
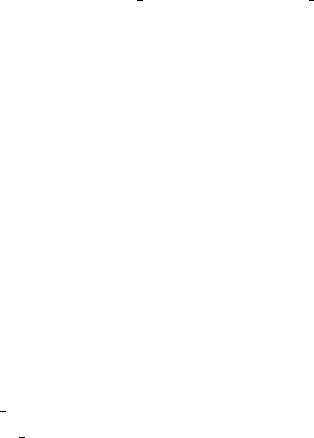
11. Thermochemistry of olefins, carbonyl compounds and imines |
547 |
or equation 7 shows, as do the uncertainties associated with the regression constants, that at least one of the three data points needs adjustment. A lesser problem confronts us with the data for the cis-3-alkenes because the enthalpies of formation for C6H12 and for C7H14 from various sources are the same within experimental error. The mean values are:
C6H12 D 46.6 š 1.8 16,26,31 and C7H14 D 68.8 š 1.7 21,27,30. Again there is only one C8H16 value29.
Figure 1 is a plot of equation 7 which helps visualize the results in Table 2 for the gaseous n-alkene homologous series. Although only the gaseous phase plot is presented, that for the liquid phase is almost identical except for scale and the absence of data for ethene, propene and the octenes. For visual clarity in Figure 1, lines connect the data points for the 1-n-, cis- and trans-2-n-alkene isomers only. Unlike in some other homologous series44, the methyl-substituted functional group, CH3CHDCH2, does not deviate from the linear relationship. Relative to the other alkenes, ethylene is destabilized, with an enthalpy of hydrogenation16 of 136.3 kJ mol 1 substantially more negative than the other alkenes. Its deviation from the 1-n-alkene homologous series is analogous to the deviation observed for any H Z compound compared to the R Z homologous series45 and so it was not included in any of the results presented here.
2. Branched monosubstituted alkenes
It is clear from equation 7 that we should not consider as one structural category all those monosubstituted alkenes with branching in the substituent group and report an average enthalpy of hydrogenation for the group. Instead, we wish to discriminate among those subcategories wherein the branching position is progressively closer to the double bond.
In the homologous series of gaseous isoalkyl-1-alkenes, a methyl group is substituted on the penultimate carbon. The equation 4a best straight line for the four members of the series incorporates the sole C5H10 and C8H16 points from References 26 and 29. The C7H14 data16,28, which vary by 4.3 kJ mol 1, lie on either side of the line. The average enthalpy of formation for 5-methyl-1-hexene from these two data (g) is 67.9 š 1.5 kJ mol 1 while the interpolated value is 68.7 kJ mol 1. Using the average value in the regression analysis significantly degrades the correlation of equation 4a.
Three branched 1-alkenes with a sec-butyl group remote from the double bond constitute another homologous series. Among the gaseous enthalpies of formation for 3-methyl-1-pentene, 4-methyl-1-hexene and 5-methyl-1-heptene derived from measured enthalpies of hydrogenation26,27,29, one of the points is a slight outlier and the correlations from either equation 4a or equation 7 are not satisfactory. Using an alternate value16 for 3- methyl-1-pentene which is 2 kJ mol 1 more negative produces a three-point series which is profoundly linear by either equation 4a or equation 7.
The 3-methyl-1-alkenes could seemingly constitute yet another series. The enthalpies of formation for (C5H10)16, (C6H12)26 and (C7H14)27 are well-correlated both with carbon number (equation 4) and with the corresponding alkanes (equation 7). Extended analysis of the alkanes reveals, however, that the first member of the corresponding alkane series, 2-methylpentane, is actually an outlier on the otherwise straight line incorporating the C6 C8 3-methylalkanes. Had an enthalpy value for 3-methyl-1-heptene been measured, the non-linearity would be apparent in a degradation of the alkene correlation. Of course, the C6 C8 3-methyl-1-alkenes and -alkanes do form homologous series, but, lacking the C8H16 enthalpy, we will not perform a regression analysis on the two-point line. This example serves as a reminder that not only must there be data of good quality but also data in sufficient quantity. We are cognizant that a new measurement for a member of the
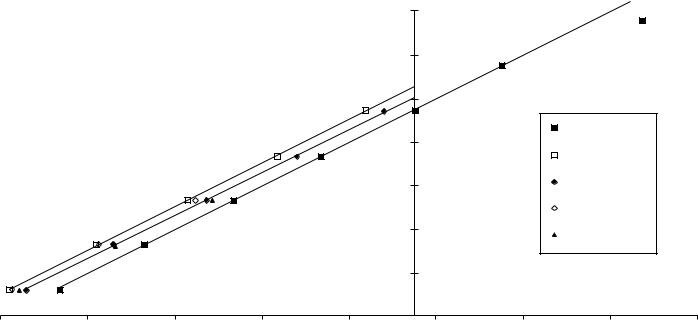
|
|
|
|
|
|
|
|
548 |
|
|
|
|
|
−80 |
|
|
C2 |
|
|
|
|
|
−100 |
|
|
|
|
|
|
|
|
|
C3 |
|
|
) |
|
|
|
|
|
|
|
|
−1 |
|
|
|
|
−120 |
|
|
|
(kJ mol |
|
|
|
|
|
|
|
|
|
|
|
|
C4 |
|
1-n-alkenes |
|
|
alkanes |
|
|
|
|
−140 |
|
|
|
|
|
|
|
|
|
trans-2-alkenes |
|
|
|
|
|
C5 |
|
|
|
|
|
of |
|
|
|
|
|
|
|
|
|
|
|
|
−160 |
|
cis-2-alkenes |
|
|
of formation |
|
|
|
|
|
|
||
|
|
C6 |
|
|
|
trans-3-alkenes |
|
|
|
|
|
|
|
|
|
||
|
|
|
|
−180 |
|
cis-3-alkenes |
|
|
Enthalpy |
|
|
|
|
|
|
|
|
|
C7 |
|
|
|
|
|
|
|
|
|
|
|
−200 |
|
|
|
|
|
|
|
|
|
|
|
|
|
|
C8 |
|
|
|
−220 |
|
|
|
−95.0 |
−75.0 |
−55.0 |
−35.0 |
−15.0 |
|
|
|
|
5.0 |
25.0 |
45.0 |
65.0 |
Enthalpy of formation of alkenes (kJ mol−1)
FIGURE 1. Enthalpies of formation of alkanes (g) vs alkenes (g)

11. Thermochemistry of olefins, carbonyl compounds and imines |
549 |
series or a measurement for a new member in a series, especially if it is a fourth member, could materially affect our choice of data to include in an analysis.
There are only two members of a homologous series containing a tertiary branched group and so we will not attempt a regression analysis. The two enthalpies of formation for 3,3-dimethyl-1-butene16,26 are essentially identical and yield an enthalpy of hydrogenation of about 125.7 kJ mol 1. The two enthalpies of formation for 4,4- dimethyl-1-pentene21,28 vary by 3.8 kJ mol 1. The derived enthalpy of hydrogenation from Reference 21 is 126.4 š 2.3 kJ mol 1 and the measured enthalpy of hydrogenation from Reference 28 is 122.6 š 0.6 kJ mol 1. We have no way of deciding between them.
3. 1,2-Disubstituted ethenes
There are enthalpy data for both geometric forms of four 1,2-disubstituted ethenes with one branch in one of the substituent groups (4-methyl-2-pentene, 4-methyl-2-hexene, 5-methyl-2-hexene and 2-methyl-3-hexene). Unfortunately, no three of these are homologous. We are additionally hampered because there are two enthalpies of formation for trans-4-methyl-2-pentene16,26 and we have no way of deciding between them. There are not even two compounds which belong to homologous series among the various more highly branched 1,2-disubstituted ethenes for which there are data. A plot of equation 7 for the cis and trans 2- and 3-alkenes, branched and unbranched, shows the branched compounds clustered around the lines describing the n-alkene homologous series (except for the sterically congested cis-4,4-dimethyl-2-pentene and cis-2,2-dimethyl-3-hexene). Thus, the enthalpies of hydrogenation of the branched alkenes are similar to, but not easily derivable from, the unbranched alkenes.
4. 1,1-Disubstituted ethenes
The unbranched 1,1-disubstituted ethenes for which there are enthalpy data belong to either of two homologous series: the 2-methyl- or 2-ethyl-1-n-alkenes. In the latter category, there are only two members, 2-ethyl-1-butene and -pentene. As such, we will not analyse their regression characteristics. A plot of equation 4a or equation 7 for the C4 C8 2-methyl-1-n-alkenes shows a straight line incorporating all the data except that the value for 2-methyl-1-butene is a too-positive outlier. Three sources16,21,32 give a very consistent value [mean Hf g D 35.3 š 0.3 kJ mol 1] for this compound, all citing a fairly recent determination46. A value estimated from equation 7 for this species is 37.6 š 0.3 kJ mol 1, a difference of 2 kJ mol 1 from the literature value. The regression analysis in Table 2 does not include either of these values. Among the 2-methyl- or 2-ethyl-1-alkenes with ethyl or methyl branches for which there are data, no three compounds are homologous.
5. Trisubstituted ethenes
Of the available trisubstituted alkenes for which we have data, only three are homologous: 2-methyl-2-butene, -pentene and -hexene. Three literature enthalpy-of-formation values16,21,32 for the C5H10 compound are well within the error bars and so we have used an average ( 41.4 š 2.0 kJ mol 1). A plot of equation 4a clearly shows the enthalpy of formation for C6H12 derived from Reference 26 to be the best value.
6. Tetrasubstituted ethenes
There are enthalpy-of-formation data for only two tetrasubstituted ethenes (2,3- dimethyl-2-butene and 2,3-dimethyl-2-pentene). Although homologous, we will not
550 |
Suzanne W. Slayden and Joel F. Liebman |
perform a regression analysis for only two members. Complicating any analysis are the four enthalpies of formation16,20,26,32 for C6H12 which span a range of 3.9 kJ mol 1.
D. Constancy of the Enthalpy of Hydrogenation
We deduced from equations 4 and 7 that only when the slope, m, is approximately equal to 1 will the enthalpies of hydrogenation for a homologous series of alkenes be reasonably constant. Inspecting the slope and intercept data from equation 7 in Table 2, together with the actual experimental enthalpies of hydrogenation, shows our conclusion to be valid. We define for each alkene homologous series
υ Hhyd D Hhyd (experimental mean) ‘ Hhyd’(y-intercept from equation 7) 8
and plot υ Hhyd versus the slope, m, from equation 7 (Figure 2). When m is between
0.999 and 1.01 (very close to 1), υ Hhyd is clustered around š0.2 kJ mol 1 and the experimental Hhyd are fairly constant. If m is less than 0.98 or greater than about 1.01,
υ Hhyd shows a steep, nearly linear increase; the experimental Hhyd changes monotonically. A particularly illustrative example is the cis-3-alkenes (m D 0.939). Although we used two composite enthalpies of formation in equations 4 and 7, the individual alkene enthalpies of formation, as determined by various methods, were quite consistent. The experimental, directly determined enthalpies of hydrogenation26,27,29 by themselves show decreasing exothermicity with increasing carbon number: 121.6 š 0.32, 118.5 š 0.3,117.8 š 0.4 kJ mol 1, differences which cannot be attributed to experimental error. Contrast those to such series as the 1-n-alkenes (m D 1.001) where the experimental enthalpies of hydrogenation fluctuate around the mean with increasing carbon number.
The foundation of these observations is the universal applicability of equation 4 to all known homologous series of organic compounds34. The regression constants in Table 2 derived from equation 4a may be used to calculate the desired enthalpy of formation for an as-yet unmeasured alkene species. The information in Table 2 also may be useful in yet another form, again derivable from equation 4. For a given homologous series, to calculate an approximate enthalpy of hydrogenation when the enthalpy of formation of neither the alkane nor the alkene is known
Hhyd D ncž ˛alkane ˛alkene C ˇalkane ˇalkene 9
E. Enthalpies of Isomerization of Alkenes
Complementary to enthalpies of hydrogenation for studying the energetics of alkenes are enthalpies of isomerization. These may be directly determined experimentally in favourable cases or derived from other enthalpies of reaction. The most accessible and general-purpose derivation is from enthalpies of formation of the alkenes of interest, as in equation 10.
Hisom D Hf[alkene(2)] Hf[alkene(1)] |
10 |
Because the enthalpies of formation of all homologous series of alkenes correlate with the number of carbons according to equation 4, any two series must correlate with each other. We can recast equation 10 in linear form as equation 11.
Hf [alkene 2 ] D m0 Hf [alkene 1 ] C ‘ Hisom’ |
11 |
Just as for the enthalpy of hydrogenation in equation 7, the enthalpies of isomerization between alkene members of two homologous series will be constant and equal to the y- intercept (‘ Hisom’) only when m0 D 1. For homologous series with non-parallel slopes
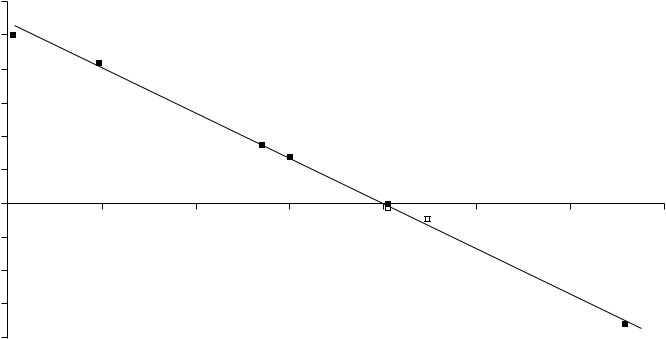
− (kJ mol 1)
6
5
4
3
2
1
hyd |
|
|
|
|
|
|
|
|
|
δ∆H |
|
|
|
|
|
|
|
|
|
0 |
|
|
|
|
|
|
|
|
|
0.92 |
0.94 |
0.96 |
0.98 |
1 |
|
|
1.02 |
1.04 |
1.06 |
|
|||||||||
|
|||||||||
−1 |
|
|
|
|
|
|
|
|
|
−2
−3
−4
FIGURE 2. The constancy of enthalpies of hydrogenation determined from equations 7 and 8
551
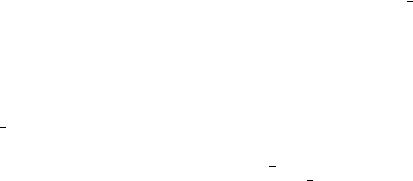
552 |
Suzanne W. Slayden and Joel F. Liebman |
generated from equation 4, the individual enthalpies of isomerization for the members will
either increase or decrease with carbon number. The slopes, ˛alkene, in Table 2 are such that some of the isomerization enthalpies of interest will be constant, but others will change
monotonically over a greater or smaller range depending on the two compared series. A perceived limitation of this analysis is that the experimental error limits generated35 from equation 10 are large enough seemingly to obscure any patterns in the enthalpies of isomerization. However, the individual data for members of homologous series are very well fitted by equation 4 and by equation 11 (where there are enough data) and it is the large correlation coefficients and the small uncertainties associated with the constants which determine the degree of confidence to be placed in the recognition of trends in the results.
An admitted limitation to analysis by equation 11 is the sparsity of data. Whereas all the corresponding alkane data were available to analyse with the alkenes in equation 7, the same is not true when we attempt to pair alkene isomers to analyse by equation 11. The regression constants are naturally affected by the different-sized data sets. An alternative equation for such circumstances is equation 12, which uses the best available data in each alkene series and avoids the problem of having to eliminate non-paired alkene data.
Hisom D ncž[˛alkene 2 ˛alkene 1 ] C [ˇalkene 2 ˇalkene 1 ] |
12 |
It would be helpful if there were more experimentally determined enthalpies of isomerization to provide an independent comparison with those derived from equation 11. The most recent ones are from References 30 and 31. However, as in the analysis of the enthalpies of hydrogenation, it is only because we can derive enthalpies of formation from enthalpies of combustion, isomerization and hydrogenation that we have a large enough database of compounds to confidently attempt an analysis. The same selected alkene enthalpies of formation are used for the analyses in this section as were used in the preceding section.
The trends in alkene isomer stability can be easily seen in plots of the enthalpies of formation versus the number of carbons, nc (equation 4). For example, in Figure 3 which includes the gaseous 1-, cis- and trans-2-, and the cis- and trans-3-n-alkenes, the relatively more negative enthalpies of formation for the 2- and 3-n-alkenes show them to be more stable than their 1-n-alkene double bond positional isomers. (Only the C4 C8 1-n-alkene members are shown for clarity.) Another well-known relationship is depicted as well: acyclic trans hydrocarbons are more stable than their cis isomers.
1. Double-bond migration in monoand di-substituted alkenes
Exploring all combinations of n-alkene isomerization reveals an apparent discrepancy within those series containing the 2-butenes. For example, consider the isomerization of the 1-n-alkenes to trans-2-n-alkenes. The individual enthalpies of isomerization (C4 C8: 11.5, 10.1, 10.6, 11.2, 11.7 kJ mol 1), or equivalently, plotting the data according to equation 11, show the butene isomerization enthalpy to be anomalously negative in this otherwise increasing series. The same anomaly is present in the enthalpies of isomerization of 1-n-alkenes to cis-2-n-alkenes (C4 C8: 7.2, 5.5, 6.2, 7.2,7.8 kJ mol 1) and of trans-2-n-alkenes to cis-2-n-alkenes (C4 C8: 4.3, 4.6, 4.5,4.0, 3.9 kJ mol 1). However, the correlations of the n-alkenes according to equation 4 do not show the C4H8 points to be outliers47. We will return to this topic after considering other isomerizations involving double-bond migration.
Figure 3 also highlights some curious data which complicates our understanding of alkene stability. That is, although the enthalpies of formation of the trans-2-n-alkenes are
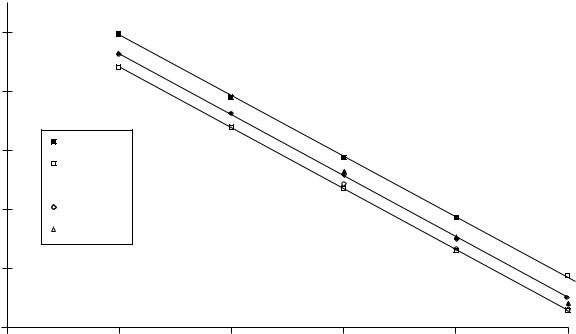
− Enthalpy of formation (kJ mol 1)
FIGURE 3.
0
−20
1-n-alkene
−40
trans-2-alkene
cis-2-alkene
−60 |
trans-3-alkene |
cis-3-alkene
−80
−100 |
|
|
|
|
|
|
3 |
4 |
5 |
6 |
7 |
8 |
|
|
|
|
Number of carbon atoms |
|
|
|
Enthalpies of formation of n-alkenes (g) vs number of carbon atoms
553
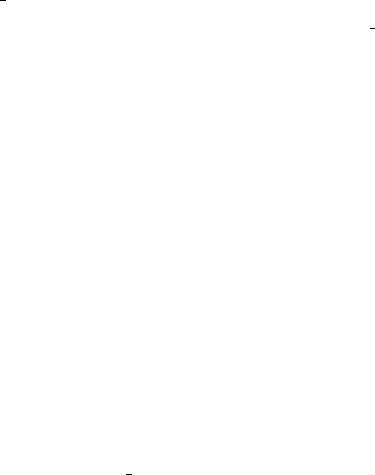
554 Suzanne W. Slayden and Joel F. Liebman
more negative than those for their trans-3-n-alkene isomers (and are thus more stable), the same is not true of the cis-2- and cis-3-n-alkenes. In those latter two series, the enthalpies of formation of the C7H14 isomers are nearly identical, cis-2-hexene is more stable than cis-3-hexene (by ca 1.5 kJ mol 1) and cis-3-octene is more stable than cis- 2-octene (by 1.6 kJ mol 1). If the lines are extrapolated, it means that for nc > 7, the cis-3-n-alkenes become increasingly stable relative to their cis-2-isomers. Likewise, for nc 9, the trans-3-alkenes would be more stable than the trans-2-alkenes48. Wiberg and coworkers30 attributed the greater stability of liquid trans-2-hexene and trans-2-heptene relative to their 3-ene isomers to the electronic effect of the methyl group and noted that the isomerization enthalpy was slightly less negative for the C7H14 isomers than for the C6H12 isomers. Now that there are data for the C8H16 compounds, we observe continuous attenuation of Hisom for the trans-3- to trans-2-alkenes with increasing chain
length (C6 C8: 1.7, 0.7, 0.3 kJ mol 1). Qualitatively, the diminution of Hisom for the cis-3- to cis-2-alkene transformation mimics that for the trans isomers except for
the reversed stability order for C8H16 and presumably longer-chain cis-alkenes (C6 C8:1.2, 0.5, C1.6 kJ mol 1). Within the n-octene series, the trans double-bond migration enthalpy from C2 to C3 is C0.3 and from C3 to C4 it is 0.8 kJ mol 1. The corresponding cis migration enthalpies are 1.6 and C0.4 kJ mol 1. Overall, each of the 4-octenes is more stable than the corresponding 2-octene. Returning to the subject of the anomalous 2-butene isomerizations, it is tempting to ascribe the seeming additional stability of the 2-butenes to the presence of a second methyl group. That is, two methyl groups attached to the double bond is especially stabilizing, one methyl group on a relatively short chain is somewhat stabilizing and one methyl group on larger alkenes is either destabilizing or ineffective at off-setting some other effect. However, we acknowledge that this is only describing the phenomenon, not explaining it.
There are four pairs of 1- and 2-alkenes with branching in a substituent group for which we can examine double bond migration enthalpies49: 4-methyl-1- and 4-methyl-2-pentene; 5-methyl-1- and 5-methyl-2-pentene; 4-methyl-1- and 4-methyl-2-hexene; and 4,4- dimethyl-1- and 4,4-dimethyl-2-pentene. Each of the 2-enes exists in cis and trans forms. The first two pairs, which are homologous, have C1 to trans-C2 isomerization enthalpies of ca 13 (for two measurements of trans-4-methyl-2-pentene) and 14.4 kJ mol 1, and C1 to cis-C2 isomerization enthalpies of 9.6 and 10.4 kJ mol 1. The third pair, not homologous with any other, has a C1 to trans-C2 isomerization enthalpy of11.5 and a C1 to cis-C2 of isomerization enthalpy of 7.1 kJ mol 1. The last pair shows a cis repulsion steric effect in the isomerization enthalpies: from C1 to trans- C2 D 10.8 and from C1 to cis-C2 D C5.3 kJ mol 1. Not much can said about the trends in the homologous series to which these compounds belong, but we can generalize concerning isomerization of the C7H14 compounds: the isomerization enthalpy order for
H2CDCH CH2 R ! trans-CH3 CHDCH R is R D iso-Bu > sec-Bu, n-Bu > t-Bu. The somewhat odd substituent effect order is due to the stability orders of the two
alkene sets. For the 1-alkenes the order is n-Bu < iso-Bu < sec-Bu < t-Bu and for the trans-2-alkenes the order is n-Bu < sec-Bu < iso-Bu < t-Bu. However, the accuracy of the enthalpy of formation of 5-methyl-1-hexene was questioned in the previous section.
Comparing the effect of the double-bond migration within the identical -frameworks of isomeric isoalkyl-1-alkenes and the 2-methyl-1-alkenes, we find that the individual enthalpies of isomerization for C6 C8 are: 10.4, 10.9 and 11.3 kJ mol 1. These monoto disubstituted isomerization enthalpies are very similar to those for isomerization of the 1-n-alkenes to the trans-2-n-alkenes, homologous series which also possess a common structural skeleton.
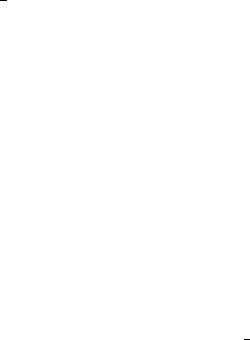
11. Thermochemistry of olefins, carbonyl compounds and imines |
555 |
2. Cis trans isomerization
The cis-to-trans isomerization enthalpies can be summarized with the understanding that the average given for a homologous series is the middle of a range of decreasing values and the standard deviation of the mean is an indication of the magnitude of the range of values: 2-n-alkenes ( 4.3 š 0.3); 3-n-alkenes ( 3.2 š 1.1); 4-octene ( 3.2). For the branched 2-alkenes discussed above (except for the 4,4-dimethyl-2- pentenes) the average cis-to-trans enthalpy of isomerization is 3.9 š 0.5 kJ mol 1. Thus there does not seem to be any discernible steric destabilization due to branching unless the substituent is tertiary, in which case for the 4,4-dimethyl-2-pentenes,Hisom D 16.1 kJ mol 1. The cis-to-trans (Z-to-E) isomerization enthalpies for three trisubstituted pairs of alkenes are less negative than or about equal to 1 kJ mol 1. Using averages of the several measurements for the 3-methyl-2-pentenes16,26,32 results in
Hisom D 1.0 kJ mol 1. Relying on enthalpies of hydrogenation from Reference 28 for the 3-methyl-3-hexenes and the 3,4-dimethyl-2-pentenes yields enthalpies of isomerization
of 1.2 and 0.3 kJ mol 1, respectively.
3. Isomerization of the sigma framework of substituted alkenes
Several alkene isomers vary structurally in the position of a methyl branch on their parent 1-alkene chain. Generally, moving the methyl group from C3 to positions further from the double bond results in an exothermic enthalpy of isomerization. That is, the isoalkyl-1-alkenes are the most stable isomers and the 3-methyl-1-alkenes are (presumably) the least stable. Because of the problematic 5-methyl-1-hexene data and the lack of data for 3-methyl-1-heptene, nothing more quantitative can be said other than each methyl re-positioning down the chain results in about 1 2 kJ mol 1 stabilization. A similar change in branching position from 3-methyl-n-alkanes to 2-methyl-n-alkanes releases about 3 kJ mol 1.
The two main categories of trisubstituted alkenes which allow us to compare the effect of redistributing methyl substituent groups are the 1,1-dimethyl-2-alkyl ethenes, (CH3)2CDCHR, and the E- and Z-1,2-dimethyl-2-alkyl ethenes, CH3CHDC(CH3)R. The former category includes 2-methyl-2-pentene, 2-methyl-2-hexene and 2,4-dimethyl-2- pentene. The latter category includes 3-methyl-2-pentene, 3-methyl-2-hexene (Z only) and 3,4-dimethyl-2-pentene. Using only the enthalpies of formation derived from enthalpies of hydrogenation26,28, in all cases the 1,1-dimethyl-2-alkyl ethenes are more stable50. The mean enthalpy of isomerization from the E isomers is 1.3 š 0.1 kJ mol 1 and
the mean enthalpy of isomerization from the Z isomers is |
|
1.8 |
š |
0.1 kJ mol 1 |
. Thus, |
||
two methyl groups on the same sp |
2 |
|
|
|
|
||
|
-carbon are more stabilizing than two methyl |
||||||
groups on adjacent sp2-carbons, at |
least for alkenes of |
nc 7. Because |
the Z- |
isomer enthalpies of isomerization are almost identical, there does not seem to be any additional steric effect due to the isopropyl group. Most interesting is to compare these results with the enthalpies of isomerization of E- and Z-3-methyl-3-hexene to 3-ethyl- 2-pentene: 0.9 and 2.1 kJ mol 1, respectively. The 1,1-diethyl ethene is more stable than the 1,2-diethyl ethene. From these limited examples, our empirical observation is that the more stable isomer is the one with two identical groups on the same sp2- carbon.
A related isomerization is that of the trans-1,2- to 1,1-disubstituted alkenes. The only series for which there is sufficient data are for the methyl, n-alkyl substituents. The slope of equation 11 is 1.01 and so the enthalpies of isomerization are nearly constant, although there is some scatter in the data. The point representing the C5 isomers is deviant in the plot, again suggestive of the unreliability of the enthalpy of formation of
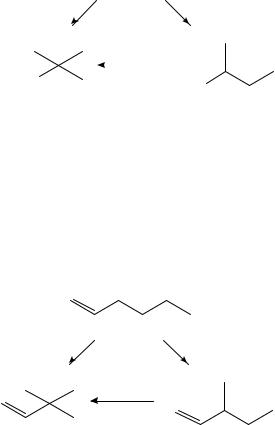
556 Suzanne W. Slayden and Joel F. Liebman
2-methyl-1-butene. Without using that data, the 2-methyl-1-alkenes are more stable by6.1 š 0.8 kJ mol 1.
Finally, we will explore the gaseous enthalpies of isomerization of small alkyl groups substituted in 1-alkenes, cis- and trans-2-alkenes, and 2-methyl-2-alkenes which may provide insight into the electronic and steric effects in substituted alkenes. The results cannot be extended reliably to larger alkyl groups because the isomeric compounds belong to different homologous series which very likely have non-identical slopes as determined from equation 4a and thus have non-constant enthalpies of isomerization. Nevertheless, the data are useful for estimating isomerization enthalpies of small alkyl groups on more complicated alkene parent compounds. The saturated alkanes are a convenient starting point for making comparisons, and the exothermic enthalpies of isomerization for the n-, sec- and tert-butyl ethanes16 are shown in Scheme 1. Not shown is the n-primary to secondary isomerization of n-pentane to 2-methylbutane (the propyl-substituted ethanes):6.8 š 1.3 kJ mol 1.
CH3 CH2
−19.0 ± 1.3 −5.0 ± 1.3
|
− 14.0 ± 1.4 |
|
CH3 CH2 |
|
CH3 CH2 |
|
|
SCHEME 1
The effect on the enthalpies of isomerization upon dehydrogenating the substituted ethanes to the corresponding 1-alkenes is different for the primary-to-secondary (more negative) and the secondary-to-tertiary (less negative) rearrangements, although the overall primary-to-tertiary isomerization is the same (Scheme 2). For comparison, the enthalpy of isomerization of 1-pentene to 3-methyl-1-butene (the propyl-substituted ethenes)
is 6.2 š 0.9 kJ mol 1. When the butyl |
groups are substituted on a |
double bond |
trans to a methyl group, the enthalpies of |
isomerization are comparable |
to or slightly |
smaller than when the methyl group is absent (Scheme 3). There are two available enthalpies of formation for trans-4,4-dimethyl-2-pentene which are just within each other’s error bars21,28. Having no way to decide between them, the mean value was used. For comparison with the values shown in Scheme 3 are those for isomerization of
−18.8 ± 2.1 |
−7.9 ± 2.0 |
−10.9 ± 2.3
SCHEME 2