enable further fission. This paved the way for more substantial production reactors at Hanford. The plutonium-239 generated in these could be separated by simple chemical methods, with no need for the complexities of isotope separation. The plutonium was first used for the test explosion at Alamogordo in New Mexico on 16 July 1945, ushering in the nuclear age with all its threat and promise.
The Effects of the Hiroshima and Nagasaki Bombs
The devastating effects of both kinds of bombs depended essentially upon the energy released at the moment of the explosion, causing immediate fires, destructive blast pressures, and extreme local radiation
189
exposures. Since the bombs were detonated at a height of some 600 metres above the ground, very little of the fission products were deposited on the ground beneath. Some deposition occurred however in areas near to each city, owing to local rainfall occurring soon after the explosions. This happened at positions a few kilometres to the east of Nagasaki, and in areas to the west and north-west of Hiroshima. For the most part, however, these fission products were carried high into the upper atmosphere by the heat generated in the explosion itself.
In Hiroshima, of a resident civilian population of 250 000 it was estimated that 45 000 died on the first day and a further 19 000 during the subsequent four months. In Nagasaki, out of a population of 174 000, 22 000 died on the first day and another 17 000 within four months. Unrecorded deaths of military personnel and foreign workers may have added considerably to these figures.
It is impossible to estimate the proportion of these 103 000 deaths, or of the further deaths in military personnel, which were due to radiation exposure rather than to the very high temperatures and blast pressures caused by the explosions. From the estimated radiation levels, however, it is apparent that radiation alone would not have been enough cause death in most of those exposed beyond a kilometer of the ground zero below the bombs. Most deaths appear to have been from the explosion rather than the radiation. Beyond 1.5 km the risk would have been much reduced (and 24 Australian prisoners of war about 1.5 km from the Nagasaki ground zero survived and many lived to a healthy old age.)
To the 103 000 deaths from the blast or acute radiation exposure have since been added those due to radiation induced cancers and leukaemia, which amounted to some 400 within 30 years, and which may ultimately reach about 550. (Some 93,000 exposed survivors are still being monitored.)
Teratogenic effects on foetuses was severe among those heavily exposed, resulting in birth deformities and stillbirths over the next 9 months. Beyond this, no genetic damage has been detected in survivors' children, despite careful and continuing investigation by a joint Japanese-US Foundation.
The major source of exposure in both cities was from the penetrating gamma radiations, and to a lesser extent from the neutrons (mostly at Hiroshima), emitted during and shortly after fission. There were two further, and smaller, sources of exposure. One, already mentioned, was due to the
190