
Environmental Biotechnology - Theory and Application - G. M. Evans & J. C. Furlong
.pdf224 Environmental Biotechnology
Transgenic Plants
Currently, genetic engineering in agribiotechnology is focusing on genetic modifications to improve crop plants with respect to quality, nutritional value, and resistance to damage by pests and diseases. Other avenues under investigation aim to increase tolerance to extreme environmental conditions, to make plants better suited for their role in pollutant assimilation, degradation or dispersion by phytoremediation, or to modify plants to produce materials which lead to the reduction of environmental pollution. Crop quality improvements such as the control of fruit ripening (Grierson and Schuch 1993), an example of which is the oft quoted, Flavr-Savr tomato, and the production of cereals with improved nutritional value, are not addressed here because, although of great interest to the food industry, are of more peripheral relevance to environmental biotechnology. Many of the transgenic plants, examples of which are given later in this chapter, have been produced using the Ti plasmid transfer system of Agrobacterium tumefaciens and often, together with the 35S CaMV promoter. Both of these tools are described from a GE technique viewpoint in this chapter and from a biological standpoint in Chapter 10.
Transformation of plants
There are two practical problems associated with genetic engineering of plants which make them more difficult to manipulate than bacteria. Firstly they have rigid cell walls and secondly they lack the plasmids which simplify so much of genetic engineering in prokaryotes. The first problem is overcome by the use of specialised techniques for transformation, and the second by performing all the manipulations in bacteria and then transferring the final product into the plant. The DNA construct contains regions of DNA which are complementary to the plant DNA to enable the inserted piece to recombine into the plant genome.
The most popular method of transforming plants is by the Ti plasmid but there are at least two other methods also in use. The first is a direct method where DNA is affixed to microscopic bullets which are fired directly into plant tissue. An example of this technology is the introduction into sugarcane, of genes able to inactivate toxins produced by the bacterium, Xanthomonas albilineans, causing leaf scald disease (Zhang, Xu and Birch 1999). This method of biolistic bombardment, may increase in popularity in line with improvements to plastid transformation. It is now possible to produce fertile transgenics expressing foreign proteins in their edible fruit (Ruf et al. 2001).
The second is by protoplast fusion which is a process whereby the plant cell wall is removed leaving the cell surrounded only by the much more fragile membrane. This is made permeable to small fragments of DNA and then the cells allowed to recover and grow into plants. These methods can be unsuccessful due to difficulties in recovery of the cells from the rather traumatic treatments and also because the DNA introduced, has a tendency to be inserted randomly
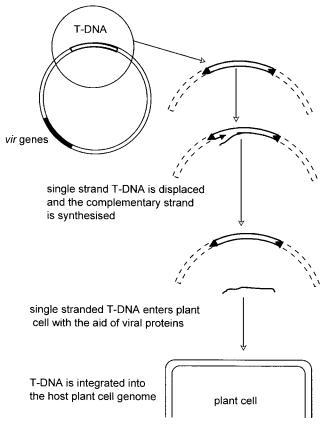
Genetic Manipulation 225
into the genome, rather than at a defined site. However, both methods enjoy the advantage that DNA enters the cell exactly as constructed and has not passed through an intermediate vector giving the opportunity for gene rearrangement.
Transformation by the Ti plasmid of Agrobacterium tumefaciens, shown diagrammatically in Figure 9.4, suffers from few disadvantages other than the limitation that it does not readily infect some cereal crops. This potential problem has been addressed by attempting to increase its host range (Godwin, Fordlloyd and Newbury 1992) which has met with success, leading to improved transformation procedures (Le et al. 2001). In essence, the wild-type plasmid contains genes which causes the transfer of a piece of DNA, ‘T-DNA’, into a plant cell. This piece is bordered by sequences of 24 base pairs in length which are repeats of each other. This structure is fairly common in DNA and is described as direct repeat. The T-DNA comprises genes which cause crown gall disease. These
Figure 9.4 Ti plasmid of Agrobacterium tumefaciens
226 Environmental Biotechnology
genes may be cut out and replaced by DNA containing the gene of choice to be introduced into the plant. There are many additional elements which may be included in the construct. For example, if the aim is to express the gene, it is preceded by a strong promoter, most commonly the ‘35S’ promoter of Cauliflower Mosaic Virus (CaMV).
In addition to the above, it is important to know if the ‘foreign’ gene is being expressed and so frequently a ‘reporter’ gene described in the section above is also included located close to the gene of interest. Recombination is not 100% efficient, and so a method of selection is required such that only plants containing the novel DNA grow. This is frequently a gene coding for resistance to weedkiller or antibiotic. On the grounds of size, this is usually introduced more successfully on a second Ti plasmid during a co-infection with Agrobacterium carrying the plasmid containing the gene of interest. The experiment can become somewhat complicated at this stage, as other selector genes are introduced into the plasmids to ensure that growth is only possible if all the desired elements are present in the plant cell. This can involve infection with two or three cultures of Agrobacterium each containing its own engineered Ti plasmid. A very detailed description of the Ti plasmid is published elsewhere (Hughes 1996).
Examples of developments in plant GE
The purpose of these examples is to illustrate the potential plant genetic engineering could bring to future practical applications in the field of environmental biotechnology. In some cases the intention is to reduce the amount of herbicide and pesticides, or other agricultural chemicals required to produce a given crop yield, in others it is to improve tolerance of harsh conditions or to protect the plants from attack thus reducing wastage. The intention is to note the technical details here, while the effects such developments may have on the environment as a whole, feature elsewhere throughout this book.
Broad range protection
A general strategy to protect plants from various viruses, fungi and oxidative damage by a range of agents, has been proposed using tobacco plants as a model. The transgenics express the iron-binding protein, ferritin, in their cells which appears to afford them far-ranging protection (Deak´ et al. 1999).
Resistance to herbicides
‘Glyphosate’, one of the most widely used herbicides, is an analogue of phosphoenol pyruvate and shows herbicidal activity because it inhibits the enzyme
5-enolpyruvylshikimate-3-phosphate synthase. The gene coding for this enzyme has been identified, isolated and inserted into a number of plants including
Genetic Manipulation 227
petunias. In this case, the gene was expressed behind a CaMV promoter and introduced using A. tumefaciens, leading to very high levels of enzyme expression. As a consequence, the recombinant plants showed significant resistance to the effects of glyphosate (Shah et al. 1986). Developments in this strategy include the formation of a chimaeric synthase enzyme, the analysis of which should lead to improved herbicide resistance in transgenic crops using this strategy (He 2001).
An alternative approach but still using A. tumefaciens has been to transfer the genes for mammalian cytochrome P450 monooxygenases, known to be involved in the detoxification (and activation) of many xenobiotics including pesticides, into tobacco plants. These transgenics displayed resistance to two herbicides, chlortoluron and chlorsulphuron (Yordanova, Gorinova and Atanassov 2001).
Improved resistance to pests
Plants have an inbuilt defence mechanism protecting them from attack by insects but the damage caused by the pests may still be sufficient to reduce the commercial potential of the crop. The usual procedure is to spray the crop with insecticides but in an effort to reduce the amount of chemical insecticides being used, plants are being engineered to have an increased self-defence against pests. Attack by insects not only causes damage to the plant but also provides a route for bacterial or fungal infection in addition to the role played in the spread of plant viruses. With a view to increasing resistance to sustained attack, the genes coding for the δ-endotoxin of the bacterium, Bacillus thuringiensis (Bt), described a little more fully in Chapter 10, have been transferred into plants. Examples are of synthetic B. thuringiensis δ-endotoxin genes transferred, in the first case, by A. tumefaciens into Chinese cabbage (Cho et al. 2001) and in the second, by biolistic bombardment into maize (Koziel et al. 1993). In both cases, the transgenic plants showed greatly improved resistance to pest infestation. There are, however, some problems with crop performance of some genetically engineered plants highlighted in Magg et al. (2001). Insects are able to develop resistance to Bt products which is a problem addressed by insertion of δ-endotoxin genes into the chloroplast genome rather than into that of the plant’s nucleus, with promising early results (Kota et al. 1999).
It may be recalled, that for each amino acid incorporated into a protein there is usually a choice of three or four codons all of which code for that same amino acid. Different organisms have distinct preferences for a particular codon, thus Bacillus thuringiensis tends to use codons richer in thymidine and adenine than the plant cells into which the gene is placed. There are also signals controlling the expression of these genes relevant to bacteria, rather than eukaryotes, which will not function very well, if at all, in the plant cell. For these reasons, expression may benefit from modification of the DNA sequence to compensate for these differences while maintaining the information and instructions. This may account in part for the very high levels of expression and stability of the Bt proteins whose genes have been introduced, by (biolistic) microbombardment, into chloroplasts
228 Environmental Biotechnology
(De Cosa et al. 2001) which, because of their prokaryotic ancestry, have ‘protein synthesising machinery’ more in keeping with prokaryotes than the eukaryotic cell in which they cohabit.
Attempts to improve virus resistance have led to the introduction, by A. tumefaciens, of the genes expressing antibodies to the coat protein of Tobacco Mosaic Virus (TMV). Expression of these in the plant led to complete immunity against TMV (Bajrovic et al. 2001).
Improved resistance to disease
Bacteria communicate with each other by way of small diffusible molecules such as the N-acylhomoserine lactones (AHLs) of Gram negative organisms. In this way, described as ‘quorum sensing’, they are able to detect when a critical minimum number of organisms is present, before reacting. These responses are diverse and include the exchange of plasmids and production of antibiotics and other biologically active molecules. Plants are susceptible to bacterial pathogens such as Erwinia carotovora, which produces enzymes capable of degrading its cell walls. The synthesis of these enzymes is under the control of AHLs and so they are made only once the appropriate threshold level of this chemical has been reached. The rationale behind using AHLs for plant protection is to make transgenic plants, tobacco in this case, which express this signal themselves. The consequent high level of AHL presented to the pathogenic bacteria, wrongly indicates a very high number of similar organisms in the vicinity, and triggers the bacteria into responding. As a consequence, they produce enzymes able to degrade the plant cell walls and continue infection. The plant will mount its normal response to invasion but on a far greater scale than necessary to destroy the few bacteria actually causing the infection, thus improving the plant’s resistance to the disease. It seems complicated, but research into the validity of the hypothesis is under way (Fray et al. 1999).
Improved tolerance
Plant – microbe interactions are addressed in Chapter 10. Among the examples given are that of Pseudomonas syringae which colonises the surface of leaves. This example is of bacterial rather than plant modification but impinges on interaction between the two. Pseudomonas syringae produces a protein which promotes the formation of ice crystals just below 0 ◦C thus increasing the risk of frost damage. Lindow et al. (1989) have identified and isolated the gene for this protein. They transferred it to the bacterium Eschericia coli to simplify the genetic manipulations. This required the deletion of sufficient regions so that a truncated, and therefore nonfunctional, ice mediating protein was expressed. They reintroduced this mutated gene into Pseudomonas syringae and selected for ice− mutants which were no longer able to produce the ice nucleating protein. Many such regimes fail in practice because it is difficult to maintain a population of
Genetic Manipulation 229
mutant bacteria in a community dominated by the wild type as, frequently, the latter will soon outnumber the mutant by competition for nutrients, since it is usually better adapted to the particular environment than the mutant. However, in this case, due to massive application of Pseudomonas syringae ice− to strawberry plants, the mutants were able to compete with the wild type and protect this particularly susceptible crop against frost damage.
Salt tolerance in tomatoes has been established by introducing genes involved in Na+/H+ antiport, the transport of sodium and hydrogen ions in opposite directions across a membrane. The quality of the fruit was maintained by virtue of the fact that the sodium accumulation caused by the antiport occurred in leaves only and not in the fruit (Zhang and Blumwald 2001).
Improved tolerance to drought, salt and freezing in Arabidopsis has been achieved by overexpressing a protein which induces the stress response genes. However, if too much of this factor is produced, which was the case when the 35S CaMV was employed, severe growth retardation was observed. No such problem existed when instead, the overexpression was under the control of a promoter which was only switched on when stressful conditions existed (Kasuga et al. 1999).
Improved plants for phytoremediation
Chapter 7 mentioned the genetic modification of a poplar to enable mercury to be removed from the soil and converted to a form able to be released to the atmosphere. This process is termed ‘phytovolatilisation’ (Rugh et al. 1998). The modification required a gene to be constructed, styled on the bacterial mer A gene, by making a copy reflecting the codon bias found in plants using PCR technique. The mer A gene is one of a cluster of genes involved in bacterial detoxification of mercury, and is the one coding for the enzyme, mercuric ion reductase, which converts mercury from an ionic to a volatile form. Initially the constructed mer A gene was expressed in Arabidopsis thalia (rape) where resistance to mercury was observed, and in this study, the gene was transferred by microprojectile bombardment (‘gene guns’) to poplar tree (Liriodendrn tulipifera) embryogenic material. When the resulting yellow poplar plantlets were allowed to develop, they were found to exhibit tolerance to mercury and to volatalise it at 10 times the rate observed in untransformed yellow poplar plantlets. This study demonstrated the possibility that trees can be modified to become useful tools in the detoxification of soil contaminated with mercury. These studies were pursued in Arabidopsis thalia where it was observed that successful remediation also required the mer B genes coding for a lyase (Bizily, Rugh and Meagher 2000).
A bacterial gene encoding pentaerythritol tetranitrate reductase, an enzyme involved in the degradation of explosives, has been transferred into tobacco plants. The transgenics have been shown to express the correct enzyme and trials are under way to determine their ability to degrade TNT (French et al. 1999).
230 Environmental Biotechnology
Developments in the use of transgenic plants for bioremediation have been reviewed (Francova et al. 2001).
New products from plants
The rape plant, Arabidopsis thalia has become a popular choice for the production of recombinant species. One such recombinant is a rape plant, the fatty acid composition in the seed of which has been modified. It now produces triacylglycerols containing elevated levels of trierucinic acid suitable for use in the polymer industry (Brough et al. 1996) and, in a separate project, polyhydroxybutyrate suitable for the production of biodegradable plastics (Hanley, Slabas and Elborough 2000). Synthesis of the copolymer poly(3-hydroxybutyrate – co – 3-hydroxyvalerate) by Arabidopsis, is another example of the application of Agrobacterium tumefaciens technology and the use of the 35S promoter from Cauliflower Mosaic Virus (Slater et al. 1999). This copolymer can be produced by bacterial fermentation, but due to cost considerations, it is normally synthesised chemically. This example is discussed further in Chapter 10 under the umbrella of ‘clean’ technology. Progress in this field has been reviewed recently (Snell and Peoples 2002).
Closing Remarks
It is something of an irony that genetic engineering is virtually synonymous with biotechnology. The advances in this field have been enormous and, in many areas, are of singularly great importance, yet its impact has been much less dramatic when considering the purely environmental aspects. So much of what has been discussed in this chapter has not managed to make the wholesale transition into mainstream commercial activity and whether it ever will still remains to be seen. Unquestionably, GE will continue to play a definitive role in the future development of the biological sciences; its position in respect of practical environmental biotechnology is, perhaps, much less clear at this point.
References
Bajrovic, K., Erdag, B., Atalay, E.O. and Cirakoclu, B. (2001) Full resistance to Tobacco Mosaic Virus infection conferred by the transgenic expression of a recombinant antibody in tobacco, Biotechnology and Biotechnological Equipment, 15: 21 – 7.
Beggs, J.D. (1981) Gene cloning in yeast, Genetic Engineering 2 (ed. Williamson, R.) 175 – 203, Academic Press, London.
Bialy, H. (1997) Comment – biotechnology, bioremediation, and blue genes,
Nature Biotechnology, 15: 110.
Genetic Manipulation 231
Bizily, S.P., Rugh, C.L. and Meagher, R.B. (2000) Phytodetoxification of hazardous organomercurials by genetically engineered plants, Nature Biotechnology, 18: 213 – 17.
BMB (1995) Biotechnology Means Business Status Report Textile and Clothing Industries, DTI, London.
Brough, C.L., Coventry, J.M., Christie, W.W., Kroon, J.T.M., Brown, A.P., Barsby, T.L. and Slabas, A.R. (1996) Towards the genetic engineering of triacylglycerols of defined fatty acid composition: major changes in erucic acid content at the sn-2 position affected by the introduction of a 1-acyl-sn-glycerol- 3-phosphate acyltransferase from Limnanthes douglasii into oil seed rape,
Molecular Breeding, 2: 133 – 42.
Cho, H.S., Cao, J., Ren, J.P. and Earle, E.D. (2001) Control of Lepidopteran insect pests in transgenic Chinese cabbage (Brassica rapa ssp pekinensis) transformed with a synthetic Bacillus thuringiensis cry1C gene, Plant Cell Reports,
20: 1 – 7.
Cook, M.A., Osborn, A.M., Bettandorff, J. and Sobecky, P.A. (2001) Endogenous isolation of replicon probes for assessing plasmid ecology of marine sediment microbial communities, Microbiology, 147: 2089 – 101.
De Cosa, B., Moar, W., Lee, S.B., Miller, M. and Daniell, H. (2001) Overexpression of the Bt cry2Aa2 operon in chloroplasts leads to formation of insecticidal crystals, Nature Biotechnology, 19: 71 – 4.
Deak,´ M., Horvath,´ G.V., Davletova, S., Tor¨ok,¨ K., Sass, L., Vass, I., Barna, B., Kiraly,´ Z. and Dudits, D. (1999) Plants ectopically expressing the iron-binding protein, ferritin, are tolerant to oxidative damage and pathogens, Nature Biotechnology, 17: 192 – 6.
Francova, K., Macek, T., Demnerova, K. and Mackova, M. (2001) Transgenic plants – a potential tool for decontamination of environmental pollutants,
Chemicke Listy, 95: 630 – 7.
Fray, R.G., Throup, J.P., Daykin, M., Wallace, A., Williams, P., Stewart, G.S.A.B. and Grierson, D. (1999) Plants genetically modified to produce N-acylhomoserine lactones communicate with bacteria, Nature Biotechnology, 17: 1017 – 20.
French, C.E., Rosser, S.J., Davies, G.J., Nicklin, S. and Bruce, N.C. (1999) Biodegradation of explosives by transgenic plants expressing pentaerythritol tetranitrate reductase, Nature Biotechnology, 17: 491 – 4.
Godwin, I., Fordlloyd, B. and Newbury, H. (1992) In vitro approaches to extending the host-range of agrobacterium for plant transformation, Australian Journal of Botany, 40: 751 – 63.
Grierson, D. and Schuch, W. (1993) Control of ripening, Philosophical Transactions of the Royal Society of London, 342:241 – 50.
Hanley, Z., Slabas, T. and Elborough, K.M. (2000) The use of plant biotechnology for the production of biodegradable plastics, Trends In Plant Science, 5: 45 – 6.
He, M., Yang, Z.Y., Nie, Y.F., Wang, J. and Xu, P.L. (2001) A new type of class 1 bacterial 5-enolpyruvylshikimate-3-phosphate synthase mutants with enhanced
232 Environmental Biotechnology
tolerance to glyphosate, Biochimica et Biophysica Acta-General Subjects, 1568: 1 – 6.
Hughes, M.A. (1996) Plant Molecular Genetics, Longman, Essex, pp. 77 – 105. Kasuga, M., Liu, Q., Miura, S., Yamaguchi-Shinozaki, K. and Shinozaki, K. (1999)
Improving plant drought, salt, and freezing tolerance by gene transfer of a single stress-inducible transcription factor, Nature Biotechnology, 17: 287 – 91.
Kota, M., Daniell, H., Varma, S., Garczynski, S.F., Gould, F. and Moar, W.J. (1999) Overexpression of the Bacillus thuringiensis (Bt) Cry2Aa2 protein in chloroplast confers resistance to plants against susceptible and Bt-resistant insects, Proceedings of the National Academy of Sciences of the United States of America, 96: 1840 – 5.
Koziel, M.G., Beland, G.L., Bowman, C., Carozzi, N.B., Crenshaw, R., Crossland, L., Dawson, J., Desai, N., Hill, M., Kadwell, S., Launis, K., Lewis, K., Maddox, D., McPherson, K., Meghji, M.R., Merlin, E., Rhodes, R., Warren, G.W., Wright, M. and Devola, S.V. (1993) Field performance of elite transgenic maize plants expressing an insecticidal protein derived from Bacillus thuringiensis, Biotechnology, 11: 194 – 200.
Le, V.Q., Belles-Isles, J., Dusabenyagasani, M. and Tremblay, F.M. (2001) An improved procedure for production of white spruce (Picea glauca) transgenic plants using Agrobacterium tumefaciens, Journal of Experimental Botany, 52: 2089 – 95.
Lindow, S.E., Panopoulos, N.J. and McFarland, B.L. (1989) Genetic engineering of bacteria from managed and natural habitats, Science, 244: pp. 1300 – 7.
Magg, T., Melchinger, A.E., Klein, D. and Bohm, M. (2001) Comparison of Bt maize hybrids with their non-transgenic counterparts and commercial varieties for resistance to European corn borerand for agronomic traits, Plant Breeding, 120: 397 – 403.
Pessi, G., Blumer, C. and Haas, D. (2002) lac z fusions report gene expression, don’t they? Microbiology, 147: 1993 – 5.
Promega (1996) Protocols and Applications Guide, 3rd edition, Promega Corporation, USA.
Reanney, D. (1976) Extrachromosomal elements as possible agents of adaptation and development, Bacteriological Reviews, 40: pp. 552 – 90.
Ruf, S., Hermann, M., Berger, I.J., Carrer, H. and Bock, R. (2001) Stable genetic transformation of tomato plastids and expression of a foreign protein in fruit,
Nature Biotechnology, 19: 870 – 5.
Rugh, C.L., Senecoff, J.F., Meagher, R.B. and Merkle, S.A. (1998) Development of transgenic yellow poplar for mercury phytoremediation, Nature Biotechnology, 16: 925 – 8.
Shah, D.M., Horsch, R.B., Klee, H.J., Kishore, G.M., Winter, J.A., Tumer, N.E., Hironaka, C.M., Sanders, P.R., Gasser, C.S., Aykent, S., Siegel, N.R., Rogers, S.G. and Fraley, R.T. (1986) Engineering herbicide tolerance in transgenic plants, Science, 233: 478 – 481.
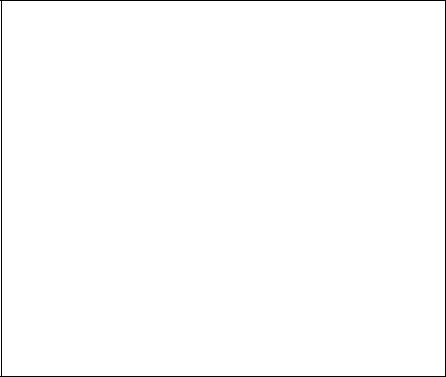
|
|
Genetic Manipulation 233 |
|
Slater, S., Mitsky, T.A., Houmiel, K.L., Hao, |
M., Reiser, |
S.E., Taylor, |
|
N.B., Tran, M., |
Valentin, H.E., Rodriguez, D.J., |
Stone, D.A., |
Padgette, S.R., |
Kishore, G. and |
Gruys, K.J. (1999) Metabolic |
engineering of Arabidopsis |
and Brassica for poly (3-hydroxybutyrate-co-3-hydroxyvalerate) copolymer production, Nature Biotechnology, 17: 1011 – 16.
Snell, K.D. and Peoples, O.P. (2002) Polyhydroxyalkanoate polymers and their production in transgenic plants, Metabolic Engineering, 4: 29 – 40.
Stewart, L.M.D., Hirst, M., Ferber, M.L., Merryweather, A.T., Cayley, P.J. and Possee, R.D. (1991) Construction of an improved baculovirus insecticide containing an insect-specific toxin gene, Nature, 352: 85 – 8.
Yordanova, E., Gorinova, N. and Atanassov, A. (2001) The use of cytochrome P450 monooxygenase genes to introduce herbicide tolerance in tobacco,
Biotechnology and Biotechnology Equipment, 15: 49 – 55.
Zhang, H.-X. and Blumwald, E. (2001) Transgenic salt-tolerant tomato plants accumulate salt in foliage but not in fruit, Nature Biotechnology, 19: 765 – 8.
Zhang, L., Xu, J. and Birch, R. (1999) Engineered detoxification confers resistance against a pathogenic bacterium, Nature Biotechnology, 17: 1021 – 4.
Case Study 9.1 Engineered Salt Tolerance (Rehovot, Israel)
One area where agrobiotechnological advances could have distinctly environmental applications, in the widest sense, is in the production of transgenic plants. While much of this research has centred on greater productivity, some work has been done to address other issues and one of the potentially most important of these relates to improving salt tolerance.
According to some authorities, over half the world’s agricultural land is expected to become increasingly saline within the next 50 years. In some countries, such as Israel where this work was pioneered, fresh water supplies are already stretched to the point that farmers are forced to use a proportion of salty water for irrigation and it is anticipated that this usage will continue to grow in the future. If encroaching desertification is to be avoided under these circumstances, then the development of salt-resistant crops and trees becomes essential.
Researchers at the Hebrew University in Rehovot isolated the protein, BspA, which is produced by the European aspen, Populus tremula, when growing in salty conditions and appears to protect the tree’s cells from damage by attracting water molecules, though the exact mechanism is still unknown. By introducing the appropriate genes into other plant species, it is hoped that the varieties produced may be afforded the same kind of protection.
Attempts to achieve this have been undertaken and the abilities of a related tree, the Euphrates poplar, Populus euphratica, which is even more salt-tolerant are also under investigation to see if it has other, additional methods which could likewise be incorporated into future generations of transgenic crops. If this work proves successful, it would herald a major breakthrough in mitigating a major cause of global environmental degradation.