
Воробева Нуцлеар Реацтор Тыпес (Леарн то реад бы реадинг) 2010
.pdfpower generation; 12) to account for the transient; 13) to refer to the HTGR; 14) to make use of ceramic coatings; 15) to take advantage of high efficiency.
Ex. 7. Give a free translation.
Атомная энергетика Англии развивается устойчивыми темпами, и в настоящее время её вклад в производство электроэнергии является существенным: в1980 годуонсоставил12,6 %.
Первая английская АЭС Calder Hall была запущена в 1956 году и имела суммарную мощность 200 МВт.
Особенностью английской атомной энергетики является то, что она основана на национальных разработках реакторов, а именно реакторов газоохлаждаемого типа. Английские специалисты утверждают, что их реакторы экономичны, надежны, безопасны. Опыт развития английской энергетики подтверждает эту характеристику. Первый реактор АЭС Calder Hall надежно работает уже более 25 лет.
Английские специалисты разработали по существу два типа га-
зоохлаждемых реакторов: GCR (Gas-Cooled Reactor), AGR (Advanced Gas-Cooled Reactor). В этих двух реакторах используется графит и как замедлитель, и как отражатель, а теплоносителем служит углекислый газ (CO2). GCR отличается от AGR тем, что загружается природным ураном, в то время как последний — ураном, обогащенным в незначительной степени.
READING 6-B
HTR
High Temperature Reactor
Thermal Reactor-Graphite Moderatе
Indicative data for а reactor of 1300 MW(e) size:
Uranium enrichment 10 % U235. Coolant outlet temperature 720 °С. Pressure 715 psi. Thermal efficiency 39 %. Core dimensions 9.8 m dia × × 6 m high
The inherent high temperature of this type of reactor makes it a potential source of process heat. Experimental versions have been built in the UK, USA and Germany. Its commercial future is however still uncertain.
51
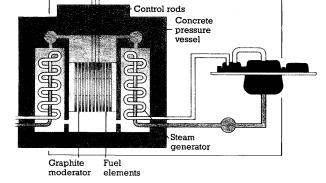
Fuel: Small spheres (about 800µm diameter) of uranium dioxide with a coating of silicon carbide, bonded h a graphite matrix and assembled with graphite into а fuel element. The fuel can operate at higher temperatures than metal clad fuel and а larger proportion is 'burned up'.
Moderator: Graphite, replaced with the fuel at each fuel change. Core layout: Fuel elements are arranged with vertical coolant pas-
sages in the graphite moderator.
Heat extraction: Helium gas is heated by passing over the fuel in the core and transfers its heat to water in а steam generator; the steam drives а turbine coupled to an electric generator. It is possible to use the heat directly in chemical processes.
The reactors discussed above depend on 235U for their initial fissile loading. The “enrichment”, the percentage of 235U, varies considerably. However, in each case the uranium is present in a ceramic form, often as uranium dioxide (UO2), but also - in the case of the HTGR - as a carbide. Use of uranium dioxide, rather than metallic uranium, reduces the density of uranium atoms, in the fuel and also reduces the heat conductivity of the fuel, both disadvantages from the point of view of reactors. However, ceramics such as uranium dioxide have the advantage over metallic uranium of greater stability against radiation damage and chemical corrosion; the dioxide also retains a large portion of the gaseous fission products which in other environments might more easily escape. Uranium dioxide’s high melting point (2800 °C) allows high-temperature operation, overcoming to some extent its low conductivity. The other nuclear materials with possible
52
importance in reactors are plutonium and thorium. For our “commercial” nuclear reactors, these too would be present as dioxides and carbides. However, it is important to note that advanced forms of these reactors could utilize nuclear fuels in other chemical forms.
READING 6-C
In the 1950’s and 1960’s, when the US developed LWRs, Britain and France developed gas-cooled graphite-moderated reactors, in which the coolant was carbon dioxide. The first series of gas-cooled reactors built in Britain were called Magnox reactors because the fuel rods, which contained natural unenriched uranium, were clad in a magnesium alloy.
Britain now has more than 40 gas-cooled reactors in operation or under construction, France has 7 and Italy, Spain and Japan have one each. More than 600 reactor-years of operating experience has been gained with the European gas-cooled reactors.
The US concept of the high-temperature gas-coded reactor (HTGR) evolved from those concepts of Britain and France, and was tested at Peach Bottom and on a larger scale at Fort St. Vrain. Because the US has plentiful supplies of helium, that gas was selected as a coolant instead of Carbon dioxide. Helium has the important advantage that it is stable to the high radiation flux in the reactor, does not become radioactive, is chemically inert and has excellent heat-transfer characteristics.
The attractive features of HTGR’s are as follows: they have efficiencies as good as the best fossil-fuel plants and are substantially more efficient than the water-cooled reactors. They not only get better thermal efficiency but also get better energy utilization out of each pound of uranium. HTGR’s have some safety advantages. Because the fuel elements are embedded in a massive matrix of graphite, which absorbs the heat, the t° within the reactor core rises only slowly, and there is time to take safety measures. And because the HTGR operates at an efficiency of about 39 percent compared with an efficiency of about 33 percent for LWR’s the HTGR releases about 25 percent less waste heat to be dissipated into the surrounding environment.
53
UNIT VII
BREEDING REACTORS
READING 7-A
The basic idea of a breeder is to effectively increase conversion enough so that the conversion ratio exceeds 1. To be more precise, the actual quantity of interest for breeders is the “breeding ratio”, the ratio of the number of fissile atoms produced to the number of the same kind that are consumed. This definition is more precise than that of conversion ratio (where we do not distinguish between fissile types) and is appropriate for breeders where basically the same material is bred as is supplied. Exceeding 1 implies production of enough fissile material both to replace that consumed in the reactor and to fuel additional reactors.
For an expanding nuclear system, an important number is the “doubling time”, the period required to double the amount of fissile that was initially loaded. It is the time after which a second reactor could be fueled from the excess productivity of the first. Assuming that the electrical energy produced is simply proportional to the total number of fissioned nuclei, it is easy to see how the doubling time is related to the breeding ratio. Defining the breeding gain as the excess of the breeding ratio over 1, the amount of excess fissile material produced per day for any given power level will be proportional to this gain assuming other factors — such as the electrical energy per fission and the ratio of absorption to fission cross sections — are fixed. The doubling time is simply the ratio of the reactor inventory to this amount of excess material, so that doubling time is proportional to inventory divided by breeding gain.
In practical terms, there are two classes of breeders: “thermal” breeders, which “tune up” the conversion ratio by using a thorium cycle to achieve breakeven or better and “fast” breeders, which take care not to moderate the neutrons, thereby taking advantage of the increased value of eta (the number of neutrons produced per neutron absorbed in the fuel) in a fast spectrum. The latter exceeds 2 by an amount which suggests that breeding is easily available. However, difficulties arise because the fission cross sections are much smaller than for thermal neutrons, so that a higher concentration of fissile is necessary to make use of the neutrons. The result is that the core of a fast breeder is typically very compact, with fissile enrichments of 15 % and greater; around
54
this “seed” region is a “blanket” of fertile fuel to utilize any neutrons that escape for fissile production. The liquid metal fast reactor (LMFBR) is now the mainstay of every breeder development program in existence, including that of the United States. The molten-salt breeder reactor is a thermal reactor, but its concept is radically different; because it is no longer being developed, we do not consider it here.
Exercises
Ex. 1. Answer the following questions:
1. What are the main disadvantages of thermal reactors? 2. What are the prospects for atomic power engineering for the rest of this century? How many reactors are expected to operate by 2000? What amount of U will they require? What proved resources of U are thought to be available? 3. What questions will arise after 2000? 4. What is the solution to this problem? 5. What reactor is referred to as a breeder? 6. What is the breeding ratio? What is the difference between the breeding ratio and the conversion ratio? What is the breeding gain? 7. What is the doubling time? In what way is the doubling time related to the inventory and to the breeding gain? 8. What are the two classes of breeders? 9. What is the distinctive feature of a thermal breeder? 10. What are the essential features of a fast breeder? 11. What is meant by the value of eta? 12. What is the disadvantage of fast breeders? 13. What types of fast breeders do you know? Which is the mainstay of the breeder development program?
Ex. 2. Give the 3 forms:
to burn, to build, to think, to begin, to breed, to feed, to grow, to do, to see, to give, to take, to arise, to leave.
Ex. 3. Translate. Pay attention to the underlined words.
1. The solution to this problem is that we should begin building breeders. 2. Fast breeders take care not to moderate neutrons, thereby taking advantage of the increased value of eta. 3. A higher concentration of fissile is necessary to make use of the neutrons. 4. Surrounding the seed region is a blanket of fertile fuel. 5. One such modification is to reduce the coolant-to-fuel ratio, thereby decreasing neutron absorption by water. 6. We can illustrate this question in terms of the multiplication
55
factor. 7. One can express the same information in terms of the decay constant. 8. An intermediate cooling loop is no longer necessary. 9. In the HTGR the coolant is helium rather than carbon dioxide. 10. It takes time to solve this complicated problem.
Ex. 4. Translate. Pay attention to the meanings of “one”.
1. One system operates while the other is shut down for decay. 2. 0ne can express the same information in terms of the decay constant. 3. One is to choose a moderator that absorbs fewer neutrons, say, heavy water instead of light water. 4. Of two nuclei with the same mass number, the one with the more protons and the fewer neutrons has the larger charge radius. 5. The ground state is the only one that is stable. 6. One measure of the performance of a breeder reactor is its breeding ratio. If the ratio is greater than one, the reactor will breed more nuclear fuel than it consumes. 7. A nuclear drop like one of water can take a variety of shapes. 8. One neutron, on the average, is needed to continue the chain reaction; one must convert a fertile nucleus to one that is fissile.
Ex. 5. Analyze the sentences
1. Even if we agreed not to build any new fission power plants after the year 2000, fuel requirements for those already built would substantially exceed the uranium now thought to be available.
Ex. 6. Translate. Pay attention to the Participle:
1) the plants operating; |
12) the atoms consumed; |
2) the plants starting up; |
13) the material bred; |
3) the reactors being operated; |
14) the material supplied; |
4) the reactors being built; |
15) the nuclear system expanding; |
5) the plants already built; |
16) the reactor being developed; |
6) the uranium required; |
17) the period required; |
7) the values estimated; |
18) the energy produced; |
8) uranium burned; |
19) the nuclei fissioned; |
9) the fuel used; |
20) the value increased; |
10) the fuel to be used; |
21) therely increasing the value. |
11) the atoms produced; |
|
56
Ex. 7. Translate. Define the functions of the Infinitive.
1. To carry out the program of such experiments it is necessary to have a source of energetic particles. 2. To carry out this program of experiments is necessary. 3. To be more precise, the actual quantity of interest for breeders is the breeding ratio. 4. The basic idea of a breeder is to increase conversion ratio. 5. The purpose of a nuclear power system is to make energy stored in the nucleus available as electricity. 6. The models to be applied in this calculation are carefully specified. 7. Many reactors require that uranium fuel be enriched to contain a higher percentage of U235. 8. There is much information to be obtained by raising the energy of the nucleus to an excited level. 9. The basic purpose of the containment system is to minimize the amount of radioactivity released. 10. Breeders not only replace their own fuel, but produce an excess to be used in other reactors.
Ex. 8. Condense the following sentences according to the model using the Infinitive Constructions instead of subordinate clauses.
Model: One can expect that the scope of research expands steadily. One can expect the scope of research to expand steadily.
1. One knows that physicists have penetrated the mysteries of the atomic nuclei. 2. You know that the fission of certain heavy elements give a large amount of heat. 3. We .may suppose that the alpha particles within the nucleus are in motion. 4. They expect that breeders will be necessary by the end of this century. 5. Specialists prove that an important number for an expanding nuclear system is the doubling time. 6. Specialists assume that the energy produced is proportional to the total number of fissioned nuclei.
7.One can easily see that the doubling time is related to the breeding ratio.
8.The latter number exceeds 2 by an amount which suggests that breeding is easily available. 9. We watch that the core of a fast breeder is typically compact. 10. We have shown that the LMFBR is now the mainstay of every breeder development program in existence.
Ex. 9. Give a free translation.
Реакторы на быстрых нейтронах — бридеры — имеют важные преимущества. Во-первых, быстрые нейтроны вызывают деление не только U235, но и U238. Во-вторых, ядра U238, которые поглощают
57
нейтрон, но не подвергаются делению, после двукратного бетараспада превращаются в ядра Pu239. Последний является высокоэффективным ядерным горючим.
Однако чтобы реализовать эти преимущества, необходимо решить ряд проблем. Во-первых, активная зона бридера должна быть очень компактной. Во-вторых, бридеры требуют особых конструкционных материалов. В-третьих, недопустимо использование в качестве теплоносителя воды, так как нейтроны замедляются чрезвычайно быстро, сталкиваясьс атомамиводорода.
Отсюда проблема теплоносителя. Однако эти сложные проблемы в значительной степени к настоящему времени решены. Одним из основных научно-исследовательских институтов по разработке быстрых реакторов в СССР является физико-энергетический ин-
ститут в г. Обнинске (The Physics Power Engineering Institute).
READING 7-B
Fast Reactors
Uranium resources are not unlimited. Although a gram of 235U “burned” in a reactor, yields more energy than a ton of coal, reasonably assured U.S. deposits of uranium are only enough to supply, until the year 2000, reactors as they are presently being built and operated. By that year there will be roughly 1000 nuclear power plants operating, and the cumulative uranium requirements for initial cores and for refueling will amount to 2 or 3 million tons of U3O8. This is comparable to the total amount of uranium that is presently thought to be available at reasonable economic and environmental cost. The question is, then after the year 2000, how do we supply (1) initial cores for new plants starting up and (2) the continuing fuel needs of the accumulated nuclear power system? Even if we agreed not to build any now fission power plants after that year, fuel requirements for those already built would substantially exceed the uranium now thought to be available. The standard solution to this problem is that late in this century we should begin building “breeder” reactors which not only replace their own fuel, but produce an excess to be used in other reactors.
58
Fast reactors currently use mixed plutonium-uranium fuel produced initially as a by-product from thermal reactors. The plutonium is sufficiently concentrated for the fission reaction to be sustained by fast neutrons so no moderator is necessary. Without a moderator, the core of the reactor is compact and has a high heat rating so very efficient coolantliquid sodium is used to transfer the heat.
In addition, the core can be surrounded by a uranium blanket and thus 'breed' more plutonium.
Doubling time
As we have already mentioned, the doubling time is simply the ratio of the reactor (or fuel cycle) inventory to the amount of excess material. Doubling time is therefore proportional to the fissile inventory divided by the breeding gain.
Strictly speaking, this definition would be correct only if the excess fissile material were held in storage until a sufficient amount accumulated to supply the entire fissile inventory of a new reactor. In the more likely situation, excess production of a number of reactors would contribute to a single new reactor, so that even the excess fissile material would soon be producing its own excess. In this situation, the rate of excess production has, effectively, to be compounded to yield a real doubling time.
In other respects, too we have not defined doubling time precisely enough. For example, we have not given any attention to how differing input and output isotopic mixes affect the definition of doubling time. However, for present purposes, the most important clarification is to distinguish the doubling time associated with only the fuel inventory of the reactor itself and that associated with the fuel, not only in the reactor, but also in the fuel cycle, i.e., in storage, reprocessing, fabrication, so on. The practical doubling time of a breeder reactor system is the time it takes the reactor and its facilities to yield enough excess fissile material to supply an additional reactor and its support facilities.
59
UNIT VIII
FAST REACTORS
READING 8-A
Liquid metal fast breeder reactor.
As indicated by the name, the LMFBR uses a liquid metallic coolant, a suitable choice where good heat-transfer characteristics are required. The basic nuclear configuration for all fast breeders is similar. The core is a compact arrangement of fuel assemblies that are similar to those used in light water reactors except that the fuel material is more highly enriched, the fuel pins typically have a smaller diameter, and the cladding is stainless steel rather than zircaloy.
Instead of using 235U or 233U as the fissile material, the present choice is to load the core with 239Pu; this is the inevitable product of the current generation of light water reactors, and because of its high value of eta (neutrons produced per neutron) in a fast spectrum, It is much more profitably used in the breeder than in thermal reactors. (235U, on the other hand probably does not yield enough neutrons for a practical breeder; this is not true of 233U, but the latter nuclide is not presently being produced in any large quantity). The fertile nuclide now chosen is 238U, at least in the LMFBR. This is expected to produce more than enough 239U to replace that which is consumed in the core. The “core” itself would be a mixture of oxides of plutonium and uranium. Surrounding this core would be a blanket of uranium. In both cases, the uranium would be almost entirely 238U. Fission would occur primarily in the core region and conversion would occur in both regions. Regular reprocessing would be needed to recover the bred fissile material.
The blanket assemblies would have rods with the same composition throughout. Core assemblies, on the other hand, would have fissile loads along the portion of the rods that constitutes the core, but only fertile material at the top and bottom of the rods. In this way, the entire core is surrounded with blanket regions.
Power generation in the core will be quite intense, compared with thermal reactors. As a result, the coolant must have good heat transfer properties. In the present case, the LMFBR, this has led to the choice of sodium as the coolant. A metal that is liquid over a large range of temperature, sodium can successfully cool the very compact core. Additional advantages of great importance are that it can be used at essentially atmospheric pressure thereby making design easier, and that it can
60