

11. Thermochemistry of olefins, carbonyl compounds and imines |
577 |
|||||||||
TABLE 5. |
Enthalpies of isomerization of aldehydes and ketones (kJ mol 1) |
|
|
|||||||
R1 X R2 |
|
R3 X R4 |
Hisom(l) |
Hisom(g) |
||||||
R1 |
R2 |
|
R3 |
|
R4 |
|
|
|
|
|
n-Pr |
H |
|
i-Pr |
H |
8.2 š 2.1 |
11.0 š 1.7 |
||||
Me |
n-Pr |
|
Me |
i-Pr |
2.1 š 1.3 |
3.5 š 1.3 |
||||
Et |
n-Pr |
|
Et |
i-Pr |
5.7 š 1.3a,b |
7.8 š 1.3a,b |
||||
n-Pr |
n-Pr |
|
i-Pr |
i-Pr |
2.9 š 1.3 |
5.0 š 1.3 |
||||
Me |
n-Bu |
|
Me |
t |
-Bu |
. |
. |
. |
.5 |
|
n-Bu |
|
t-Bu |
|
6 6 |
š 1 3b |
10 9 š 1 b |
||||
n-Bu |
|
t-Bu |
7.1 š 1.8 |
0.5 š 1.8 |
a Calculated from an estimated value for 4-heptanone using equation 4 and the constants in Table 4.
b Statistically corrected for two isomerizing alkyl groups.
of these entities produces enthalpies of isomerization for the corresponding alcohols of8.1 š 1.0 kJ mol 1 and for the corresponding alkanes of 6.8 š 1.3 kJ mol 1. This is perhaps not the best comparison of substituent isomerization effects because in the aldehydes the isomerization is from an n-primary to a secondary substituent attached to the carbonyl group, while in the alcohol the isomerization is from an n-primary to a branched primary substituent group attached to the hydroxyl group. The more closely related enthalpy of isomerization103 of n-propanol to isopropanol is 17.7š0.7 kJ mol 1.
Two ketone pairs provide experimental data for an n-primary-to-secondary isomerization but both contain only propyl substituents. Estimating the enthalpy of formation of 4-heptanone from equation 4 and the constants in Table 4 gives a third pair. All of these enthalpies of isomerization are less negative than the propyl isomerization in aldehydes. Ketones, more stable than aldehydes generally, thus seem less susceptible to the stabilizing effect of increased branching in the substituent group, although they are stabilized more than aldehydes per CH2 group. The propyl isomerization enthalpy is somewhat affected by the nature of the other substituent bonded to the carbonyl (Me, Et or n-Pr). One ketone pair yields a single n-butyl-to-tert-butyl isomerization enthalpy, while a second pair gives us partial insight into the effects of di-tertiary branching and steric hindrance: the gaseous enthalpy of isomerization of di-n-butyl ketone to di-t-butyl ketone is only0.9 š 1.8 kJ mol 1. Although there is an experimental enthalpy of formation for ethyl t-butyl ketone, there is none for its isomer, 3-heptanone. We can roughly estimate a value for the latter from the methylene enthalpy increment between 3-pentanone and 3-hexanone which is 20.4 kJ mol 1. Using the derived enthalpy of formation ( 298.7 kJ mol 1), the approximate gaseous enthalpy of isomerization for the ethyl butyl ketone pair is ca15 kJ mol 1, compatible with that of the methyl butyl pair in Table 5 and presumably not too different from one butyl isomerization in di-n-butyl ketone. Thus, if ca15 kJ mol 1 is released during the first rearrangement to the more stable tertiary substituent with presumably minimal steric interference, then ca C14 kJ mol 1 is required for the second rearrangement. To produce such a net endothermicity in the hypothetical second isomerization, the enthalpy effect of unfavourable steric hindrance must be about C29 kJ mol 1, assuming additivity of the favourable branching effect. Even if the first butyl isomerization is closer to 11 kJ mol 1 (as for the gaseous methyl butyl ketones), the endothermic steric enthalpy would be about C21 kJ mol 1. To assess the importance of tertiary branching (even though not adjacent to the functional group) but with a presumably smaller steric effect, consider the isomerization of di-n-pentyl ketone to di-neopentyl
ketone: Hisom(g) D 33.8 š 3.0, or 16.9 for each pentyl group rearrangement, similar to the single primary-to-tertiary isomerization enthalpy derived above.
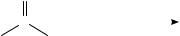
578 |
Suzanne W. Slayden and Joel F. Liebman |
The more negative enthalpies of isomerization of ethers are presumably a consequence of the greater electronegativity of the oxygen atom compared to the carbonyl group103. For example, the gaseous enthalpy of isomerization for the methyl propyl ethers is 13.8 š 1.2 kJ mol 1 and that for methyl n-butyl to methyl t-butyl ether is 25.4 š 1.6 kJ mol 1. The magnitude of the steric interaction in the ether can be assessed in the same way as for the ketones. Tertiary branching of the first primary group in di-n-butyl ether is favourable by 27.6 kJ mol 1. Assuming additivity of the branching enthalpy104, the total isomerization of di-n-butyl ether to di-t-butyl ether would be 55.2 kJ mol 1. That the actual enthalpy of isomerization is 28.6 kJ mol 1 means that C26.6 kJ mol 1 are lost, presumably to destabilizing steric interactions. The methyl ether series provides the only example of an n-pentyl to t-pentyl isomerization, 26.8 š 2.0 kJ mol 1, which is indistinguishable from the single butyl isomerization in di-n-butyl ether. From this comparison of ketones and ethers, it seems the steric effects on the enthalpy of isomerization are nearly the same in the two families of compounds.
We now reconsider from a previous section the multiple gaseous enthalpies of formation available for the 1,1-disubstituted ethenes corresponding to the aforementioned ethyl and methyl ketones with one branched substituent: 2,3-dimethyl-1-butene6,26,32, 2-ethyl- 3-methyl-1-butene16,28 and 2,3,3-trimethyl-1-butene21,27. The calculated ranges for the alkene enthalpies of isomerization for the methyl/propyl and ethyl/propyl substituted pairs were respectively 3.5š1.6 to 7.4š2.3 kJ mol 1 and 2.8š2.6 to 8.2š3.0 kJ mol 1, and for the methyl/butyl pair, 6.8 š 1.8 to 11.4 š 1.8 kJ mol 1. If ketones are analogous to aldehydes with respect to their larger exothermicity of isomerization relative to alkenes, then the H2CDC(CH3)Pr enthalpy of isomerization value of 3.5 kJ mol 1, at the lower end of the range (compared to 3.5 š 1.3 kJ mol 1 for the corresponding ketones), is more accurate26. For the H2CDC(CH2CH3)Pr and H2CDC(CH3)Bu alkenes, no choice can be made because any of the isomerization enthalpies could be rationalized as correct. There are no data for the corresponding 1,1-disubstituted ethenes to compare with the enthalpies of isomerization for the other ketones.
C. Enthalpies of Reaction
The chemical reactivity of the carbonyl group is such that several reaction types are applicable to exploring the energetics of aldehydes and ketones105,106. It might be interesting and useful here to computationally interrelate a few common carbonyl reactions especially as we continue to investigate the behaviour of homologous series with respect to the constancy, or lack of it, in their interconversions.
A typical reaction of aldehydes and ketones is addition to the C O bond. Examples of addition reagents are H2 (resulting in reduction to the corresponding alcohol), ROH (to give a hemiacetal or hemiketal) and RM (yielding a metal alkoxide). Only the hydrogenation reaction produces an addition product for which there is any useful quantity of thermochemical data, however. Equation 34 represents an overall reaction of the carbonyl compound with a (hypothetical) reagent XY, an equation which includes any reaction, subsequent to an initial addition reaction, to form products for which there are sufficient data.
O |
|
|
|
|
|
|
OY |
|
||
C |
+ X |
|
Y |
|
|
|
|
|
|
(34) |
|
|
|
|
C |
|
|||||
|
|
|
|
|||||||
|
|
|
|
|
|
|
|
|
|
|
|
|
|
|
|
|
|
X |
|
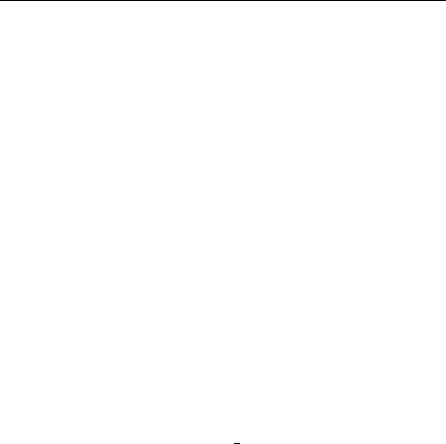
11. Thermochemistry of olefins, carbonyl compounds and imines |
579 |
|||||||
TABLE 6. Linear analysis of equation 36 for several carbonyl addition reactions (kJ mol 1)a |
||||||||
Carbonyl compound |
|
|
Product |
m(l) |
‘ Hrxn’(l) |
m(g) |
‘ Hrxn’(g) |
|
>CDO |
|
|
>C(OX)Y |
|
|
|
|
|
|
|
|
X |
Y |
|
|
|
|
|
|
|
|
|
|
|
|
|
n-R(CDO)H |
|
|
H |
H |
0.955 š 0.005 |
73.3 š 1.6 |
0.966 š 0.006 |
60.8 š 1.7 |
[R D Me, Et, Pr, Hex] |
|
0.967 š 0.005 |
116.0 š 1.6 |
0.925 š 0.025 |
86.0 š 7.2 |
|||
n-R(CDO)H |
|
|
H |
CH3 |
||||
[R D Me, Et, Pr] |
|
|
|
|
0.989 š 0.015 |
223.7 š 6.9 |
|
|
n-R(CDO)H |
|
|
CH3 |
OCH3 |
|
|
||
[R D Me, Et, Pr] |
|
|
|
|
|
|
|
|
n-R(CDO)Me |
b |
] |
H |
H |
0.998 š 0.007 |
69.0 š 2.6 |
0.997 š 0.037 |
54.2 š 10.9 |
[R D Me, Et, Pr, Bu |
|
|
1.250 |
200.8 |
1.168 |
147.6 |
||
n-R(CDO)Me |
|
|
H |
CH3 |
||||
[R D Me, Et] |
|
|
|
|
1.019 š 0.013 |
221.2 š 6.4 |
|
|
n-R(CDO)Me |
|
|
CH3 |
OCH3 |
|
|
||
[R D Me, Et, Pr] |
|
|
|
|
|
|
|
|
a The correlation coefficients for all equations are 0.999. b Enthalpy of formation (g) of 2-hexanol is not available.
For example, aldehydes and ketones react with excess alcohol to produce acetals or ketals, and organometallic additions followed by hydrolysis produce alcohols. The formal reagents X Y for formation of acetals/ketals and substituted alcohols are thus ROR and RH, respectively. Equation 34 is a clean chemical equation, as opposed to the real chemical reactions with the accompanying complicating effects of reagents, by-products and solvents. Equation 35 expresses the reaction thermochemically.
Hrxn D Hf[>C(OY)X] f Hf[>CDO] C Hf[XY]g |
35 |
Since Hf [XY] is a constant in Equation 35, we can further simplify the analysis by not including it and writing Equation 36:
Hf[>CDO] D mž Hf[>C(OY)X] ‘ Hrxn’ |
36 |
Its obviously linear form lends itself to analysis by the plotting and regression techniques introduced previously for equations 7 and 11. For homologous series in which the enthalpy of reaction is more-or-less constant for all members, the slope of equation 36 is close to 1 and the y-intercept, ‘ Hrxn’, is approximately equal to the mean experimental enthalpy of reaction, Hrxn. The direction of deviation of the slope from unity for any carbonyl/product pair indicates whether the hypothetical reaction enthalpy will increase or decrease for the series. Inclusion of the enthalpies of formation of the appropriate XY reagent (or a combination of reagents and/or by-products) shows whether the reaction is actually endoor exothermic. Some results are summarized in Table 6.
1. Reduction to alcohols
In the hydrogenation reduction reaction of non-branched aldehydes to primary alcohols, for both liquids and gases the enthalpy of hydrogenation becomes more negative with increasing nc. The range of gaseous reaction enthalpy is 69.1 to 72.6 kJ mol 1 for acetaldehyde through heptanal. With increasing nc, the alcohol becomes slightly more stabilized than does the aldehyde from which it is formed. The hydrogenation enthalpy of the branched aldehyde, 2-methylpropanal, is 1 2 kJ mol 1 less negative than its isomer.
For methyl n-alkyl ketones, both liquid and gaseous enthalpies of hydrogenation to secondary alcohols107 are essentially constant, as predicted by the almost unit slope of

580 Suzanne W. Slayden and Joel F. Liebman
equation 36 [mean values: Hrxn(l) D 69.8š 0.33; Hrxn(g) D 55.1š 0.8 kJ mol 1]. The reduction of 3-methyl-2-butanone is ca 3 kJ mol 1 less exothermic than the reduc-
tion of 2-pentanone, its unbranched isomer. |
Almost all of this difference is due to |
the 3.5 kJ mol 1 difference in stability of |
the two gaseous methyl ketones because |
the stability of the two alcohol reduction products are nearly identical [ Hisom(g) D0.6 kJ mol 1]. This contrasts with the one example of an n-alkyl sec-alkyl ketone, 2-methyl-3-pentanone, whose enthalpy of hydrogenation is virtually identical to its di-n- alkyl isomer, 3-hexanone. (There are not enough alcohol data to assess the other di-n-alkyl ketone reduction enthalpies.) However, the enthalpy of reduction of liquid 3,3-dimethyl-
2-butanone ( 105.4 kJ mol 1) is much greater than for the other ketones. This is because the difference in stability of liquid 3,3-dimethyl-2-butanol17b (ca 42 kJ mol 1) and its unbranched isomer is very much greater than the stability difference for the corresponding ketones ( 6.6 kJ mol 1).
Hydrogenation of an aldehyde or ketone results in the corresponding primary or secondary alcohol; a Grignard reaction with methylmagnesium iodide, for example, followed by hydrolyis produces a secondary or tertiary alcohol with a methyl group bonded to the carbinol. The overall reaction is tantamount to adding CH3 H to the carbonyl bond. This enthalpy of reaction for aldehydes to the corresponding 2-alkanols is slightly increasingly negative with larger nc as it was for the hydrogenation, but for the ketones, the exothermic enthalpies of reaction decrease substantially with nc. The range for C2 C4 gaseous aldehydes is 106.7 to 109.8 kJ mol 1 and the values for gaseous acetone and 2-butanone are 95.2 and 92.1 kJ mol 1, respectively. Both of these results are predicted by a comparison of the relevant slopes generated from equation 36. The aldehydes are reduced to the secondary alcohols of slightly steeper slope (ca 20.1) and so the difference between them increases with nc. The ketones are reduced to tertiary alcohols with a less steep slope (ca 18.3) and so the difference decreases with increasing nc. The reaction enthalpy for 2-methylpropanal is fully 10 kJ mol 1 less exothermic than its isomer, while the reaction enthalpy for 3-methyl-2-butanone, 94.5 kJ mol 1, is presumably more exothermic than that of its unbranched isomer.
2. Acetal and ketal formation
Most measured acetal and ketal enthalpies of formation are for the dimethyl derivatives in the liquid phase. The slopes of equation 36 for the formation of 1,1-dimethoxyalkanes108 from aldehydes and for the formation of 2,2-dimethoxyalkanes109 from methyl ketones are in the region where constancy of the enthalpy of reaction is indeterminate. Although
the acetalization for nc |
D 2 |
|
4 is overall increasingly exothermic (mean D 228.4 š |
||
|
|||||
0.5 kJ mol 1), and the |
ketalization for nc D 3 |
|
5 is overall decreasingly exothermic |
||
|
(mean D 212.2 š 0.6 kJ mol 1) as predicted by their slopes, neither enthalpy range is large enough, nor are there data enough, to define a trend. The ketalization of methyl isopropyl ketone is about 1 kJ mol 1 more negative than ketalization of its n-propyl isomer while the ketalization of methyl t-butyl ketone is about 15.6 kJ mol 1 more positive than ketalization of its n-butyl isomer110. This large difference is due mainly to the endothermic isomerization of 2,2-dimethoxyhexane to 2,2-dimethoxy-3,3-dimethylbutane (ca C9 kJ mol 1).
3. Methylenation
Earlier we compared the enthalpies of isomerization of small substituent groups in aldehydes and ketones with those of the correspondingly substituted alkenes. We now
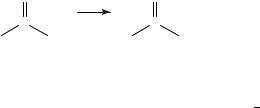
11. Thermochemistry of olefins, carbonyl compounds and imines |
581 |
consider the enthalpy of reaction which converts the carbonyl-containing compounds into those alkenes. The overall reaction may be written as
O |
CH2 |
C |
(37) |
C |
which corresponds to, for example, a Wittig reaction with methylene triphenylphosporane, Ph3PDCH2 (the reverse of equation 37 might be an ozonolysis reaction). Since any reactants and/or by-products for this reaction will be constants in the thermochemical equation, we can restrict consideration to just the alkene and the carbonyl, as in equation 36. The n-aldehydes exhibit a decreasing endothermicity of methylenation (nc D 2 4, 7) with a range of 186.1 to 182.4 kJ mol 1. The enthalpy of reaction for 2-methylpropanal is 4.8 kJ mol 1 more endothermic than its unbranched isomer. Immediately we see that the reaction enthalpy should be constant for the methyl ketones and the 2-methyl-1-n-alkenes since their slopes from equation 4a are virtually identical (Tables 2 and 4). Indeed, from
a linear regression of equation 36 the slope is 1.008 and the y-intercept ( ‘ Hrxn’) is 200.2. The average enthalpy of reaction calculated from the experimental enthalpies of
formation is the nearly constant C200.6 š 0.3 kJ mol 1. The reaction enthalpies of the two 3-n-alkanones are also constant and only 1 kJ mol 1 more endothermic.
When the enthalpies of reaction between branched ketones and the corresponding 1,1- disubstituted alkenes are calculated using the multiple enthalpies of formation available for the latter, the following ranges are obtained: Me/i-Pr, 196.6 to 200.5; Et/i-Pr, 201.2 to 206.6; and Me/t-Bu, 200.5 to 205.1 kJ mol 1. Perhaps it is reasonable to conclude that the reaction enthalpies for the branched compounds either will be approximately constant, as for the unbranched ketone/alkene conversions, or will be more endothermic with branching, as in the branched aldehyde/alkene conversions. In either case, the least endothermic reaction enthalpy for the Me/i-Pr conversion above seems inconsistent and therefore the enthalpies of formation for 2,3-dimethyl-1-butene from References 16 or 26, which are essentially identical, should be selected. These enthalpies were also selected in a previous section. However, there is too much inconstancy, as well as too much uncertainty, in the replacement reactions of carbonyls and olefins to be more definitive in our conclusions.
IX. ALICYCLIC KETONES
A. Monocyclic Ketones
We start with the unsubstituted cycloalkanones (38), compounds of the generic formula cyclo-[(CH2)n 1CO]. The gas-phase enthalpies of formation of all of these species from n D 3 to 12, 15 and 17 are available from experiment. An obvious comparison is with the corresponding n-membered ring cycloalkanes, cyclo-[(CH2)n]. Consider equation 38 in which the difference quantity υ38 n is defined as the difference of the gas-phase enthalpies of formation of the ketone of interest and the related cycloalkane.
υ38 n H°f cyclo-[ CH2 n], g H°f cyclo-[ CH2 n 1CO], g 38
The numerical values of the enthalpies of formation of the various cycloalkanones and the corresponding υ38 n values are found in Table 7.
The values of υ38 n almost all lie between 100 and 150 kJ mol 1. The two exceptions to that are the n D 3 case, cyclopropanone, and the n D 17 case, cycloheptadecanone. That the first is deviant is consistent with the ca 50 kJ mol 1 general additional destabilization
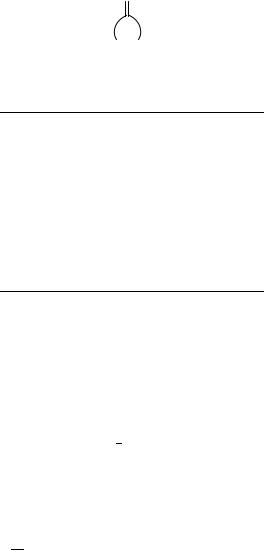
582 |
Suzanne W. Slayden and Joel F. Liebman |
O
(CH2 )n-1
(38)
TABLE 7. Enthalpies of formation of gaseous cycloalkanones and the corresponding values of υ38 n
n |
Hf (g) |
Reference |
υ38 n |
|
3 |
16 š 4 |
a |
37 š 4 |
|
b |
||||
4 |
101.3 š 1.3 |
b |
129.7 š 1.5 |
|
5 |
194.8 š 1.7 |
b |
118.4 š 1.9 |
|
6 |
227.8 š 1.9 |
b |
104.4 š 2.1 |
|
7 |
248.1 š 1.3 |
b |
130.0 š 1.6 |
|
8 |
272.2 š 1.8 |
b |
147.8 š 2.1 |
|
9 |
279.7 š 1.7 |
b |
146.9 š 2.3 |
|
10 |
305.1 š 1.9 |
b |
150.8 š 2.5 |
|
11 |
322.0 |
š 2.2 |
b |
142.6 š 2.8 |
12 |
349.1 |
š 2.3 |
c |
118.9 š 2.6 |
15 |
414.5 |
š 9.2 |
c |
113.1 š 9.4 |
17 |
460.3 |
š 10.9 |
|
96.0 š 11.2 |
a See Reference 111 for cyclopropanone.
b See Reference 112 for the cycloalkanone data. c See Pedley.
of three-membered rings upon introduction of a trigonal carbon52. This correction results in the value for the υ38 n of cyclopropanone to appear ‘almost normal’, and thereby legitimizes the enthalpy of formation as well despite its unconventional non-calorimetric, origin113. The n D 17 case is not sorely out of line, but we note that doubts have been raised about the accuracy of the measurement of the enthalpy of formation of the appropriate cycloalkane, cycloheptadecane114. Although the two outliers have now been tended to, no apparent pattern in the 100 150 kJ mol 1 difference quantity, υ38 n , is to be found. Nonetheless, the cyclopropanone case aside, low values seem to accompany the most strain-free cycloalkanes, n D 6 and 17.
B. Bicyclic and Polycyclic Ketones
For these species, we consider the difference quantity υ39 which is defined as the difference of the gas-phase enthalpies of formation of the ketone of interest and the related hydrocarbon there is no single parameter such as the ring size n to provide a conceptual framework. Letting * denote the phase,
υ39 Ł Hf° >CH2, Ł Hf° >CO, Ł |
39 |
(If no phase is explicitly given, the reader is implicitly told that the data and the difference quantity are for the gas.)
We thus start with the smallest and simplest systems for which we have thermochemical data, the isomeric 2- and 7-bicyclo[2.2.1]heptanones (the norbornanones, 39a and 39b). Our intuition suggests that the latter species is more strained since the increased geometric constraints of a single-carbon bridge over those for a two-carbon bridge are
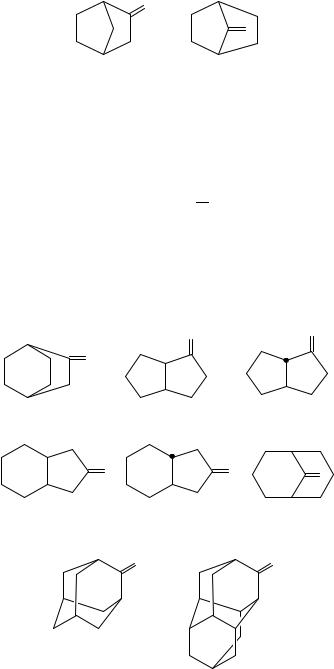
11. Thermochemistry of olefins, carbonyl compounds and imines |
583 |
|
|
O |
|
|
O |
|
(39a) |
(39b) |
|
expected to be more sterically severe or destabilizing for a trigonal than tetrahedral carbon because of the larger endocyclic >C angle for the former115. This is realized by the enthalpy-of-formation data on these bicycloheptanones: the former ketone is more stable than the latter by 36.5 š 5.8 kJ mol 1. The enthalpy of formation for the single bicycloalkane which corresponds to both ketone isomers is 54.9 š 4.7 kJ mol 1 from which we find υ39 values of 115.7 š 6.8 and 79.2 š 5.6 kJ mol 1, respectively. Unlike the case for the monocyclic ketones, the smaller value of υ39 is found for the more strained ketone. This value is not merely ‘smaller’ it is quite astonishing how small υ39 is for 7-bicyclo[2.2.1]heptanone when compared with any of the monocyclic ketones or its 2-isomer.
Consider now bicyclooctanones. Of the nine possible species, there are three for which the desired thermochemistry is available in the literature: bicyclo[2.2.2]octanone116,117, and the cis- and trans-bicyclo[3.3.0]octan-2-ones, species 40a, 40b and 40c,
respectively. We find the |
following υ39 values: |
119, |
137.2 š 5.3 and 140.1 š |
|
O |
|
O |
|
O |
|
|
(40a) |
(40b) |
|
(40c) |
|
O |
O |
O |
(41a) |
(41b) |
|
(41c) |
|
O |
|
O |
(42) |
(43) |
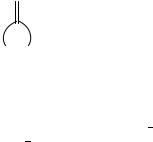
584 |
|
Suzanne W. Slayden and Joel F. Liebman |
|
|||
5.4 kJ mol 1. Interestingly, the υ39 values |
for the two |
bicyclo[3.3.0]octanones |
are |
|||
quite comparable |
despite |
the ca 25 kJ mol 1 |
difference in |
strain between these latter |
||
ketones. |
|
|
|
|
|
41a |
Bicyclononanones |
are |
represented by cis- and trans-hexahydro-2-indanone, |
||||
and 41b, which |
like |
the |
above bicyclo[3.3.0]octan-2-ones |
are fused cyclopentanones. |
The values of υ39 are 122.5 š 2.4 and 117.8 š 2.7 kJ mol 1. Another example is bicyclo[3.3.1]nonan-9-one126 (41c), for which υ39 equals ca 112 kJ mol 1.
We now consider adamantanone (42) and diamantanone (43). The two values of υ39 are rather close, 96.0 š 5.1 and 90.6 š 3.2, and both values are rather much smaller than for their monocyclic six-membered ring relative, cyclohexanone (104.4 š 2.1 kJ mol 1).
C. Comparison of Alicyclic Ketones and Their Methylene Analogs
Before commencing our comparison we now define the methylene analog of a ketone to be that species in which the >CDO of the former is replaced by the isoelectronic and isosteric >CDCH2 group, the erstwhile Wittig reaction of a previous section. Starting with 1-ring species, it might have been thought that these methylenecycloalkanes (18b, now redrawn as 44, cyclo-[(CH2)n 1CDCH2]) would be good ‘mimics’ of the cycloalkanones. After all, they both have a trigonal carbon replacing a >CH2 in the alicyclic cycloalkane ring. Consider now the difference quantity υ40 n which is defined as the difference of the gas-phase enthalpies of formation of the cycloalkanones and the related methylenecycloalkanes:
υ40 n H°f [cyclo-[ CH2 n 1CDCH2, g] H°f cyclo-[ CH2 n 1CO] , g] 40
(CH2 )n-1
(44)
The numerical values of the enthalpies of formation of the cycloalkanones and the corresponding υ40 n values are found in Table 8. Admittedly, the enthalpies of formation of the desired gas-phase methylenecycloalkanes are only available for n D 3 7. However, while the difference, υ40 n , clusters between 200 220 kJ mol 1, a pattern is disappointingly just as absent for the derived values of υ40 n as for those of υ38 n .
The thermochemical data are likewise sparse for the methylene derivatives of the bicyclic and polycyclic ketones. For these species, we consider the difference quantity υ40 which is defined as the difference of the gas-phase enthalpies of formation of the ketone of interest and the related hydrocarbon:
υ34 Hf° >CDCH2, g Hf° >CO, g |
41 |
We thus start with the smallest and simplest systems. For bicyclo[2.2.1]heptan-7-one, υ41 is deduced to be 194.2 š 3.8 kJ mol 1. No immediate comparison from experiment is available for the -2-one and its methylene analog for the gas-phase enthalpy of formation of the latter species, 2-methylenebicyclo[2.2.1]heptane (45a), remains unmeasured for want of the enthalpy of vaporization. We are not, however, particularly thwarted. From our standard enthalpy-of-vaporization protocol, we would predict a value of 40 kJ mol 1,
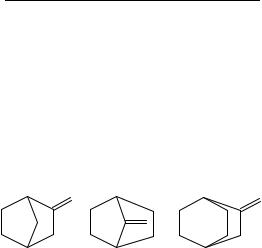
11. Thermochemistry of olefins, carbonyl compounds and imines |
585 |
|||||
|
TABLE 8. Enthalpies of formation of gaseous cycloalka- |
|
||||
|
nones and the corresponding values of υ40 n |
|
|
|
||
|
n |
Hf°(g) |
Reference |
υ40 |
|
|
3 |
16 š 4 |
a |
184 š 4 |
|
||
b |
|
|||||
4 |
101.3 š 1.3 |
b |
222.8 š 1.5 |
|
||
5 |
194.8 š 1.7 |
b |
206.8 š 1.8 |
|
||
6 |
227.8 š 1.9 |
c |
202.6 š 4.3 |
|
||
7 |
248.1 š 1.3 |
|
210 |
|
|
a See Reference 111 for cyclopropanone.
b See Reference 112 for the cycloalkanone data.
c The enthalpy of formation of cycloheptanone was obtained from Reference 112. The enthalpy of formation of methylenecycloheptane was obtained by combining the directly measured enthalpy of hydrogenation (Reference 22) to form methylcycloheptane and the suggested gas-phase enthalpy of formation of this latter hydrocarbon from Reference 8.
nearly identical to that of the vaporization enthalpy for the 7-methylene isomer (45b) from the experimental literature. From this we deduce a value of 206 kJ mol 1 for υ41. The υ41 value for methylenebicyclo[2.2.2]octane (46) is 213.0 š 6.0 kJ mol 1. No further comparison is now possible for there are no reported enthalpies of formation of the remaining methylene derivatives of any of the bicyclic or polycyclic ketones for comparison.
(45a) |
(45b) |
(46) |
D. Methylated Cyclic Ketones
Ketones are a relatively common and widespread class of natural products: we recognize camphor, carvone, chlorophyll, cortisone, estrone, υ-ketolevulinic acid and testosterone as examples. We now discuss if there are any simple rules for estimating the enthalpies of formation of cyclic ketones upon methylation. There are problems as soon as we consider monocyclic species. Using solely liquid-phase data so as not to need estimates of enthalpies of vaporization, we find 2-methylation of cyclopentanone is accompanied by the enthalpy of formation becoming more negative by 29.9 š 5.7 kJ mol 1 while the corresponding 2-methylation of cyclohexanone results in a change of but 17.9 š 3.9 kJ mol 1. Trans-hexahydro-2-indanone and its 8-methyl derivative (47) differ by 28.2š2.7 kJ mol 1. A difference of 25 kJ mol 1 allows for rough consistency between all three results. The enthalpy of formation changes upon methylating cyclopentane, cyclohexane and trans-decalin (on C2) are 32.8 š 1.1, 33.7 š 1.3 and 34.3 š 2.9 kJ mol 1. Consistency is achieved with a difference of 34 kJ mol 1. We are thus forced to conclude there is a ca 9 kJ mol 1 difference between the enthalpies of methylation of cyclic ketones and of cycloalkanes. Alternatively, it is more plausible that the reported enthalpy of formation of 2-methylcyclohexanone is in error. However, it is disturbing because this is a ‘normal’ compound that we would not have expected to be plagued by calorimetric complications.
Let us briefly consider camphor (48) and compare it with its ‘nor’ derivative, bicyclo[2.2.1]heptan-2-one that lacks its 1,7,7-trimethyl substitution. The enthalpies of
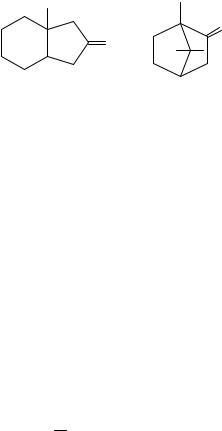
586 |
Suzanne W. Slayden and Joel F. Liebman |
O
O
(47) |
(48) |
formation of both of these substances are available both as solids and gases. We want liquids here, and so need to correct either the solidor gas-phase data. The latter is simpler: direct application of the logic in Reference 118 would suggest that the enthalpy of vaporization of their putative liquid forms would differ by the contribution of one non-quaternary carbon and two quaternary carbons, namely 7 kJ mol 1. Accordingly, the difference of formation of the gas-phase compounds, 96.9 š 5.6 kJ mol 1 ‘favoring’ the trimethylated species, gets amplified to ca 104 kJ mol 1 for the liquids. If it is assumed that introduction of the 1-methyl and the two 7-methyls into the ‘nor’ species corresponds to methylation of a ketone and a cycloalkane respectively, the difference would have been ca 93 kJ mol 1. Given we have blithely ignored any strain incumbent on methylation, that the difference between the two values is but 11 kJ mol 1 is encouraging.
E. Solid Cyclic Ketones
What can be said about the thermochemistry of methylated cyclic ketones in any phase other than liquid? In that we would have discussed gas-phase species if the data were available, what we are really asking is: ‘what can be said about the species of interest as solids?’, Let us generalize this to see what can be said about the thermochemistry of cyclic ketones as solids. In that the cyclic ketones in the previous section were all formally quite strain-free derivatives of cyclopentanone or cyclohexanone, let us now consider only such species and their cycloalkane analogs. There are no enthalpy-of-fusion data available for cyclopentanone. For cyclohexanone, the temperature-uncorrected fusion enthalpy is about 1.3 kJ mol 1 we arbitrarily ignore in this discussion enthalpies of any crystal ! different crystal phase enthalpy. The same treatment for cyclohexane discloses the fusion enthalpy of 2.7 kJ mol 1. Accordingly, the earlier reported value of υ38 6 D 104.4 for the gas phase is sequentially modified to ca 115 kJ mol 1 for the liquid and to 113 kJ mol 1 for the solid. For 2-norbornanone, adamantanone and diamantanone (39a, 42 and 43), the gas-phase differences of 115.7 š 6.8, 96.0 š 5.1 and 90.6 š 3.2 become for the solids 124.4 š 6.8, 116.9 š 4.5 and 97.8 š 3.1 kJ mol 1. Very roughly, υ39(*) is some 10 kJ mol 1 more positive for solids than for gases and υ39(s) is about 113 kJ mol 1 for all of the solids discussed here.
The above is such a coarse analysis that it may appear unlikely it could be of any use until it is remembered that many compounds of interest lack thermochemical data except as solids. Agreement with our suggested difference quantity suggests ‘consistency’ and ‘plausibility’. Marked discrepancy from our suggested difference quantity suggests either error or a ‘new phenomenon’. To illustrate this, consider the polycyclic hydrocarbon 5˛-androstane (49a) and its 3,17-dione 49b. The enthalpies of formation of both were reported nearly 30 years ago119 and chronicled by Domalski, but ignored by Pedley. These values, 314 and 544 kJ mol 1 respectively, result in the normalized value (i.e. divided by the number of keto groups) of υ39(s) of 115 kJ mol 1. We thus conclude that these data are, at least, consistent and plausible. An example of the latter