

11. Thermochemistry of olefins, carbonyl compounds and imines |
567 |
R1
(CH2 )n-2
R2
(24)
Using the enthalpy of formation for tetramethylethylene and that of the product (Z)-2- butenes from Pedley, we find reaction 15 to be endothermic by 3.6 š 2.1 kJ mol 1, a value comfortably close to the anticipated thermoneutrality.
Relevant examples of 1,2-dialkylcycloalkenes with which to test our conjecture about reaction 12 are disappointingly few. The simplest example of this class of compounds
is 1,2-dimethylcyclopropene (24, n D 3, R1 D R2 D thermochemical data are available. Using the derived
Me), and indeed, the requisite enthalpy of formation of 1,2-
dimethylcyclopropene from Reference 56, we find this reaction to be 32 kJ mol 1 endothermic. A posteriori , we are not surprised that this reaction is endothermic. After all, if it is part of the ‘folklore’ of cyclopropanes that they are said to have olefinic character, then cyclopropenes are also said to have acetylenic character. Indeed, the related transalkylation reaction involving acetylenes
MeC CMe C C2H4 ! HC CH C Z-MeCHDCHMe |
16 |
is endothermic by 23 kJ mol 1. Admittedly, we would have thought that this acetylene reaction 16 would be more endothermic than reaction 12 for 1,2-dimethylcyclopropene because, for the former, the ring size is smaller (cyclopropene vs ‘cycloethene’57) and there is greater s-ness in the C Me bond being broken. This conceptual discrepancy between the endothermicities for cyclopropene and acetylene corroborates the suggestion in Reference 56 that the enthalpy of formation of 1,2-dimethylcyclopropene is suspect. While this last enthalpy of formation was derived using the enthalpy of hydrogenation measurements in the problematic polar solvent AcOH, the discrepancy is still disappointingly large. Regrettably, this is the sole thermochemical datum for any 1,2-dialkylcyclopropene, and indeed we admit now that this is one of the very few enthalpy-of-formation data known to the authors for any 1,2-dialkylcycloalkene!
Another sole thermochemically useful compound is the n D 4 case 1,2-dimethyl- cyclobutene, for which we must also use problematic data derived from measurements of hydrogenation enthalpies in AcOH. We also acknowledge complications generally found in the early measurements of the enthalpies of hydrogenation of tetrasubstituted olefins73. There is also uncertainty in the stereochemistry of the resulting 1,2-dimethylcycloalkane74. We are optimistic, however, that many of the above-enunciated problems with enthalpies of hydrogenation should cancel in the energetics of the following transmethylation reaction:
cycloCH2 1 MeCDCMe C cycloCH2 2 HCDCH ! cycloCH2 2 MeCDCMe
C cycloCH2 1 HCDCH (17)
More precisely, the sum of enthalpies of hydrogenation of the species on the left should equal the sum for the right. In fact, the right-hand sum is ca 25 kJ mol 1 more positive (less negative) than that on the left. This result is approaching plausiblity. Comparing established substituent effects in species with 3- vs 4-membered rings (e.g. Liebman1) and making simple assumptions about molecular geometries, we conclude that methyls
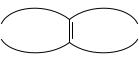
568 |
Suzanne W. Slayden and Joel F. Liebman |
on the cyclopropene ring on the left should result in more electronic stabilization via substituent-ring interaction and less steric destabilization via inter-substituent repulsion than methyls on the cyclobutene on the right.
We now turn to the n D 5 case, 1,2-dimethylcyclopentene, for which there are literature enthalpy-of-hydrogenation measurements in an innocuous solvent coupled with a stereochemical analysis of the products67. Of all the dialkylcycloalkenes that are mentioned in this section, it is with dimethylcyclopentene that equation 14, now with n D 5 and R D Me, is most expected to be essentially thermoneutral. We find the reaction is endothermic by ca 11 kJ mol 1 and fail to understand the discrepancy75.
B. Bicyclo[m.n.0]alkenes and Related Species
Bicyclo[m.n.0]alkenes (25: m, n) with the double bond linking the bridgehead carbons are another class of relevant compounds. Enthalpy-of-formation data are available for the m D n D 1 case through analysis of gas-phase acidity and electron-affinity measurements76, and for m D 2, n D 2, 3 and 4 from hydrogenation data54 from reaction in a thermochemically innocuous hydrocarbon solvent. Parallelling the earlier transalkylation reactions (equation 14), perhaps the simplest comparison we can make is to use the enthalpy of the reaction of the bicyclic species with ethylene to form two monocyclic ones.
25 m, n C C2H4 ! cycloCH2 m CHDCH C cycloCH2 n CHDCH 18
(CH2 )m |
(CH2 )n |
(25)
This reaction enthalpy probes the superstrain associated with the two, trigonal, bridgehead carbons beyond that found in the individual rings. As may simply be concluded by looking at the molecular structure, the m D n D 1 species is clearly highly strained (quantum chemical calculations show the additional structural feature of non-planarity77). Experimental enthalpy-of-formation measurements of bicyclobutene show this compound to be accompanied by an exothermicity of reaction 18 of 42 š 42 kJ mol 1.
For the m D 2 and n D 2, 3 and 4 cases, equation 18 is found to be exothermic by 42, 34 and 4 kJ mol 1, respectively. The large error bars for the energetics of the m D n D 1 case precludes comparison with these exothermicities for the ‘larger’ species and so it is not obvious that it is ‘clearly highly strained’ beyond the presence of two cyclopropene rings. The data are consistent with this m D n D 1 species having much more superstrain than any of the others, and the m D n D 1 species being somewhat less superstrained than the m D 2, n D 4 case. That reaction 18 for this last species is so close to thermoneutral suggests there is almost no additional strain or superstrain associated with ring fusion, for the corresponding acyclic reaction is the effectively thermoneutral equation 14. We can test this assumption of the absence of additional strain by considering species with more rings and thereby modulate the effective ring sizes connoted by the numbers m and n. Bridging the 2 and 4 carbons of the m D 2, n D 3 species by an ethano link to form 26 (equivalently, bridging the 2 and 5 carbons of the m D 2, n D 4 species by a

11. Thermochemistry of olefins, carbonyl compounds and imines |
569 |
(26)
methano bridge) results in a norbornene derivative. This is expected to result in a species with superstrain intermediate to the m D 2, n D 2 and m D 2, n D 3 species78. Using equation 18 and the recommended norbornene enthalpy of formation from Rogers and coworkers60 results in an exothermicity of 45 kJ mol 1. While one should not conclude this norbornene derivative must be more strained than the m D 2, n D 2 compound, a high degree of strain is suggestive. Or one can join the 5 and 6 carbons of the ‘2’ bridge in the m D 2, n D 3 compound by a propano or trimethylene link. Taking the enthalpy of formation of the resultant bicyclo[3.2.0]hept-5-ene from Roth and coworkers54, an exothermicity of 33 kJ mol 1 suggests little change in strain by including this quite long and strainless link. This result is not particularly surprising.
We close this section with a brief discussion of how our analysis can be used to evaluate other data from the experimental literature. Consider compound 25 with m D n D 4. Based on the above reasoning, we expect reaction 18 to be essentially thermoneutral and so its gas-phase enthalpy is expected to be ca 65 kJ mol 1. Let us assume that it is the same as the octahydronaphthalene cited by both Kharasch and Stull, Westrum and Sinke. If we accept the enthalpy-of-combustion data value cited in the former archive, we derive an enthalpy of formation of the liquid as 106 kJ mol 1. From the latter archive, the corresponding enthalpy-of-formation value of 145 kJ mol 1 is found. Using our standard enthalpy of vapourization estimation protocol, we obtain a phasechange enthalpy of ca 50 kJ mol 1 and so the two ‘competing’ gas-phase enthalpies of formation are 56 and 95 kJ mol 1. The former value is more tenable, although the 9 kJ mol 1 difference between theory and experiment is larger than we would have perhaps liked79.
C. Polyhedrenes
Admittedly, not all polycyclic tetrasubstituted olefins can be so simply constructed or described as bicyclo[m.n.0]alkenes. Gas-phase ion molecule experiments (combined with some judicious but non-experimentally measured numbers) allowed the derivation of80 the formal enthalpy of hydrogenation of cubene (27a), i.e. the exothermicity of reaction 19 to form the saturated cubane (27b).
cubene(g) 27a C H2(g) ! cubane(g) [ CH 8, 27b] |
19 |
Accepting the archival enthalpy of formation of gaseous cubane81, we thus deduce the enthalpy of formation of cubene80 to be 1000š 17 kJ mol 1. It is hard to have an intuitive feel for such a large, positive enthalpy of formation. One possible probe of the energetics of cubene is the exothermicity of reaction 20
cubene C C2H4 ! tetracyclo[4.4.0.02,5.07,10]deca-3, 8-diene 28 |
20 |
which we recognize as an ‘intramolecular’ counterpart of equation 18. We would be disappointed if this last diene with all of its fused cyclobutane rings lacked some form of homoconjugative stabilization and/or destabilizing superstrain. As such, even were its
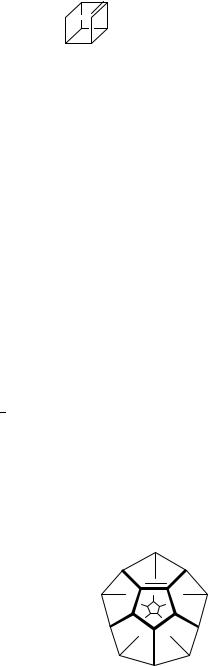
570 |
Suzanne W. Slayden and Joel F. Liebman |
|||||||||
|
|
|
|
|
|
|
|
|
|
|
|
|
|
|
|
|
|
|
|
|
|
|
|
|
|
|
|
|
|
|
|
|
|
|
|
|
|
|
|
|
|
|
|
(27)(28)
enthalpy of formation known, somehow the comparison of the energetics of the polycyclic reaction 20 and of 18 for m D n D 2 would still be suspect as a reference energy calculation82. Alternatively, cubene and its fewer-ring counterpart, species 25 (with m D n D 2), are naturally contrasted by looking at the energetics of reaction 21.
27a C bicyclo[2.2.0]hexane ! 27b C 25 m D n D 2 |
21 |
The desired number may be recognized as the difference of the enthalpies of hydrogenation of cubene and bicyclo[2.2.0]hex-1-ene and found to be ca 50 š 17 kJ mol 1 endothermic. In that cubene is more rigid than the bicyclohexene, it is surprising that the hydrogenation enthalpy is smaller for the former than the larger. Alternatively, consider reaction 22.
27a C Me2CHCHMe2 ! 27b C Me2CDCMe2 |
22 |
We may translate its energetics into the difference of the enthalpies of hydrogenation of cubene and the acyclic tetramethylethylene16. We deduce reaction 22 is some 266 š 17 kJ mol 1 exothermic. This number is not particularly useful in that we have already seen there are no obvious patterns in the hydrogenation enthalpies of monocyclic alkenes. As such, why should there be patterns in the energetics of polycyclic olefins such as the various species 25. A forteriori, expecting sense from the energetics of cubene could be viewed as at best premature.
Sometimes a polyhedrene is so complex that comparison is all but impossible with any but the simplest paradigm. An example is dodecahedrene (29). From a different type of gas-phase ion molecule reaction than used for cubene, it was deduced83 that the enthalpy of hydrogenation of dodecahedrene to form dodecahedrane is some 157 š 15 kJ mol 1 more exothermic than that of tetramethylethylene. We lack the standard gas-phase enthalpy of formation of this dodecahedrane, although it was recently derived from knowledge of a relatively simple derivative84 to be ca 94 kJ mol 1. But this is not the obstacle. We do not know how to write a meaningful reaction analogous to equation 20: the product with the resultant two CHDCH groups finds them too close85. The simpler comparison of dodecahedrene with species 25 (m D n D 3) is thwarted by the absence of thermochemical information on this last, quite normal-looking, species.
(29)
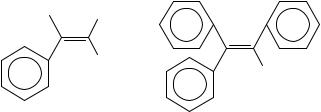
11. Thermochemistry of olefins, carbonyl compounds and imines |
571 |
VII. OLEFINS WITH AROMATIC SUBSTITUENTS
A. Phenylated Ethylenes
The compounds discussed in this section are all of the generic type ‘Phq H4 q C2’ and their substituted derivatives. These species may be considered to be on the boundary of acyclic and cyclic because the ‘relevant’ (i.e. olefinic) double bond will almost never be found in a ring, but there are ‘irrelevant’ (i.e. benzenoid) double bonds that are always found in rings. We also recognize these compounds as being on the borderline of compounds with a single relevant double bond and those with many because of the wellestablished near-constancy of the difference of enthalpies of formation of corresponding (gas-phase) phenyl and vinyl groups86,87. As such, these species may be said to thermochemically mimic di-, triand even more extended polyenes. Let us commence with the three simplest, and best characterized, members of this series of phenylated ethylenes: with 0, 1 and 2 phenyl groups, we have ethylene itself, styrene (30a), and (because it is the least strained) (E)-stilbene (30b). The relevant gas-phase enthalpies of formation are 52.5 š 0.4, 147.9 š 1.5 and 236.1 š 1.3 kJ mol 1. These numbers correspond to sequential enthalpies of phenylation of 95.4 š 1.6 and 88.2 š 2.0 kJ mol 1. It is interesting to note that the second phenyl group is slightly more stabilizing than the first, i.e. the increase of enthalpy of formation upon sequential phenylation is less going from one phenyl to two than from none to one. This is in contrast to the case of the first and second methylation enthalpies of ethylene [to form propene and (E)-2-butene, respectively] which are identical to within experimental error. The enthalpy of formation of the gem-disubstituted 1,1-diphenylethylene (30c) is some 10 kJ mol 1 more positive than that of its (E)-1,2- isomer, while the cis-disubstituted (Z)-1,2-diphenylethylene (30d) is some 20 kJ mol 1 more positive than that of the (E)-isomer88. By contrast, (E)-2-butene is less stable than 2-methylpropene by ca 5 kJ mol 1 but it is 4 kJ mol 1 more stable than (Z)-2-butene. This is not particularly surprising: phenyl groups are larger than methyl groups and steric inhibition of phenyl/ethylene resonance is to be expected when two phenyls are on the same carbon atom89.
R1 R2
R3
(30a) R1 = R2 = R3 = H
(30b) R1 = R3 = H R2 = Ph (30c) R1 = Ph R2 = R3 = H (30d) R1 = R2 = H R3 = Ph
R
(31a) R = H
(31b) R = Ph
Such destabilization is therefore to be expected for the triand tetraphenylethylenes (31a and 31b). The enthalpy of formation is found only for the solid-phase species. The first step is to estimate their enthalpies of sublimation. Let us apply an equation explicitly designed for the study of planar aromatic hydrocarbons90, where nc is the total number of carbons,
Hs D 6.1nc C 2.5 |
23 |
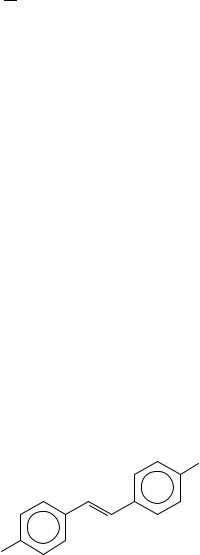
572 |
Suzanne W. Slayden and Joel F. Liebman |
Summing the experimentally determined solid-phase enthalpies of formation of the olefin and the sublimation enthalpies predicted by equation 23 results in the desired gasphase quantities of 358 and 473 kJ mol 1 for the enthalpies of formation of triand tetraphenylethylene. Combined with the above enthalpies of formation of ethylene, styrene and (E)-stilbene, our new numbers allow us to generate a reasonably straight line as a function of the number of phenyl groups:
Hf° ‘Phq H4 qC2’ D 44.9 C 104q n D 5, r D 0.9974 |
24 |
Leaving out (E)-stilbene after all, why should this species be the ‘representative’ of
the n D 2 compounds and not either 1,1-diphenylethylene or (Z)-stilbene? |
|
we find the |
|
||
even more precise equation |
|
|
Hf° ‘Phq H4 qC2’ D 50.1 C 102q n D 4, r D 0.9997 |
25 |
from the data for the series ethylene, styrene, triphenylethylene and tetraphenylethylene. From equation 25, we find the ‘predicted’ value of the enthalpy of formation of gaseous diphenylethylene, 254 kJ mol 1, is plausible: it is approximately the average of the reported values for the 1,1-, (Z)-1,2- and (E)-1,2-species.
B. ‘Tolylated’ Ethylenes
The compounds highlighted in this section are all of the generic type ‘Tolq H4 q C2’. The most natural comparison is with the corresponding phenylated olefins, ‘Phq H4 q C2’, with which we define a difference quantity υ26(Ph, Tol) as
υ26 q, Ph, Tol 1/qf[ H°f Phq H4 qC2, s ] [ H°f Tolq H4 q C2 , s ]g 26
For the p-tolyl species, we have archival enthalpies of formation for q D 2 [however, for only the (E)-1,2-isomer, 32, are there relevant data91], q D 3 and q D 4. These difference quantities equal 39.5 š 1.7, 37.4 š 2.1 and 35.0 š 2.2 kJ mol 1 and are comparable to the difference of 34.2 kJ mol 1, found for the not quite ‘proper’ q D 1 case of solid benzene and solid toluene92 used in lieu of the desired enthalpy-of-formation data for solid p-methylstyrene to accompany solid styrene. For the o-tolyl species, we have archival enthalpies of formation for q D 2 [again, only (E)-1,2- is applicable here] and q D 3, resulting in difference quantities of 31.0 š 1.7 and 36.1 š 2.1 kJ mol 1 and again comparable to the solid benzene/solid toluene difference. Let us assume a consensus value of υ26(q, Ph, Tol) of 36 kJ mol 1. Working backwards from the cited enthalpies of formation of the solid 1,1-bis(p-tolyl) and 1,1-bis(o-tolyl)ethylenes results in an enthalpy of formation of solid 1,1-diphenylethylene of 158 and 161 kJ mol 1, respectively. Combining the archival enthalpy of formation of gaseous 1,1-diphenylethylene and a suggested enthalpy of sublimation from equation 23 results in the nearly identical prediction of 158 kJ mol 1 for this quantity. There appears to be consistency93.
(32)
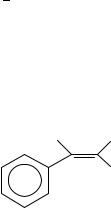
11. Thermochemistry of olefins, carbonyl compounds and imines |
573 |
C. Alkylated Styrenes
We start with methylated styrenes. The enthalpies of formation of gaseous ˛-, (E)-ˇ- and (Z)-ˇ-monomethylstyrenes (33a 33c) and of ˇ,ˇ-dimethylstyrene (33d) have been ascertained by a combination of hydrogenation, rearrangement and combustion calorimetry94 to be 115.3š 2.3, 112š 2, 123.0š 1.3 and 86.0š 1.2 kJ mol 1, respectively. Interestingly, the values for the three monomethylstyrenes are almost identical to what is found by averaging the enthalpies of the correspondingly substituted dimethyl and diphenylethylenes. That is, the following reactions are nearly thermoneutral:
Ph2H2C2 C Me2H2C2 ! 2PhMeH2C2 |
27 |
R1 R2
R3
(33a) R1 = Me, R2 = R3 = H
(33b) R1 = R3 = H, R2 = Me
(33c) R1 = R2 = H, R3 = Me
(33d) R1 = H, R2 = R3 = Me
Relatedly, the enthalpy of formation of the dimethylstyrene is very nearly equal to that predicted assuming thermoneutrality for the reaction
E -PhCHDCHMe C Z -PhCHDCHMe ! PhCHDCH2 C PhCHDCMe2 28
Owing to the large size of t-butyl groups relative to those of methyl, we do not expect thermoneutrality for the reaction
E -PhCHDCHBut C Z -PhCHDCHMe ! Z -PhCHDCHBut
C E -PhCHDCHMe (29)
This would only be achieved if the (Z)/(E)-enthalpy of formation difference of ˇ- methylstyrene and ˇ-t-butylstyrene were the same. For the former, we find the desired difference to be ca 13 š 2 kJ mol 1, while for the latter the difference is found41 to be 33.2 š 9.3 kJ mol 1. Consider reaction 30 that interrelates the above two ˇ-alkylated styrenes:
E -PhCHDCHBut l C MeCHDCH2 g ! E -PhCHDCHMe g |
|
C t BuCHDCH2 l |
(30) |
If all four olefins were in the same phase then this reaction would be expected to be thermoneutral. As it is, the right-hand side has six more carbons in the gaseous phase than does the left. We thus expect the right-hand side to be automatically more positive than the left by ca 28 kJ mol 1, the enthalpy-of-vaporization contribution of the six carbon atoms according to the estimation approach in Reference 3. In fact, making use of the data in Reference 41 for the ˇ-t-butylstyrene, this reaction is seen to be exothermic by 37 kJ mol 1. The 9 kJ mol 1 discrepancy is surprising and disappointing.
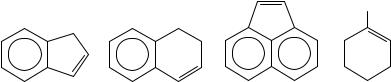
574 |
Suzanne W. Slayden and Joel F. Liebman |
Kharasch, and Stull, Westrum and Sinke contain references to some other alkylated styrenes which we hesitate to use in the current chapter: it is well-established that styrene and many of its derivatives readily oligomerize and so we expect purity problems to plague earlier studies. Yet we briefly proceed here. For example, consider the formal reaction
E-PhCHDCHEt Ł C PhPr Ł ! E-PhCHDCHMe Ł C PhBu Ł |
31 |
If * corresponds to either the gas or pure liquid for each species, we might expect this reaction to be essentially thermoneutral. If:
(a)all of the enthalpies of formation are correct,
(b)our protocol for estimating enthalpies of vapourization is accurate and
(c)the assumption that numerically, enthalpies of hydrogenation of hydrocarbons are independent of the choice of gas, pure liquid, or in dilute hydrocarbon solution is valid, then the following reaction 32 should also be thermoneutral:
E-PhCHDCHEt l C PhPr g ! E-PhCHDCHMe g C PhBu l 32
Using the earlier value ignored by Pedley for the enthalpy of formation of 1-phenyl-1- butene but taken from our earlier archives, the very recent value for 1-phenylpropene94 and the archival values for propyl and butylbenzene, we find this reaction is thermoneutral to within 2 kJ mol 1!
D. ‘Cyclic Styrenes’
By our definition of olefins with aromatic substituents, it appears plausible to include indene (34), 1,2-dihydronaphthalene (35) and acenaphthylene (36) in this category. The natural comparison is with the saturated indane, tetralin, and acenaphthene for which the enthalpies of hydrogenation of our putative styrene derivatives are 99.1 š 2.3 (l) and 102.7 š 2.7 (g); 110.7 š 2.3 (l) kJ mol 1; 116.4 š 5.3 (s) and 103.7 š 5.6 (g), respectively. By comparison, making use of the above enthalpy of formation of gaseous (E)-1-phenylpropene, we derive its enthalpy of hydrogenation95 to be 104š 3 kJ mol 1. It would thus appear that indene, 1,2-dihydronaphthalene96 and acenaphthylene are logically, if roughly, considered ‘cyclic styrenes’97.
Ph
(34) |
(35) |
(36) |
(37) |
By our definition of ‘cyclic styrenes’, it is also logical to include 1-phenylcyclohexene (37) for which an enthalpy of formation has been measured and is given in our archive. In addition, its (Z)-/(E)-isomerization enthalpy is also available from experiment (cf Reference 55). Indeed, it may be argued that discussion of these species belongs in the current section of the chapter. Perhaps, but now we note that we do not consider the reported enthalpy of formation of the conventional isomer to be correct. From the proferred liquid-phase value of the enthalpy of formation of the olefin of interest, 16.8 š 6.7 kJ mol 1, and of the related saturated species, phenylcyclohexane,

11. Thermochemistry of olefins, carbonyl compounds and imines |
575 |
76.6 š 1.4 kJ mol 1, we deduce an enthalpy of hydrogenation of ca 60 kJ mol 1. This value is totally incompatible with any other styrene derivative we know of, e.g. the various other unsaturated hydrocarbons enunciated earlier in this section99. Likewise, additionally using the enthalpies of formation of liquid 1-phenylcyclohexene and biphenyl results in an enthalpy of dehydrogenation of 132 kJ mol 1, while the parent cyclohexene and its 1-methyl and 1-ethyl derivatives and their benzene analogs have enthalpies of dehydrogenation of ca 93 kJ mol 1. The origin of the discrepancy remains unknown99.
VIII. ACYCLIC ALDEHYDES AND KETONES
A. Assessment and Correlation of Data
All the enthalpies of formation for acyclic aliphatic aldehydes and ketones which are discussed in this section are tabulated by Pedley and coworkers16. The number of these carbonyl compounds whose enthalpies of formation have been measured are few compared to the abundant data available for alkenes and so there are fewer decisions to make on which data to include. However, we then lack the means to make the comprehensive analyses we would prefer. The demonstrated linearity34 of the enthalpies of formation of the members of a homologous series vs the number of carbon atoms, nc, in the molecules provides an excellent visual method for determining the quality of the data; any enthalpy of formation which deviates significantly from linearity is of questionable reliability. The linear relationship can be expressed as in equation 4 and the results of the regression analyses of this equation for aldehydes and ketones appear in Table 4.
When the enthalpies of formation of the n-aldehydes are plotted versus nc, the pentanal point is a clear outlier in both the liquid and gaseous states. Interpolating a value from the constants given in Table 4 yields Hf(g) D 224.5 š 0.9 kJ mol 1 and Hf(l) D263.1 š 0.1 kJ mol 1. The methyl derivative, acetaldehyde, does not appear to deviate44 at all from the straight line established by the nc D 3, 4 and 7 members and so it is included in the regression analysis.
As for other disubstituted functional groups such as ethers4 and sulphides3, it is necessary to divide the non-branched aliphatic ketones into two categories: methyl n-alkyl ketones and di-n-alkyl ketones. The latter category includes examples of 3-, 5- and 6- alkanones belonging to two different homologous series, the ethyl alkyl ketones and the symmetrical dialkyl ketones; 3-pentanone is common to both series. In the methyl n- alkyl ketone series, the enthalpies of formation of both liquid and gaseous 2-nonanone appear about 2 kJ mol 1 too positive compared to the otherwise straight line incorporating the other data points. Neither the gaseous nor liquid enthalpy of formation of acetone, the methyl derivative, deviates from its respective line. The enthalpies of formation of
TABLE 4. Results from the |
linear regression analysis of equation 4 for aldehydes |
and ketonesa |
||
(kJ mol 1) |
|
|
|
|
|
˛l |
ˇl |
˛g |
ˇg |
|
|
|
|
|
n-R(CDO)H |
23.77 š 0.13 |
144.2 š 0.3 |
19.45 š 0.07 |
127.2 š 0.2 |
[R D Me, Et, Pr, Hex] |
25.09 š 0.18 |
172.5 š 1.0 |
20.67 š 0.10 |
155.6 š 0.5 |
n-R(C=O)Me |
||||
[R D Me, Et, Pr, Bu, Hept, Dec] |
169.9 š 0.7 |
21.66 š 0.10 |
149.7 š 0.7 |
|
n-R2(CDO) |
25.33 š 0.10 |
|||
[R D Et, Et; Bu, Bu; Pen, Pen] |
|
|
|
|
a In the least-squares analyses of equation 4, the individual enthalpies were weighted inversely as the squares of the experimental uncertainty intervals. The correlation coefficient for each analysis is 0.999.

576 |
Suzanne W. Slayden and Joel F. Liebman |
gaseous and liquid acetone do deviate from linearity when considered as members of the symmetrical di-n-alkyl ketone series, their measured enthalpies being more negative than those calculated by equation 4.
B. Enthalpies of Isomerization
1. Ketone isomerization
Both 2-pentanone and 2-hexanone are more stable than their 3-alkanone isomers by 1.1 and 1.5 kJ mol 1, respectively, in the gaseous state. Thus, the exothermic enthalpy of isomerization of ethyl to methyl ketones probably increases slightly with increasing nc, as deduced from this very limited data set. However, 5-nonanone is more stable by 4.2 kJ mol 1 than 2-nonanone (or by 2.2 kJ mol 1 using an estimated value for the 2-isomer). 6-Undecanone is 4.7 kJ mol 1 more stable than the estimated enthalpy of formation for the unmeasured 2-undecanone (g, 382.7 š 0.5). Because the slopes, ˛g, are quite different for the methyl n-alkyl ketones and the symmetrical di-n-alkyl ketones, we expect the stability difference to increase with nc. Both a graphical plot of the data and a calculation using the slope and intercept values in Table 4 show that the reversal in stability of the methyl n-alkyl ketones and the symmetrical di-n-alkyl ketones occurs at about C7 (g). Recall the change in stability order for the cis-2- and -3-n-alkenes at ca nc D 7 and for the trans-2- and -3-n-alkenes at a calculated nc D 9. In contrast, the slope for the methyl n-alkyl ethers is nearly parallel to that of the more stable di-n-alkyl ethers100,101.
2. Aldehyde/ketone isomerization
As a comparison of the enthalpies of formation of isomeric aldehydes and ketones shows, the disubstituted carbonyl compounds (ketones) are more stable than the monosubstituted carbonyl compounds (aldehydes), analogous to the stability order for the corresponding 1,1-disubstituted ethenes and the 1-n-alkenes. For the three aldehyde/methyl ketone isomeric pairs (nc D 3 5), initially it seems that the enthalpies of isomerization are fairly constant. However, if the interpolated value for pentanal is used, the trend is clearly that of more negative enthalpy of isomerization with increasing nc(g): 31.7, 33.9, 34.5 kJ mol 1. The non-constant enthalpies are expected because the slopes, ˛g, in Table 4 are quite different for aldehydes and ketones. The greater contribution to the isomerization enthalpy differences comes from the methyl ketone which is more stabilized than the aldehyde by an additional CH2 group. The enthalpies of isomerization of the corresponding alkenes are much less exothermic ( 6.1 š 0.8 kJ mol 1) than those of the carbonyl compounds.
3. Isomerization of substituent groups
Just as for the alkenes in a previous section, we would like to compare the enthalpies of isomerization of small alkyl substituents attached to the carbonyl functional group. The results are summarized in Table 5 for the isomerization reaction
R1 X R2 ! R3 X R4 |
33 |
We will also compare the carbonyl compounds with alkenes and with other oxygencontaining compounds102 to assess steric and electronic effects on the isomerizations.
The only available aldehyde data are for isomerization of butanal to 2-methylpropanal for which Hisom(g) D 11.0 š 1.7 kJ mol 1. The corresponding alkene isomerization enthalpy of 1-pentene to 3-methyl-1-butene is 6.2 š 0.9 kJ mol 1. Hydrogenating all