
Environmental Biotechnology - Theory and Application - G. M. Evans & J. C. Furlong
.pdf204 Environmental Biotechnology
There are many of advantages to this method, most obviously in that it allows for the input biowaste to undergo established sanitisation procedures without detriment to the worms themselves, which are, as discussed previously, temperature sensitive. A less commonly appreciated benefit of this approach is that the period of initial composting significantly reduces worm ammonia exposure, to which, again, they are very sensitive. However, as with so much of these combined approaches, there is a need to manage the treatment conditions carefully to produce the optimisation desired. There is evidence to suggest that a precomposting phase has a negative effect on worm growth and reproduction rates (Frederickson et al. 1994) which obviously represents a direct reduction in the overall rate of worm biomass increase. Obviously this has an effect on the overall rate of stabilisation and processing, particularly since it has been demonstrated that the enhanced waste stabilisation achieved under worm treatment is only attained under conditions of high resident worm biomass (Frederickson et al. 1994). It seems reasonable to suggest, then, that to maximise the effectiveness of the combined treatment approach, the initial composting period should be no longer than the minimum necessary to bring about pathogen control of the input biowaste. Though this represents the kind of compromise balancing act so typical of much of environmental biotechnology, it is one which holds much promise. The combination of annelidic conversion with composting permits both enhanced stabilisation rate and product quality, with the additional bonus that the volatile organic content is also significantly reduced. It is also possible that the natural ability of worms to accumulate various hazardous substances within their bodies will also have implications for waste treatment, particularly if it proves possible to use them deliberately to strip out particular contaminating chemicals.
Annelidic conversion is currently very clearly a minority technology in this role, but the potential remains for it to play a role in the future biological treatment of waste, either as a standalone or, as seems more likely, as part of an integrated suite of linked processes. This seems particularly likely if the characteristically superior product derived from this process can be shown to be consistent, since specialist materials in the horticultural and gardening market generally tend to offer better returns. However, only time will tell whether this will prove to be sufficient financial incentive to offset the costs of production and encourage wider adoption of the technology.
Biowaste to ethanol
Around half of the total dry matter in plant origin biomass is cellulose, and since this makes up the majority of the biowaste component in MSW, it represents a huge potential source of renewable energy. As is widely appreciated, sugars can be broken down by certain micro-organisms to produce alcohols, of which ethanol (C2H5OH) is the most common. This is, of course, a well-known application for the production of alcoholic beverages across the world, typically using fermentative yeasts. These organisms are poisoned by ethanol accumulation
Biotechnology and Waste 205
greater than about 10% which means that, in order to derive higher concentrations, approaches relying on distillation or fractionating are required. In a wider context, ethanol, either as the typical hydrated form (95% ethanol, 5% water) or as azeotropically produced anhydrate, makes a good fuel with excellent general combustion properties.
Historically, the realisation of the huge energy source locked up in the sugars of the cellulose molecule has always been a practical impossibility. The combination of the β 1 – 4 linkage in cellulose itself, coupled with its typically close association with lignin, making large-scale hydrolysis to sugars a costly and difficult prospect. Some early attempts employed enzymes from wood-rotting fungi working on a feedstock of old newspapers or pulp, though the energy involved in actually making the process work often became a limiting factor. In the mid-1990s, various researchers began to investigate the potential of genetically modified bacteria, by inserting appropriate sequences from a variety of naturally occurring wood-rotting organisms. In the following years, a number of technologies have emerged, based both on whole-organism and isolated-enzyme techniques, and the commercial processing of cellulose to alcohol now appears to be about to become a mainstream reality.
A number of countries have begun to show an interest in the potential gains to be had from developing a biowaste-based ethanol industry. Within the USA, many individual states have started to undertake feasibility studies for their own areas. A recent California Energy Commission Report, for example, has established that the state-wide annual generation of biowaste exceeds 51 million dry tonnes, comprising forestry residue, MSW and agricultural waste. The same document estimates the resultant maximum ethanol yield at more than 3 billion gallons (US).
There are several thriving biomass-to-ethanol production plants elsewhere in the USA and the world, though most of these make their alcohol from primary crop plants, rather than biowaste. As with biogas, further discussion of the wider aspects of ethanol and the role of biotechnology in energy production appears in a later chapter.
Eutrophic fermentation (EF)
Eutrophic fermentation was the name given by one of the authors to the experimental, wet, in-vessel, aerobically enhanced biodegradation process he designed to investigate accelerated decomposition, principally as an alternative to anaerobic digestion. Fermentation in this context relates to the wider application of the word to encompass all microbial breakdown and not simply the anaerobic production of alcohol and eutrophic refers to the nutrient-rich environment within which it takes place.
The process arose as a result of research into the enhanced aerated remediation of post-anaerobic digestion liquor. The idea of introducing air into liquid or slurrified waste, as was discussed in Chapter 6, is well established as a means
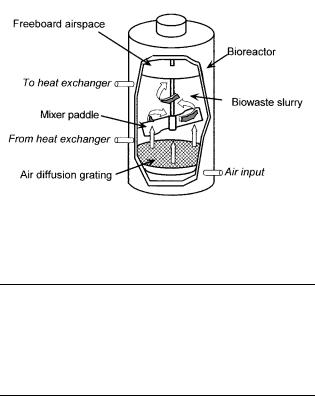
206 Environmental Biotechnology
of treatment. A laboratory prototype treatment was developed for the specific effluent and was successfully trailed at pilot scale, before subsequently being further developed and extended to the treatment of biowaste.
Eutrophic fermentation involves rendering the waste into a fine slurry, which is then contained within a bioreactor (Figure 8.4), aerated by means of bubble diffusers at the base of the vessel, mixed and heated to around 35 ◦C. Within this environment, the input waste breaks down to leave only about 10% of the original volume as recoverable solids within 35 days. The process liquor itself is characteristically less strong than for anaerobic digestion and typically contains only some 6 – 10% suspended solids. Analysis of this liquid by the Agricultural Development Advisory Service (ADAS) has indicated that it has some potential fertiliser use, based on key performance indicators such as nitrogen, phosphorous and potassium levels, electrical conductivity, generalised nutrient content and heavy metal residues. The results are shown in full in Tables 8.4 and 8.5.
With a ‘satisfactory’ pH of 6, a ‘low’ BOD of 2790 mg/l, low ammoniacal nitrogen and the likely slow release of much of the total nitrogen content over
Figure 8.4 EF bioreactor
Table 8.4 EF analysis results (nutrients)
Principal nutrients |
Units per 1000 |
Comments |
|
gallons |
|
Nitrogen (ammoniacal) |
2 |
Low |
Nitrogen |
5 |
Moderate |
Magnesium |
<1 |
Low |
Phosphorus |
1 |
Low |
Potassium |
6 |
Moderate |
Standard UK agricultural measure for fertiliser value.

Biotechnology and Waste 207
Table 8.5 EF analysis results (metals)
Metal |
mg/l |
Comments |
|
|
|
Cadmium |
<0.25 |
Low |
Chromium |
<1 |
Low |
Copper |
<1 |
Low |
Lead |
<1 |
Low |
Mercury |
<0.01 |
Low |
Nickel |
<1 |
Low |
Zinc |
2.7 |
Low |
|
|
|
several months, ADAS concluded that the liquor was a ‘useful source of nitrogen and potash for crop growth’.
Though this method proved itself remarkably efficient in laboratory and intermediate trials, the company for which it was developed subsequently abandoned further research into biological waste treatment and, consequently, EF has never been taken to pilot scale. As a result, it is not possible to say how well it might perform, though for such aerobic processes utilising a completely mixed, suspended growth regime, even relatively small-scale studies tend to be fair representations of full-scale performance. This is particularly relevant to this system, as the hydrolysed organics are directly assimilated by the resident microbes, rather than simply accumulating in the process liquor.
In the foreseeable future, it is unlikely that this approach will make the transition to commercial application, not least as a result of outstanding intellectual property questions. Nevertheless, it stands as a worthwhile example of how alternative technologies can be developed to meet particular needs.
The issue of local circumstance is a recurrent theme throughout the whole of environmental biotechnology and probably so in waste management as much, if not more, than in any other aspect. As a consequence, it is highly unlikely that any one technology will establish itself as the monopoly treatment, which effectively also guarantees the scope for the further development of existing or novel minority biotechnologies.
Closing Remarks
Biowaste management cannot solely be about maximising the diversion of biodegradable material from landfill; it must seek to maximise the return of materials thus diverted back into the chain of utility as an equal priority. This is a particular consideration for those biological treatment processes that result in some kind of compost or similar product. Though it is beyond the scope of this book to discuss the issues of marketing and product quality in depth, it must be obvious that any significant level of biowaste diversion via these approaches demands
208 Environmental Biotechnology
a sizeable and sustainable outlet. Suffice it to say that any such marketing operation requires significant assurances to the customer in terms of the material’s quality, safety and value and moves are afoot to establish more widely agreed and accepted criteria. To this end, developments like the application of specific oxygen uptake rate (SOUR) testing as a means of objectively assessing microbial activity within the composting matrix may have a wider role to play.
Another area of concern often expressed is that of pathogen persistence, which leads many to view the need for sanitisation as synonymous with sterilisation, which, of course, it is not. The health risks potentially associated with biowaste processing to both workers and end-users has been well documented elsewhere and it is not our intention to restate that work here. However, what is less widely appreciated, and is more directly relevant to our central theme, is that particularly for large-scale applications a balanced and thriving community of micro-organisms is one of the most valuable contributions a good biowastederived soil amendment can make to poor soils. Removing pathogens, weed seeds and spores from biologically processed waste, while not producing a sterile wasteland, remains one of the key balancing acts of biowaste treatment.
Ironically, it is the expansion of biotechnology in areas beyond the development of better systems for immediate biowaste treatment which is likely to have major implications for waste management. For any processing technology, there is clear advantage in having a relatively pure input material and this has led to much discussion over the years regarding the respective benefits of separation on site against householder segregation. The latter approach has itself led to the plastic bags in which the biowaste so segregated becoming something of a nuisance at central treatment facilities, typically needing to be opened and removed, which is a labour-intensive operation at such a large scale. The increasing use of truly biodegradable plastics has already started to have an impact on the situation, especially at composting plants, since bags which will themselves decompose significantly reduce the amount of work involved.
If the predicted widespread use of cheap bioplastics, grown as alternative crops in transgenic plants, becomes a reality in the future, then this may itself have repercussions for the amount of material requiring biological treatment. Plastics account for around 8 – 10% of the developed world’s waste stream and while reducing the demand for finite oil resources for the production of polymers has much to recommend it, one inevitable consequence will be to increase the amount of expressly biodegradable material in refuse. With growing numbers of countries looking to increase biowaste diversion, even allowing for effective recycling initiatives and attempts at waste minimisation, biotechnology may play an even larger part in the approaches to integrated waste management of the future.
Biotechnology and Waste 209
References
Bess, V. (1999) Evaluating the microbiology of compost, BioCycle, May, pp. 62 – 3.
Cope, C. (1995) An Introduction to the Chemistry of Landfill Gas and Leachate, Centre for Environmental Research and Consultancy (CERC, Cambridge).
Denham, C. (1996) Large Scale Profitable Manure Disposal, Booklet 2 Wonder Worms, Sowerby Bridge, UK.
DETR, Department of the Environment, Transport and the Regions (1999a) Limiting Landfill: A Consultation Paper on Limiting Landfill to Meet the EC Landfill Directive’s Targets for the Landfill of Biodegradable Municipal Waste, Crown copyright, pp. 11 – 12.
DETR, Department of the Environment, Transport and the Regions (1999b) A Way with Waste; A Draft Strategy for England and Wales, Part Two, Crown copyright, p. 90.
Dolk, H., Vrijheid, M., Armstrong, B., Abramsky, L., Bianchi, F., Garne, E., Nelen, V., Robert, E., Scott, J., Stone, D. and Tenconi, R. (1998) Risk of congenital anomalies near hazardous-waste landfill sites in Europe: the EUROHAZCON study, Lancet, 352: 423 – 7.
Elliott, P., Briggs, D., Morris, S., de Hoogh, C., Hurt, C., Kold Jensen, T., Maitland, I., Richardson, S., Wakefield, J. and Jarup, L. (2001) Risk of adverse birth outcomes in populations living near landfill sites, British Medical Journal, 323: 363 – 8.
Frederickson, J., Butt, K., Morris, R. and Daniel, C. (1994) Combining Vermiculture with Traditional Green Waste Composting Systems, presented at the 5th International Symposium on Earthworm Ecology, 5 – 9 July Columbus, Ohio.
Frostell, B. (1992) The role of biological waste treatment in integrated waste management, Proceedings of Biowaste ’92, ISWA/DAKOFA, Copenhagen, p. 4.
Lemmes, B. (1998) The ‘Tao’ of organics, Wastes Management, September: 18. Menon, S. (1994) Worms recruited to clear Bombay’s rubbish, New Scientist,
17th December: 5.
Monserrate, E., Leschine, S.B., Canale-Parola, E. (2001) Clostridium hungatei sp. nov., a mesophilic, N2-fixing cellulolytic bacterium isolated from soil, International Journal of Systematic and Evolutionary Microbiology, 51: 123 – 32.
Vrijheid, M. (2000) Health effects of residence near hazardous waste landfill sites: a review of epidemiologic literature, Environmental Health Perspectives, 108: 101 – 12.
Vrijheid, M., Dolk, H., Armstrong, B., Abramsky, L., Bianchi, F., Fazarinc, J., Garne, E., Ide, R., Nelen, V., Robert, E., Scott, J., Stone, D. and Tenconi, R. (2002) Chromosomal congenital anomalies and residence near hazardous waste landfill sites, Lancet, 359: 320 – 2.
Wright, M. (1998) Home composting: real waste minimisation or just feel good factor? Wastes Management, September: 27 – 8.
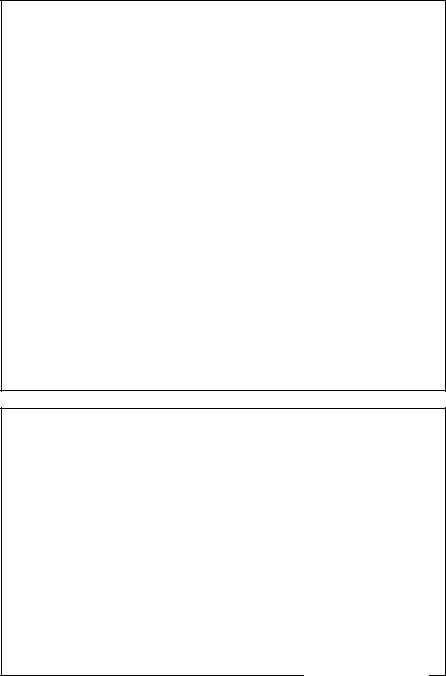
210 Environmental Biotechnology
Case Study 8.1 Integrated Waste Management (Zurich, Switzerland)
As a method of waste management, anaerobic digestion has much to offer, especially considering its potential for integration into strategies designed to address wider environmental issues. The Otelfingen Kompogas plant, near Zurich airport, Switzerland, provides an excellent example.
Constructed in 1997, the half-hectare (1 41 acre) facility processes around 14 000 tonnes of source separated biowaste annually, of which some 70% is domestic, the remainder being generated by commercial activities such as agriculture and food processing. Incoming waste is shredded and screened to remove any residual contaminants before being mixed with water to produce a slurry of 28% solids content for digestion. The bioreactor is of thermophilic plug flow design and has a retention period of 14–21 days. After processing, the digestate is dewatered by means of a screw press and the solid component, which is typically well within the relevant local heavy metal limits, is taken offsite and applied to farmland. Some of the water element is returned for reuse in the plant, while the excess is a valuable fertiliser substitute.
The biogas arising is of relatively high quality, being around 60% methane, and this energy source is exploited in two ways. Firstly, onsite engines are used to generate heat and electricity, which both covers the needs of the plant and produces a sizeable surplus for sale. Secondly, the company has invested in technology to clean up the gas to use as a fuel for its vehicle fleet, contributing a significant cost saving to its operational budget.
As a final example of biological integration, and of public and private sector cooperation, 50% funding from the Swiss government enabled the establishment of aquaculture greenhouses alongside the facility, where attenuated digester effluent is used to grow a variety of aquatic macrophytes.
Case Study 8.2 Co-composting for Reclamation (Colorado, USA)
The main advantages of composting as a means of biological waste treatment lie in its applicability to a wide range of materials and the potentially useful product which arises.
The reclamation of the Climax Molybdenum Mine, Leadville, Colorado required the revegetation of nearly 1600 hectares (4000 acres) of disturbed land, typically covered in tailings–the waste rock residue left after ore extraction. This was far from a straightforward task, since not only does such material provide a very poor footing for plants in the wind-buffered slopes, but also the growing season is only six or eight weeks long, the average temperature is slightly above 0 ◦ C and nearly 7 metres (23 feet) of snow falls yearly.
The approach employed to overcome these difficulties is a good example of both the versatility of composting and the ability of biotechnology to provide a contemporary and integrated solution to more than one environmental problem. The burgeoning population and rapid growth in the resort towns of Summit County caused major strain on the existing arrangements for the treatment of sewage
Continued on page 211
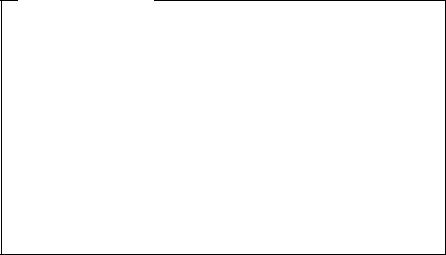
Biotechnology and Waste 211
Continued from page 210
biosolids, driving up disposal costs. At the same time, ski trail expansion, and the increased construction work, generated enormous amounts of wood waste. After a good deal of negotiation, a cooperative venture was launched between the mine’s owners, local sanitation facilities, land clearance firms, construction companies and haulers to produce a suitable compost out of the abundantly available materials.
Given the climatic conditions prevailing on the site, some 3300 metres (11 000 feet) above sea level, outdoor composting would seem an unlikely option, but considering the scale of the operation, highly engineered in-vessel systems would have been too expensive. By constructing oversized windrows to conserve heat and utilising effective control of aeration and careful blending of the input materials, it has proved possible to make good quality compost while also providing considerable economic benefits to all the parties involved.
To date, three years after it began, only around 40 hectares (100 acres) of the site has been reclaimed, so the project must, inevitably, be regarded as a very long-term one. However, the success of this combined biological treatment approach has already begun to stimulate great interest in both composting and reclamation in other areas with similar problems to solve.
9
Genetic Manipulation
Genes have been manipulated by man for a very long time, that is if selective breeding, which has been practised for centuries in agriculture and elsewhere to develop desirable characteristics in domesticated animals and plants, is to be considered as manipulation, as it rightly should. Even from the early days of Gregor Mendel, the Moravian monk and pioneer of genetic analysis, plants were bred to bring out interesting, useful and sometimes unusual traits. Many of these are now lost to classical plant breeders because of divergence of strains leading to infertile hybrids. One of the joys of genetic engineering is that in some cases, ancient genes may be rescued from seed found in archaeological digs for example, and reintroduced by transfer into modern strains. It has been proposed that the exchange of genetic information between organisms in nature is considerably more commonplace than is generally imagined (Reanney 1976) and could explain the observed rates of evolution. In bacteria, the most likely candidates for genetic transfer are plasmids and bacteriophage, and since eukaryotes lack plasmids, their most plausible vectors are eukaryotic viruses. This, of course, is in addition to DNA transfer during sexual reproduction. Current knowledge would suggest that exchange involving a vector requires compatibility between the organism donating the genetic material, the vector involved, and the recipient organism. For example, two bacteria must be able to mate for plasmid transfer to take place, or if a virus is involved as a vector, it must be able to infect both the donor and recipient cells or organisms. However, there is evidence to suggest that this view is somewhat na¨ıve and that there is considerably more opportunity for genetic exchange between all cells, prokaryotic and eukaryotic, than is popularly recognised. This idea, proposed by Reanney (1976) is developed in Chapter 3.
Bacteria are notorious for their ability to transfer genes between each other as the need arises thanks to the location on plasmids of most of the gene groups, or operons, involved in the breakdown of organic molecules. Strong evidence for the enormous extent of these ‘genomic pools’ comes from analysis of marine sediment (Cook et al. 2001). Throughout this book, the point has been made that micro-organisms involved in remediation do so in their ‘natural’ state largely because they are indigenous at the site of the contamination and have developed suitable capabilities without any external interference. However, sometimes after a sudden contamination such as a spill, microbes are not able to amass useful mutations to their DNA quickly enough to evolve suitable pathways to improve