

Supplement A3: The Chemistry of Double-Bonded Functional Groups. Edited by Saul Patai Copyright 1997 John Wiley & Sons, Ltd.
ISBN: 0-471-95956-1
CHAPTER 11
Thermochemistry of olefins, carbonyl compounds and imines
SUZANNE W. SLAYDEN
Department of Chemistry, George Mason University, 4400 University Drive, Fairfax, Virginia 22030-4444, USA
Fax: 703-993-1055; e-mail: SSLAYDEN@GMU.EDU
and
JOEL F. LIEBMAN
Department of Chemistry and Biochemistry, University of Maryland Baltimore County, 1000 Hilltop Circle, Baltimore, Maryland 21250, USA
Fax: 410-455-2608; e-mail: JLIEBMAN@UMBC2.UMBC.EDU
I. INTRODUCTION: SCOPE AND DEFINITIONS . . . . . . . . . . . . . . . . |
538 |
A. Thermochemistry . . . . . . . . . . . . . . . . . . . . . . . . . . . . . . . . . . . |
538 |
B. Olefins, Carbonyl Compounds and Imines . . . . . . . . . . . . . . . . . . . |
539 |
II. ACYCLIC OLEFINS . . . . . . . . . . . . . . . . . . . . . . . . . . . . . . . . . . . |
540 |
A. Sources of Data . . . . . . . . . . . . . . . . . . . . . . . . . . . . . . . . . . . . |
540 |
B. Assessment of Alkene Data . . . . . . . . . . . . . . . . . . . . . . . . . . . . |
541 |
C. Enthalpies of Hydrogenation of Alkenes . . . . . . . . . . . . . . . . . . . . |
543 |
D. Constancy of the Enthalpy of Hydrogenation . . . . . . . . . . . . . . . . . |
550 |
E. Enthalpies of Isomerization of Alkenes . . . . . . . . . . . . . . . . . . . . . |
550 |
III. CYCLIC OLEFINS WITH A CHDCH GROUP . . . . . . . . . . . . . . . |
558 |
A. Cis-Cycloalkenes . . . . . . . . . . . . . . . . . . . . . . . . . . . . . . . . . . . |
558 |
B. Trans-Cycloalkenes . . . . . . . . . . . . . . . . . . . . . . . . . . . . . . . . . . |
559 |
C. Bicycloalkenes . . . . . . . . . . . . . . . . . . . . . . . . . . . . . . . . . . . . . |
560 |
D. Polycycloalkenes . . . . . . . . . . . . . . . . . . . . . . . . . . . . . . . . . . . |
562 |
IV. EXO- VS ENDO-CYCLIC DOUBLE BONDS . . . . . . . . . . . . . . . . . . |
563 |
A. Isomeric Methylcycloalkenes and Methylenecycloalkanes . . . . . . . . . |
563 |
B. Related Polycyclic Species . . . . . . . . . . . . . . . . . . . . . . . . . . . . . |
564 |
C. Isomeric Ethylcycloalkenes, Ethylidenecycloalkanes |
|
and Vinylcycloalkanes . . . . . . . . . . . . . . . . . . . . . . . . . . . . . . . . |
564 |
537

538 |
Suzanne W. Slayden and Joel F. Liebman |
|
V. BREDT’S RULE, BRIDGEHEAD OLEFINS AND CYCLIC SPECIES |
|
|
|
WITH A >CDCH OLEFINIC LINKAGE . . . . . . . . . . . . . . . . . . . . |
565 |
VI. CYCLIC OLEFINS CONTAINING A TETRASUBSTITUTED |
|
|
|
DOUBLE BOND . . . . . . . . . . . . . . . . . . . . . . . . . . . . . . . . . . . . . |
566 |
|
A. Monocyclic 1,2-Disubstituted Cycloalkenes . . . . . . . . . . . . . . . . . . |
566 |
|
B. Bicyclo[m.n.0]alkenes and Related Species . . . . . . . . . . . . . . . . . . |
568 |
|
C. Polyhedrenes . . . . . . . . . . . . . . . . . . . . . . . . . . . . . . . . . . . . . . |
569 |
VII. OLEFINS WITH AROMATIC SUBSTITUENTS . . . . . . . . . . . . . . . . |
571 |
|
|
A. Phenylated Ethylenes . . . . . . . . . . . . . . . . . . . . . . . . . . . . . . . . . |
571 |
|
B. ‘Tolylated’ Ethylenes . . . . . . . . . . . . . . . . . . . . . . . . . . . . . . . . . |
572 |
|
C. Alkylated Styrenes . . . . . . . . . . . . . . . . . . . . . . . . . . . . . . . . . . |
573 |
|
D. ‘Cyclic Styrenes’ . . . . . . . . . . . . . . . . . . . . . . . . . . . . . . . . . . . . |
574 |
VIII. ACYCLIC ALDEHYDES AND KETONES . . . . . . . . . . . . . . . . . . . . |
575 |
|
|
A. Assessment and Correlation of Data . . . . . . . . . . . . . . . . . . . . . . . |
575 |
|
B. Enthalpies of Isomerization . . . . . . . . . . . . . . . . . . . . . . . . . . . . |
576 |
|
C. Enthalpies of Reaction . . . . . . . . . . . . . . . . . . . . . . . . . . . . . . . . |
578 |
IX. ALICYCLIC KETONES . . . . . . . . . . . . . . . . . . . . . . . . . . . . . . . . . |
581 |
|
|
A. Monocyclic Ketones . . . . . . . . . . . . . . . . . . . . . . . . . . . . . . . . . |
581 |
|
B. Bicyclic and Polycyclic Ketones . . . . . . . . . . . . . . . . . . . . . . . . . |
582 |
|
C. Comparison of Alicyclic Ketones and Their Methylene Analogs . . . . |
584 |
|
D. Methylated Cyclic Ketones . . . . . . . . . . . . . . . . . . . . . . . . . . . . . |
585 |
|
E. Solid Cyclic Ketones . . . . . . . . . . . . . . . . . . . . . . . . . . . . . . . . . |
586 |
|
F. Adamantyl Ketones . . . . . . . . . . . . . . . . . . . . . . . . . . . . . . . . . . |
587 |
X. CARBONYL COMPOUNDS WITH ARYL SUBSTITUENTS . . . . . . . . |
588 |
|
|
A. The Choice of Phase . . . . . . . . . . . . . . . . . . . . . . . . . . . . . . . . . |
588 |
|
B. Alkyl Phenyl Ketones . . . . . . . . . . . . . . . . . . . . . . . . . . . . . . . . |
588 |
|
C. Alkyl Acetophenones . . . . . . . . . . . . . . . . . . . . . . . . . . . . . . . . |
590 |
|
D. Alkyl Benzophenones . . . . . . . . . . . . . . . . . . . . . . . . . . . . . . . . |
590 |
XI. POLYCARBONYL COMPOUNDS . . . . . . . . . . . . . . . . . . . . . . . . . |
591 |
|
XII. IMINES . . . . . . . . . . . . . . . . . . . . . . . . . . . . . . . . . . . . . . . . . . . |
593 |
|
|
A. Definition and Organization . . . . . . . . . . . . . . . . . . . . . . . . . . . . |
593 |
|
B. Methylenimine . . . . . . . . . . . . . . . . . . . . . . . . . . . . . . . . . . . . . |
594 |
|
C. Methylated Methylenimines . . . . . . . . . . . . . . . . . . . . . . . . . . . . |
594 |
|
D. Unsaturated Imines: Propenaldimine . . . . . . . . . . . . . . . . . . . . . . . |
595 |
|
E. Cyclic Imines: 1-Azacyclopentene . . . . . . . . . . . . . . . . . . . . . . . . |
595 |
|
F. ‘Simple’ Acyclic Aldimines . . . . . . . . . . . . . . . . . . . . . . . . . . . . |
596 |
|
G. Aliphatic and Alicyclic Ketimines . . . . . . . . . . . . . . . . . . . . . . . . |
596 |
|
H. Aromatic Aldimines . . . . . . . . . . . . . . . . . . . . . . . . . . . . . . . . . |
598 |
|
I. Polyimines . . . . . . . . . . . . . . . . . . . . . . . . . . . . . . . . . . . . . . . |
598 |
|
J. Unusual Tautomers . . . . . . . . . . . . . . . . . . . . . . . . . . . . . . . . . . |
599 |
XIII. REFERENCES AND COMMENTARY . . . . . . . . . . . . . . . . . . . . . . . |
601 |
I.INTRODUCTION: SCOPE AND DEFINITIONS
A.Thermochemistry
As has been the approach for most of the authors’ other reviews on organic thermochemistry1 8, the current chapter will be primarily devoted to the relatively restricted property, ‘standard enthalpy of formation’ (more commonly and colloquially called heat of formation), where we will write this quantity as H°f , instead of the increasingly more commonly used and also proper fH°m. By intent, this chapter will forego discussion of other thermochemical properties such as Gibbs energy, entropy, heat
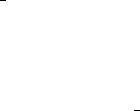
11. Thermochemistry of olefins, carbonyl compounds and imines |
539 |
capacity and excess enthalpy. Additionally (following thermochemical convention9), the temperature and pressure will be tacitly assumed to be 25 °C (‘298 K’) and 1 atmosphere (taken as either 101,325 or 100,000 Pa), respectively, and the energy units will be kJ mol 1 (where 4.184 kJ 1 kcal).
Again following our earlier chapters as precedent, we will continue to view intermolecular forces as ‘complications’ and ‘nuisances’ to be avoided whenever possible. As such, unless explicitly noted to the contrary, any species to be discussed in this chapter will be assumed in the (ideal) gas phase. To interrelate these data with those for the liquid or solid state that characterizes most organic compounds as synthesized, reacted, purified and thermochemically investigated, it will be necessary to make ‘corrections’ to the gaseous state by using enthalpies of vaporization and of sublimation. These are defined
by equations 1 and 2 |
|
HV D Hf° g Hf° l |
1 |
and |
|
Hs D Hf° g Hf° s |
2 |
where g, l and s refer to gas, liquid and solid, respectively. As always has been our practice, phase change enthalpies will be taken from whatever source available: our choice to maximize the use of gas-phase data and minimize that from the liquid or solid requires numerous expediencies. In the absence of data from experimental measurements, enthalpies of vaporization for hydrocarbons will usually be estimated using the generally accurate (š2 kJ mol 1) two-parameter equation of reference 10. It is only rarely that enthalpies of sublimation will be needed.
Finally, we note that it will occasionally be necessary to use data for a species as liquid when the compound is ‘normally’ a solid, or as a solid when it is ‘normally’ a liquid. These two phases are interrelated by the enthalpy of fusion, equation 3.
Hfus D Hf° l Hf° s |
3 |
We admit now that all phase change enthalpies should refer to determinations at 298 K. Again, for the sake of simplicity, the generally small corrections to these enthalpies to transform the temperature-dependent data to 298 K will not be made in the current study.
B. Olefins, Carbonyl Compounds and Imines
For this chapter we define an ‘olefin’ as any organic compound that contains but one carbon carbon double bond and limit substitution to only ‘hydrocarbyl’ groups (i.e. composed of hydrogen and carbon). After all, the thermochemistry of some heterosubstituted olefins has already been the subject of specialized chapters in earlier ‘Patai’ volumes. Enones2, enols4,11 and enamines5, and vinyl sulphides, sulphoxides and sulphones3 have figured prominently in other ‘not-so-narrow’ Patai volumes. Relatedly, olefins with additional unsaturation in the form of one or more double bonds8 and even cyclopropane rings7 will likewise be largely ignored in this chapter.
‘Carbonyl compounds’ will be taken in this chapter to mean any organic compound that contains at least one carbon oxygen double bond where we limit the substitution to only saturated aliphatic, saturated alicyclic and aryl ‘hydrocarbyl’ groups. Carbonyl compounds with a variety of unsaturated substituents have earlier been discussed within the context of enones4. Non-hydrocarbyl substituents, ‘X’, may be directly attached to the carbonyl and elsewhere in the molecule. The first type of species, RCOX, is alternatively identified as acyl derivatives such as carboxylic acids and their esters, halides and amides and have already been discussed in a recent Patai thermochemistry
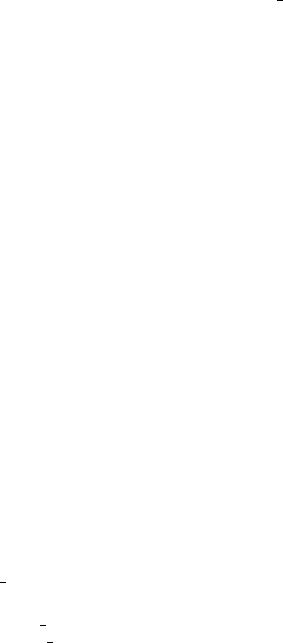
540 |
Suzanne W. Slayden and Joel F. Liebman |
chapter12. Some members of the second type have likewise been discussed as derivatives of the group ‘X’ (e.g. for a brief thermochemical discussion of ˛-haloketones, see Reference 6).
By the word ‘imine’ we mean a species containing at least one carbon nitrogen double bond that is substituted only by hydrocarbyl groups. Therefore, we are omitting such C- heterosubstituted species as amidines and imidates13, isocyanates and isothiocyanates14, as well as N-heterosubstituted species such as diazo compounds, hydrazones, nitronates, nitrones and oximes. We are also ignoring compounds with either meaning of the term azine, both that of > CDN NDC < as found in benzalazine, PhCHDN NDCHPh, and 6-membered ‘benzenoid’ rings containing nitrogen such as pyridazine and 1,3,5-triazine as well as pyridine and pyrimidine. After all, the first type of azine violates the hydrocarbyl group limitation. Inclusion of the second would have suggested benzene and its derivatives are to be included in discussion of trienes in Reference 8. To do so appears somewhere between nuisance and nonsense.
The choices of olefin, carbonyl compounds and imines have been made to allow for simple access to relevant data. All of these compounds contain carbon, hydrogen and possibly oxygen or nitrogen and so combustion calorimetry is relatively facile and direct15. As will be shown in later sections, hydrogenation and other types of reaction calorimetry can be of considerable use. We have already acknowledged that we will use relevant estimation approaches to obtain enthalpies of vaporization and sublimation to maximize the usefulness of the data available. A major simplification arises from our long-term bibliographic preferences and prejudices. We have long chosen to cite secondary and generally archival sources for enthalpies of formation16,17 and phase changes18 instead of primary sources. We find that this policy greatly simplifies the writing and reading of our text even as we admit the risk of offending an occasional author of a therefore uncited primary research paper.
II. ACYCLIC OLEFINS
A. Sources of Data
In a previous volume of this series19, the author of the chapter on the thermochemistry of double-bonded compounds reported that in the period following the publication of comprehensive reviews and monographs a few years earlier17b,20 only a small amount of experimental work had been performed. Consequently, primary attention in that chapter focused on developments in estimating enthalpies of formation by electrostatic models and group additivity. Much has been accomplished in the ensuing 20 years. Pedley and coworkers16 have published three editions of their compendium of thermochemical data, giving easy access to much of the existing experimental enthalpy-of-formation and enthalpy-of-reaction data of interest in this chapter. Steele and Chirico21 reviewed the thermodynamic properties of the acyclic mono-olefins beginning with C5. The enthalpies of formation for the liquid, gas and ideal-gas state at 298.15 K were calculated for 47 compounds based on experimental enthalpy-of-combustion values. Where these authors believe there are errors in the original experimental results they suggest revised values. Jensen22 tabulated the results of a Chemical Abstracts literature search through 1974 for experimental enthalpies of hydrogenation which yielded data on 225 compounds. Rogers and coworkers23 29 have devised a method for the microcalorimetric determination of the enthalpies of hydrogenation of alkenes in dilute alkane solution, the results of which they maintain23 are equivalent to gas-phase hydrogenation values. Contemporaneously, Wiberg and coworkers30 32 showed that it is possible to determine the overall enthalpy of hydration of many C4 C8 alkenes in the liquid phase by measuring the enthalpies
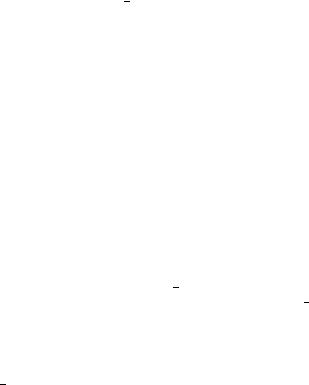
11. Thermochemistry of olefins, carbonyl compounds and imines |
541 |
of reaction of the alkene and alcohol with trifluoroacetic acid containing trifluoroacetic anhydride. In addition to determining enthalpies of formation of the alcohols, the authors suggest revisions to several of the enthalpies of formation of the alkenes based on their experimental enthalpies of isomerization.
B. Assessment of Alkene Data
Although there are abundant data for enthalpies of reaction of alkenes (combustion, hydrogenation, hydration, isomerization etc.) in the literature, some authors have expressed concern about the accuracy of the results. Jensen emphasizes in his review22 of enthalpies of hydrogenation the requirement for careful measurement of reaction enthalpies for reliably assessing isomerization, strain and stabilization energies. In this compilation of structurally diverse compounds, he provides information regarding precision of measurement and special phase or solvent conditions. Additionally, he calculates some interesting and useful enthalpies of hydrogenation using the most precise data. The focus of recent discussion is whether the precision of the enthalpy of reaction measurements reflects the accuracy of the measurements. Various authors23 32 state that the precision of reaction calorimetry reflects a comparable improvement in accuracy. Thus, because the enthalpy- of-hydration and -hydrogenation calorimetric measurements are inherently more precise than enthalpy-of-combustion measurements, they yield more accurate information regarding alkene energetics when used directly. Such considerations as optimum molecular weight in combustion calorimetry or undetermined, and perhaps not-quite-negligible, solvent effects in reaction calorimetry were cited as possibly affecting the accuracy of the various methods. Many of the enthalpies of formation listed by Pedley and colleagues16 are composite values incorporating two or more separate measurements33.
Enough data for the 1-n-alkenes exist to illustrate the magnitude of any differences we might expect to encounter were we to choose one set of enthalpy-of-formation data or another. Our method is first to plot the available experimentally derived enthalpies of formation for each member of the homologous series versus the total number of carbons, nc, in the compound. Such a procedure is known to produce a reasonably straight line34 as expressed in equation 4. A linear regression analysis using the method of weighted least squares reveals the goodness of fit and the associated uncertainty in the slope, ˛, and the y-intercept, ˇ. Results are shown in Table 1.
Hf g D ˛gžnc C ˇg |
(4a) |
Hf l D ˛lžnc C ˇl |
(4b) |
The data from pedley and colleagues16 includes nc D 4 8, 10, 12 and 16 while that from Steele and Chirico21, derived only from combustion enthalpies, comprises nc D 5 8, 10, 12 and 16. The enthalpies of formation for C8H16(l), C10H20(l,g), and C12H24(l,g) used in these two regression analyses are from the same primary sources and are thus identical. Because there is ca 4 kJ mol 1 range in the enthalpies of formation for 1-hexene reported in these two references (the reason for which will be explained below), Table 1 also includes regression analyses omitting the C6H12 data. Enthalpy-of-hydrogenation data24,26,27,29 for nc D 5 12, 16, 18 and the exceptionally consistent gaseous enthalpies of formation for alkanes from Pedley and colleagues16, were used to calculate enthalpies of formation35 according to equation 5.
Hf alkene, g D Hf alkane, g Hhyd |
5 |
Because of the precision of their alkene enthalpy of hydration measurements from Wiberg and coworkers30,31, the authors refined the liquid enthalpies of formation for 1-pentene,

542 |
|
Suzanne W. Slayden and Joel F. Liebman |
|||
|
TABLE 1. Results from the linear regression analysis of equation 4 for |
||||
|
1-n-alkenes (kJ mol 1) |
|
|
||
|
Reference |
|
Phase |
˛ |
ˇ |
|
|
|
|
|
|
|
16b |
|
l |
25.59 š 0.12 |
81.39 š 0.91 |
16 |
|
l |
25.61 š 0.10 |
81.65 š 0.761 |
|
|
21b |
|
l |
25.68 š 0.16 |
82.03 š 1.26 |
21 |
|
l |
25.65 š 0.15 |
81.56 š 1.17 |
|
30, 31 |
|
l |
25.38 š 0.006 |
79.96 š 0.03 |
|
|
16b |
|
g |
20.60 š 0.13 |
82.07 š 0.93 |
16 |
|
g |
20.62 š 0.10 |
82.37 š 0.73 |
|
|
21b |
|
g |
20.67 š 0.16 |
82.54 š 1.25 |
21 |
|
g |
20.63 š 0.13 |
81.96 š 1.11 |
|
24, 26, 27, 29 |
g |
21.52 š 0.15 |
82.32 š 1.35 |
a In the least-squares analyses of equation 4, the individual enthalpies were weighted inversely as the squares of the experimental uncertainty intervals. The correlation coefficients are all greater than 0.999.
b Analysis of equation 4 omitting the data for 1-hexene.
1-heptene and 1-octene by combining their data and the literature combustion data in a least-squares treatment. They accepted the literature enthalpy-of-hydrogenation value for 1-hexene36 and reduced the uncertainty by an arbitrary amount.
The enthalpy of formation for 1-hexene varies by ca 4 kJ mol 1 in the literature sources reviewed for this section. The enthalpy of combustion for liquid 1-hexene37 as cited by
Steele and Chirico21 is 4005.72 š 1.30 kJ mol 1 and the derived enthalpy of formation (T D 298.15 K, p° D 101.325 kPa, Hf(g, CO2) D 393.51 š 0.13 kJ mol 1,
Hf(l, H2O) D 285.830 š 0.042 kJ mol 1) is 70.32 š 1.46 kJ mol 1. The enthalpies of combustion of seventeen isomeric hexenes were later published only as values relative to 1-hexene38 where the authors remark ‘. . . one does not need an accurate value of the heat of combustion of 1-hexene for the present calculations’. This is probably a repudiation of the earlier measurement because in order to calculate enthalpies of formation or combustion for the isomeric hexenes they recommend using a standard enthalpy of formation for 1-hexene (l) of 17.30 kcal mol 1 ( 72.38 kJ mol 1) based on a derived linear equation correlating the experimental enthalpies of formation of 1-n-alkenes with the number of included methylene groups39. Some authors 20,31 selected an enthalpy of formation (l, 17.29 š 0.29 kcal mol 1 D 72.34 š 1.21 kJ mol 1) from the weighted mean of two enthalpies of hydrogenation36 although the latter citation indicates the enthalpy of formation is derived from combustion calorimetry. The selected enthalpies of formation for the isomeric hexenes in references 20 and 31 were all calculated from this value and the enthalpy-of-combustion ratios from Bartolo and Rossini38. Therefore, depending on which enthalpy of formation is chosen for 1-hexene, the calculated enthalpies of formation for the other sixteen hexene isomers differ systematically by ca 2 kJ mol 1. Finally, Pedley and colleagues16 incorporate not only the two enthalpies of hydrogenation from Reference 36, but also four enthalpies of isomerization from Reference 31 into a weighted-mean enthalpy of formation of 74.2 š 1.6 kJ mol 1.
The effect of excluding the enthalpy of formation of 1-hexene as determined either solely or in part from the combustion measurement21,37 in the regression analyses improves the goodness of fit. Using these regression constants to calculate liquid enthalpies of formation for 1-hexene yields 72.01 kJ mol 1 from the 1-n-alkene data in Reference 16 and 72.34 kJ mol 1 from similar data in Reference 21.
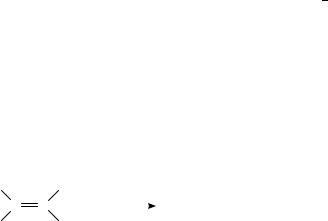
11. Thermochemistry of olefins, carbonyl compounds and imines |
543 |
Further review of the aforementioned references leads us to caution the reader not to accept uncritically the alkene enthalpy values cited throughout the literature. For example, the enthalpy of combustion for liquid cis-2-hexene38 has been repeatedly cited as probably incorrect21,26,31. Pedley and colleagues16 use that combustion enthalpy to determine a recommended enthalpy of formation which thus may be too negative by about 4 kJ mol 1. Wiberg and Wasserman31 also believe the enthalpy of combustion of liquid trans-3- hexene38 to be erroneous. Another questionable combustion enthalpy is for either one or both of the 3-methyl-3-hexenes40. The results show the cis (Z) isomer to be more stable by 2.6 kJ mol 1, contrary to the relative stabilities of most other isomeric cis trans pairs. According to others27, the trans (E) isomer probably is incorrect. In the original publication, a typographical error in the enthalpy of combustion41 for the liquid cis-1- phenyl-3,3-dimethylbut-1-ene was transformed into an incorrect enthalpy of formation in Reference 16 so that the actual stabilities of the cis and trans isomers appear reversed. In subsequent sections of this chapter we will continue to explore and comment on the quality of the data.
C. Enthalpies of Hydrogenation of Alkenes
A historically useful probe of alkene energetics is the exothermic enthalpy of hydrogenation ( Hhyd) accompanying the alkene reaction to the corresponding alkane (equation 6).
C C |
+ H2 |
|
|
|
|
|
|
|
|
|
|
(6) |
|
H |
|
C |
|
C |
|
H |
|||||
|
|
|
|
|||||||||
|
|
|
|
|
|
|
|
|
|
|
|
|
A simplifying assumption is that all members of a particular category of alkene, e.g. the 1-n-alkenes, will have about the same enthalpy of hydrogenation and, after enthalpies for a few representative compounds have been precisely measured, the mean value is applicable to other alkenes of the same structural type. This assumption is only true, or approximately so, under well-defined circumstances; if applied indiscriminately it can lead to errors in interpreting the energetics of alkenes. The basis for the assumption is clear after recasting equation 5 in the linear form of equation 7.
Hf alkane, g D mž Hf alkene, g C ‘ Hhyd’ |
7 |
Because the enthalpies of formation of homologous series of both alkanes and alkenes are linearly related to the total number of carbon atoms in their structures, the enthalpies of formation of each series are linearly related to each other. However, only in the case
where the slope, ˛alkane, from equation 4a for the alkanes is identical to the slope, ˛alkene, from equation 4a for the alkenes (and thus m D 1 in equation 7) is the enthalpy of
hydrogenation constant and equal to the y-intercept (‘ Hhyd’) of the equation 7 linear
regression42 (and thus to the difference in intercepts ˇalkane and ˇalkene from equation 4a). If the two slopes are significantly different, i.e. as the lines generated from equation 4a
become noticeably non-parallel, the enthalpy of hydrogenation will not be constant for the members of the alkene series but instead will exhibit a regular increase or decrease. Thus, the indicator of consistency in measurement of enthalpies of hydrogenation for a particular class of alkene is not necessarily a close correspondence in the measured enthalpies of hydrogenation; the values may naturally be different.
In the remainder of this section we identify members of several homologous series of alkenes and apply equation 4 to their enthalpies of formation. In this way we can assess
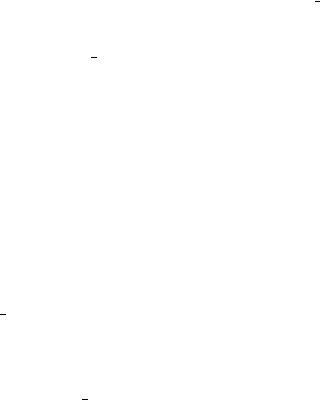
544 |
Suzanne W. Slayden and Joel F. Liebman |
the quality of the data as well as generate ˛alkene to compare with the corresponding ˛alkane. The calculation of the slopes, m, from equation 7 will show us in which series we might expect to find nearly constant enthalpies of hydrogenation. The difference between
the y-intercept (‘ Hhyd’) and the mean experimental Hhyd is a measure of the constancy of the enthalpy of hydrogenation for the series. When References 21 and 16 cite the same
enthalpy, we will cite Reference 16 as the source. Enthalpies of hydrogenation24 29 are used to calculate gaseous enthalpies of formation35 according to equation 5. Because so much data is from this source and because of our previous stated preference for this phase, we will generally restrict our interest to the gaseous phase. Gaseous enthalpies of formation cited from Wiberg and coworkers30 32 were calculated from the liquid enthalpies of formation given there and the enthalpies of vapourization43 from Reference 16. We accept as accurate the enthalpies of formation of the alkanes from Pedley.
For several of the compounds considered in this section there are two or three reported enthalpies of formation, most of which are identical within experimental error. Using a weighted mean and weighted uncertainty for these measurements, or not, can significantly affect the regression analysis. The weighted uncertainty is smaller than the uncertainty of the individual measurements and may be smaller than any of the experimental uncertainties for the other members of homologous series. When used in the weighted linear regression of equation 4, the measurement with the smaller uncertainty assumes greater influence on the fit of the resulting line. Their inclusion may be justifiable if the multiple enthalpies of formation were determined using the same experimental method or if the data for all members were treated in the same way. Neither of these being the case, we calculated a simple mean and uncertainty35 to use in the analyses.
The results of the regression analyses of equations 4a and 7 for the alkenes and the corresponding alkanes are reported in Table 2 and discussed below. The mean Hhyd experimental enthalpies of hydrogenation in Table 2 are determined from the same data chosen for the regression analyses and were obtained either from the enthalpies of formation of the corresponding alkenes and alkanes (equation 5) or from the measured enthalpies of hydrogenation24 29.
1. n-Alkenes
The most precise, consistent and presumably accurate enthalpies of formation in Table 1 are those for the liquid 1-n-alkenes from References 30 and 31. Converting to gaseous enthalpies of formation for nc D 5 7 and choosing gaseous enthalpies of formation for nc D 4, 8, 10, 12, 16 from Reference 16 and nc D 9, 11 from Reference 24 (the only source for these two members) gives us a set of enthalpies of formation which are co-linear according to equation 4a. There are three available enthalpies of formation for trans-2- hexene, two of which are identical within experimental error. However, none of them is co-linear with the other members of the homologous series; instead, the enthalpies lie on either side of the series line. By averaging all three available values, the resulting enthalpy of formation was incorporated into the straight line. For the trans-2-alkenes, the selected
values are: (C4H8)16; C5H10 D 31.5 š 1.5 16,21,30; C6H12 D 52.2 š 2.0 16,26,31;C7H14 D 73.3 š 1.8 21,27,30; and C8H16 29. For the cis-2-alkenes, the chosen values are: (C4H8)16; C5H10 D 26.9 š 1.4 16,21,30; C6H12 D 47.8 š 1.4 26,31,43;C7H14 D 69.3 š 1.3 21,30; and (C8H16)29. A difficult choice must be made from
the trans-3-alkene data. There are no three data points which describe a straight line with nc, not even those three which come from the same source26,27,29. We accept the only enthalpy of formation for trans-3-octene29. Because Reference 31 determined the enthalpy of formation of trans-3-hexene from isomerization of 1-hexene, an enthalpy of formation we trust, we accept that value and the one30 for C7H14. A plot of equation 4a
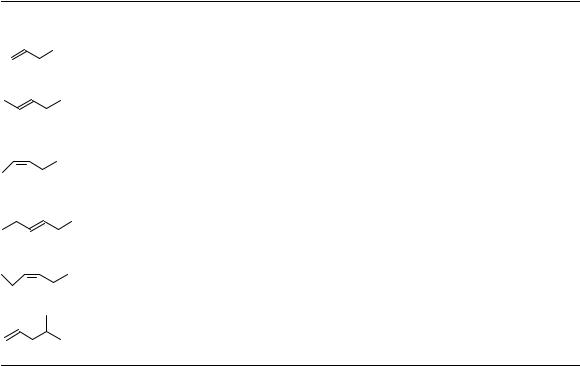
TABLE 2. Regression analyses of equations 4a and 7 for homologous series of alkenes and corresponding alkanes (kJ mol 1)a
|
Alkene Series |
|
Constants from equation 4 |
|
Constants from equation 7 |
Experimental |
||||||
|
|
|
|
|
|
|
|
|
|
|
|
Hhyd (g)f |
|
|
|
|
|
|
|
˛(g) |
ˇ(g) |
|
m(g) |
‘ Hhyd’(g)b |
|
|
c |
|
|
|
|
|
|
|
|
|
|
|
|
x D 1 |
|
( |
)x |
|
20.46 š 0.11 |
81.20 š 0.72 |
|
1.001 š 0.004 |
125.9 š 0.5 |
125.9 š 0.8 |
|
|
|
|
9, 13 |
|
|
|||||||
|
|
|
|
|
||||||||
c |
|
|
|
|
|
|
|
|
|
|
||
|
x D 1 |
|
( |
)x |
|
20.58 š 0.17 |
71.30 š 1.11 |
|
0.999 š 0.013 |
115.1 š 0.9 |
114.9 š 0.6 |
|
|
|
|
4 |
|
|
|
||||||
|
|
|
|
|
|
|||||||
|
x D 0 |
|
|
4 |
|
|
20.49 š 0.09 |
70.64 š 0.53 |
|
1.008 š 0.009 |
114.5 š 0.6 |
115.1 š 0.5 |
|
|
|
|
|
|
|||||||
c |
|
|
|
|
|
|
|
|
|
|
||
|
x D 1 |
|
( |
)x |
|
20.92 š 0.24 |
77.59 š 1.55 |
|
0.987 š 0.015 |
112.0 š 0.9 |
119.3 š 0.7 |
|
|
|
|
4 |
|
|
|
||||||
|
x D 0 |
|
|
4 |
|
|
20.64 š 0.17 |
75.70 š 0.97 |
|
1.001 š 0.012 |
119.1 š 0.7 |
119.1 š 0.7 |
|
|
|
|
|
|
|||||||
c |
|
|
|
|
|
|
|
|
|
|
||
|
|
|
|
|
( |
)x |
|
|
|
|
|
|
|
x D 1 |
|
|
3 |
|
|
21.50 š 0.69 |
78.24 š 4.69 |
|
0.980 š 0.030 |
117.2 š 2.2 |
115.8 š 0.8 |
|
|
|
|
|
|
|||||||
c |
|
|
|
|
|
|
|
|
|
|
||
|
|
|
|
|
( )x |
|
|
|
|
|
||
|
x D 1 |
|
|
3 |
|
|
22.09 š 0.06 |
85.93 š 0.40 |
|
0.939 š 0.006 |
123.3 š 0.5 |
119.1 š 1.4 |
|
|
|
|
|
|
|||||||
|
d |
|
|
|
|
|
|
|
|
|
|
|
( |
|
)x |
|
20.48 š 0.02 |
74.77 š 0.10 |
|
1.000 š 0.009 |
126.2 š 0.5 |
126.4 š 0.3 |
|||
|
x D 0, 1, 3 |
|
|
(continued overleaf )
545
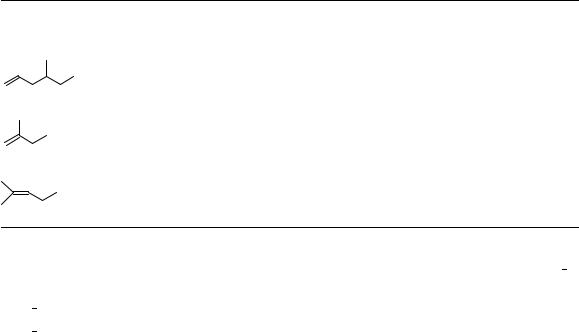
546
TABLE 2. (continued)
Alkene Series |
Constants from equation 4 |
|
Constants from equation 7 |
Experimental |
||
|
|
|
|
|
|
Hhyd (g)f |
|
˛ (g) |
ˇ (g) |
|
m (g) |
‘ Hhyd’(g)b |
|
e |
|
|
|
|
|
|
( |
|
)x |
|
|
|
|
|
|
||
x D 0 |
|
2 |
|
|
19.20 š 0.05 |
65.731 š 0.34 |
1.052 |
š 0.027 |
119.7 š 1.9 |
123.3 š 1.1 |
|
|
|
||||||||
d |
|
|
|
|
|
|
|
|
|
|
( |
|
)x |
|
|
|
|
|
|
||
x D 0, 2 |
|
4 |
20.74 š 0.11 |
66.10 š 0.63 |
0.974 |
š 0.001 |
117.8 š 0.1 |
116.1 š 0.9 |
||
|
||||||||||
d |
|
|
|
|
|
|
|
|
|
|
x D 0 |
|
( )x |
22.29 š 0.26 |
70.34 š 1.66 |
|
š .026 |
115.9 š 1.7 |
110.9 š 1.9 |
||
|
2 |
|
|
0.921 |
||||||
|
|
|
a In the least-squares analyses of equations 4a and 7, the individual enthalpies were weighted inversely as the squares of the experimental uncertainty intervals. The correlation coefficients are all greater than 0.999.
b From equation 7, the y-intercept in the regression analysis is interpreted as the estimated enthalpy of hydrogenation.
c The hydrogenation product is the corresponding n-alkane. The constants generated from equation 4a for the complete data set of n-alkanes (nc D 4 12, 16, 18) are: ˛ g D 20.63 š 0.06, ˇ g D 43.20 š 0.46 and r2 D 0.99994.
dThe hydrogenation product is the corresponding 2-methylalkane. The constants generated from equation 4a for the complete data set of 2-methylalkanes (nc D 4 8, 10) are: ˛ g D 20.50 š 0.23, ˇ g D 51.77 š 1.33 and r2 D 0.9995.
eThe hydrogenation product is the corresponding 3-methylalkane. The constants generated from equation 4a for the complete data set of 3-methylalkanes (nc D 6 8) are: ˛ g D 20.16 š 0.39, ˇ g D 51.02 š 2.70 and r2 D 0.99992.
fThe uncertainty interval was calculated as the square root of the sum of the squares of the individual enthalpies of hydrogenation.