
Radiation Physics for Medical Physiscists - E.B. Podgorsak
.pdf4.1 Collisions of Two Particles: General Aspects |
119 |
1.Heavy charged particles, such as protons, α particles or heavy ions, interact with the target through Coulomb interactions. Typical targets for heavy charged particles are atomic nuclei and atomic orbital electrons.
2.Light charged particles, such as electrons or positrons, interact with the target through Coulomb interactions. Typical targets for light charged particles are either atomic nuclei or atomic orbital electrons.
3.Neutrons interact with the target through direct collisions with the target. Typical targets for neutrons are atomic nuclei.
Targets are either atoms as a whole, atomic nuclei, or atomic orbital electrons. Two-particle collisions are classified into three categories: (1) nuclear reactions, (2) elastic collisions, and (3) inelastic collisions.
1.Nuclear reactions, shown schematically in Fig. 4.1 and discussed in Sect. 4.2, represent the most general case of a two-particle collision of a projectile m1 with a target m2 resulting in two reaction products, m3 and m4, that di er from the initial products m1 and m2.
–In any nuclear reaction a number of physical quantities must be conserved, most notably: charge; linear momentum and mass-energy.
–In addition, the sum of atomic numbers Z and the sum of atomic mass
numbers A for before and after the collision must also be conserved, i.e.,
ΣZ |
(before collision) = ΣZ |
(after collision) |
and |
|
|
ΣA |
(before collision) = ΣA |
(after collision). |
2.Elastic scattering is a special case of a two-particle collision in which:
–The products after the collision are identical to the products before the collision, i.e., m3 = m1 and m4 = m2.
–The total kinetic energy and momentum before the collision are equal to the total kinetic energy and momentum, respectively, after the collision.
–A small fraction of the initial kinetic energy of the projectile is transferred to the target.
Two-particle elastic scattering is shown schematically in Fig. 4.2 (p. 125). The energy transfer in elastic collisions is discussed in Sect. 4.3; the cross sections for single and multiple elastic scattering of two charged particles are discussed in Sect. 4.4.
3.In inelastic scattering of the projectile m1 on the target m2, similarly to elastic scattering, the reaction products after collision are identical to the initial products, i.e., m3 = m1 and m4 = m2; however, the incident projectile transfers a portion of its kinetic energy to the target in the form
of not only kinetic energy but also in the form of an intrinsic excitation energy E .

120 4 Two-Particle Collisions
The excitation energy E may represent:
–Nuclear excitation of the target.
–Atomic excitation or ionization of the target.
–Emission of bremsstrahlung by the projectile.
As a result of the various types of projectiles and targets as well as several categories of two-particle collisions, many di erent two-particle interactions are possible. The interactions of interest in medical physics and radiation dosimetry are summarized in Table 4.1.
Table 4.1. Collisions between various projectiles and targets of interest in medical physics and radiation dosimetry
Projectile |
Heavy charged |
Light charged |
Neutron |
|
||
|
particle |
|
particle |
|
|
|
|
|
|
|
|||
Target |
Nucleus Electron |
Nucleus Electron |
Nucleus Electron |
|||
|
|
|
|
|
|
|
Nuclear reaction |
Yes(a) |
No |
Yes(e) |
No |
Yes(j) |
No |
m2(m1, m3)m4 |
|
|
|
|
|
|
Elastic scattering |
Yes(b) |
No |
Yes(f ) |
Yes(h) |
Yes(k) |
No |
m2(m1,m1)m2 |
|
|
|
|
|
|
Inelastic scattering |
Yes(c) |
Yes(d) |
Yes(g) |
Yes(i) |
Yes(l) |
No |
m2(m1,m1)m2 |
|
|
|
|
|
|
Heavy charged particle interactions with nuclei of the target
(a)Nuclear reaction precipitated by a heavy charged particle projectile m1 striking a nucleus m2 resulting in products m3 and m4.
Example: Deuteron bombarding nitrogen-14
147 N(d, p)157 N
(b)Elastic Coulomb collision of heavy charged particle with atomic nucleus Example: Rutherford scattering of α particle on gold nucleus,
19779 Au(α, α)19779 Au
(c)Inelastic collision of heavy charged particle with nucleus Example: Nuclear excitation,
AZ X(α, α)AZ X AZ X → AZ X + γ
Heavy charged particle interactions with orbital electrons of the target
(d)Inelastic collision of heavy charged particle with atomic orbital electron Example: Excitation or ionization of an atom
4.2 Nuclear Reactions |
121 |
Light charged particle interactions with nuclei of the target
(e)Nuclear reaction precipitated by an energetic light charged particle striking the nucleus
Examples: (e,n) and (e,p) nuclear reactions
(f) Elastic collision between a light charged particle and atomic nucleus Example: Non-radiative scattering of electrons on the nuclei of the target
(g)Inelastic collision between a light charged particle and atomic nucleus Example: Bremsstrahlung production by electrons or positrons undergoing
a Coulomb interaction with an atomic nucleus (radiative loss)
Light charged particle interactions with orbital electrons of the target
(h)Elastic collision between a light charged particle and an orbital electron Example: Ramsauer e ect in which an electron of very low kinetic energy
(below 100 eV) undergoes an elastic collision with an atomic orbital electron
(i)Inelastic collision between a light charged particle and atomic orbital electron Example 1: Electron-orbital electron interaction resulting in atomic excita-
tion or ionization (hard and soft collisions)
Example 2: Positron annihilation leaving atom in an ionized state coinciding with emission of two γ annihilation quanta.
Neutron interactions with nuclei of the target
(j)Nuclear reaction caused by neutron colliding with atomic nucleus Example: 1: Neutron capture or neutron activation (see Sect. 8.4)
5927Co(n, γ)6027Co
Example 2: Spallation and nuclear fission for high atomic number targets
(k)Elastic collision between neutron and atomic nucleus (see Sect. 6.2.1)
(l)Inelastic collision between neutron and atomic nucleus (see Sect. 6.2.2) Example: Nuclear excitation
AZ X(n, n)AZ X AZ X → AZ X + γ
4.2 Nuclear Reactions
Two-particle collisions between the projectile m1 and target m2 resulting in products m3 and m4 are referred to as nuclear reactions and are governed by conservation of total energy and momentum laws. As shown in Table 4.1, the projectile can be a heavy charged particle, a light charged particle or a neutron.
The collision leading to a nuclear reaction is shown schematically in Fig. 4.1 with the projectile m1 moving with velocity υ1 and kinetic energy (EK)1 striking a stationary target m2. An intermediate compound entity is produced temporarily that decays into two reaction products, m3 and m4, ejected with velocities υ3 at angle θ and υ4 at angle φ, respectively.
122 4 Two-Particle Collisions
4.2.1 Conservation of Momentum in Nuclear Reactions
The conservation of momentum in a two-particle nuclear collision is expressed through the vector relationship
→ |
→ |
→ |
, |
(4.1) |
m1 υ1 |
= m3 υ3 |
+ m4 υ4 |
that can be resolved into a component along the incident direction and a component perpendicular to the incident direction to obtain
m1υ1 = m3υ3 cos θ + m4υ4 cos φ |
(4.2) |
and |
|
0 = m3υ3 sin θ − m4υ4 sin φ , |
(4.3) |
where the angles θ and φ are defined in Fig. 4.1 on page 118. |
|
4.2.2 Conservation of Energy in Nuclear Reactions
The total energy of the projectile m1 and target m2 before the interaction (collision) must equal to the total energy of products m3 and m4 after the collision, i.e.,
m |
c2 + (EK)1 |
+ (m2oc2 + 0) = |
(4.4) |
|||
|
1o m3oc2 |
+ (EK)3 |
+ m4oc2 |
+ (EK)4 , |
||
|
|
|
|
|
|
|
where
m1oc2 m2oc2 m3oc2 m4oc2
(EK)1 (EK)3 (EK)4
is the rest energy of the projectile; is the rest energy of the target;
is the rest energy of the reaction product m3; is the rest energy of the reaction product m4; is the initial kinetic energy of the projectile;
is the kinetic energy of the reaction product m3; is the kinetic energy of the reaction product m4.
Inserting into (4.4) the so-called Q value for the collision in the form
Q = (m1oc2 + m2oc2) − (m3oc2 + m4oc2) , |
(4.5) |
we get the following relationship for the conservation of energy |
|
EK1 + Q = EK3 + EK4 . |
(4.6) |
Each two-particle collision possesses a characteristic Q value that can be either positive, zero, or negative.
•For Q > 0, the collision is exothermic and results in release of energy.
•For Q = 0, the collision is termed elastic.
•For Q < 0, the collision is termed endothermic and, to take place, it requires an energy transfer from the projectile to the target.
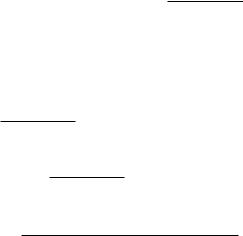
4.2 Nuclear Reactions |
123 |
4.2.3 Threshold Energy Ethr for Nuclear Reactions
An exothermic reaction can occur spontaneously; an endothermic reaction cannot take place unless the projectile has a kinetic energy exceeding a threshold energy.
•The threshold is defined as the smallest total energy Ethr or the smallest kinetic energy (EK)thr of the projectile at which an endothermic collision can still occur.
•The threshold energy for an endothermic collision is determined through the use of the so-called invariant
E2 − p2c2 = invariant , |
(4.7) |
where
E is the total energy before and the total energy after the collision;
pis the total momentum before and the total momentum after the collision;
The invariant is valid for both the laboratory coordinate system and for the center-of-mass coordinate system and, for convenience, the conditions before the collision are written for the laboratory system while the conditions after the collision are written for the center-of-mass system.
The conditions for before and after the collision are written as follows:
– total energy before: Ethr + m2oc2 = m21oc4 + p21c2 + m2oc2 , (4.8) where Ethr is the total threshold energy of the projectile;
–total momentum before: p1;
–total energy after in the center-of-mass system: m30c2 + m40c2;
–total momentum after in the center-of-mass system: 0.
The invariant of (4.7) for before and after the collision then gives
m1o2 c4 + p12c2 + m2oc2 |
2 |
− p12c2 = m3oc2 + m4oc2 2 − 0 . |
(4.9)
Solving for Ethr = m21oc4 + p21c2 results in the following expression for the total threshold energy
|
m3oc |
2 |
+ m4oc |
2 |
2 |
− |
2 |
4 |
2 |
4 |
|
|
|
|
|
|
|
m1oc + m2oc |
|
|
|
|
|||||
Ethr = |
|
|
|
2m2oc2 |
|
|
|
|
. |
(4.10) |
Noting that Ethr = (EK)thr + m1oc2, where (EK)thr is the threshold kinetic energy of the projectile, we get the following expression for (EK)thr
|
|
2 |
2 |
|
2 |
|
2o |
|
2 |
2 |
|
2 |
|
|
|
|
m3oc + m4oc |
|
|
|
m1oc + m2oc |
|
|
|
|
||||||
(EK)thr = |
|
|
|
|
− |
|
|
|
|
. |
(4.11) |
||||
|
|
|
|
|
|
c2 |
|
|
|
|
|||||
|
|
|
|
2m |
|
|
|
|
|
|
|
The threshold kinetic energy (EK)thr of the projectile may now be written in terms of the nuclear reaction Q value [(4.5)] as follows:

124 4 Two-Particle Collisions
– we note that from (4.5) for the Q value we can write
m3oc2 + m4oc2 2 = m1oc2 + m2oc2 2 |
|
+Q2 − 2Q m1oc2 + m2oc2 . |
(4.12) |
–Inserting the relationship of (4.12) into (4.11) we obtain
(EK)thr = −Q |
c2 + m |
2o |
c2 |
|
Q |
|
m1mo |
|
− |
|
|||
m2oc2 |
|
|
2m2oc2 |
m1o |
|
|
≈ −Q 1 + m2o |
, |
(4.13) |
where, since Q m2oc2, we can ignore the Q/(2m2oc2) term in (4.13).
In (4.13) the threshold kinetic energy (EK)thr of the projectile exceeds the |Q| value by a relatively small amount to account for conservation of both energy and momentum in the collision.
As a special case the invariant of (4.7) may also be used to calculate the threshold photon energy (Eγ )thr for pair production (see Sect. 7.6.2)
– In the field of nucleus of rest mass mA (pair production) as
(Eγp)thr = 2mec2 1 + |
me |
. |
(4.14) |
|
|||
|
mA |
|
– In the field of orbital electron of rest mass me (triplet production) as
(Eγt )thr = 4mec2 . |
(4.15) |
4.3 Two-Particle Elastic Scattering: Energy Transfer
Elastic scattering in a two-particle collision is a special case of a nuclear collision between a projectile m1 and target m2:
1.The initial and final products are identical (i.e., m3 = m1 and m4 = m2), however, the projectile changes its direction of motion (i.e., is scattered) and the target recoils.
2.The Q value for the collision, as given in (4.7), equals zero, i.e., Q = 0.
3.A certain amount of kinetic energy (∆EK) is transferred from the projectile m1 to the target m2. The amount of energy transfer is governed by conservation of the kinetic energy and momentum, and depends on the scattering angle θ of the projectile and the recoil angle φ of the target.
Two-particle elastic scattering between projectile m1 moving with velocity υ1 and a stationary target m2 is shown schematically in Fig. 4.2, with θ the scattering angle of the projectile, φ the recoil angle of the target, and b the impact parameter. After the collision particle m1 continues with velocity u1 and the target recoils with velocity u2.
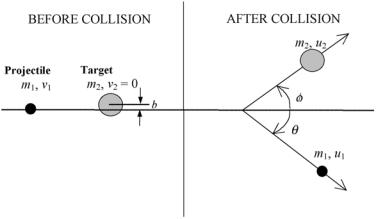
4.3 Two-Particle Elastic Scattering: Energy Transfer |
125 |
Fig. 4.2. Schematic diagram of an elastic collision between a projectile with mass m1 and velocity υ1 striking a stationary target m2. The projectile is scattered with a scattering angle θ; the target recoils with a recoil angle φ. The impact parameter is b. After the collision the velocity of the projectile m1 is u1; the velocity of the target m2 is u2
4.3.1 General Energy Transfer from Projectile m1 to Target m2 in Elastic Scattering
The kinetic energy transfer ∆EK from projectile m1 to the target m2 is determined classically using the conservation of kinetic energy and momentum laws as follows:
•Conservation of kinetic energy:
(EK)1 = |
1 |
m1υ12 |
= |
1 |
m1u12 |
+ |
1 |
m2u22 |
, |
(4.16) |
2 |
|
|
||||||||
|
|
|
2 |
|
2 |
|
|
where (EK)1 is the initial kinetic energy of the projectile m1.
•Conservation of momentum:
|
m1υ1 = m1u1 cos θ + m2u2 cos φ |
(4.17) |
|
0 = m1u1 sin θ − m2u2 sin φ , |
(4.18) |
where |
|
|
υ1 |
is the initial velocity of the projectile m1, |
|
u1 |
is the final velocity of the projectile m1, |
|
u2 |
is the final velocity of the target m2 , |
|
θ |
is the scattering angle of the projectile m1 , |
|
φ |
is the recoil angle of the target m2. |
|
Equations (4.17) and (4.18) can be written as follows: |
|
|
|
(m1υ1 − m2u2 cos φ)2 = m12u12 cos2 θ |
(4.19) |
126 4 Two-Particle Collisions and
m12u12 sin2 θ = m12u12 − m12u12 cos2 θ = m22u22 sin2 φ , |
(4.20) |
||||||
respectively. |
|
|
|
|
|
|
|
Inserting (4.19) into (4.20) gives |
|
||||||
m22u22 = m12u12 − m12υ12 + 2m1υ1m2u2 cos φ , |
(4.21) |
||||||
which, after inserting (4.16) multiplied by 2m1, reads |
|
||||||
m22u22 = 2m1υ1m2u2 cos φ − m1m2u22 or |
|
||||||
2m1υ1 cos φ = (m1 + m2) u2 . |
(4.22) |
||||||
Since ∆EK = |
m2u22 |
|
|
|
|
||
|
, we get the following classical general expression for the |
||||||
2 |
|||||||
kinetic energy transfer ∆EK from the projectile m1 to the target m2 |
|
||||||
∆EK |
= EK1 |
4m1m2 |
cos2 φ , |
(4.23) |
|||
(m1 |
+ m2)2 |
||||||
|
|
|
|
|
where φ is the recoil angle of the target m2, defined graphically in Fig. 4.2.
4.3.2 Energy Transfer in a Two-Particle Elastic Head-On Collision
A head-on (direct hit) elastic collision between two particles is a special elastic collision in which the impact parameter b equals to zero. This results in a maximum possible momentum transfer ∆pmax and maximum possible energy transfer (∆EK)max from the projectile m1 to the target m2. The head-on twoparticle elastic collision is characterized as follows:
1.The impact parameter b = 0.
2.The target recoil angle φ = 0.
3.The projectile scattering angle θ is either 0 or π depending on the relative magnitudes of masses m1 and m2
–for m1 > m2, θ = 0 (forward scattering),
–for m1 < m2, θ = π (back-scattering),
–for m1 = m2, the projectile stops and target recoils with φ = 0.
Classical Relationships for a Head-On Collision
Before collision |
After collision |
||
◦ → |
◦ |
◦ → |
◦ → |
m1, υ1 |
m2, υ2 = 0 |
m1, u1 |
m2, u2 |
Conservation of Momentum: m1υ1 + 0 = m1u1 + m2u2 |
(4.24) |
4.3 Two-Particle Elastic Scattering: Energy Transfer |
127 |
||||||||
|
m1υ12 |
|
m1u12 |
m2u22 |
|
||||
Conservation of Energy: |
|
+ 0 = |
|
|
+ |
|
|
|
(4.25) |
|
2 |
2 |
|
||||||
2 |
|
|
|
|
•The maximum momentum transfer ∆pmax from the projectile m1 to the target m2 is given by:
∆pmax = m1υ1 − m1u1 = |
2m1m2 |
|
= |
2m2 |
, (4.26) |
|
|
υ1 |
|
p1 |
|||
m1 + m2 |
m1 + m2 |
where p1 is the initial momentum of the projectile m1.
•The maximum energy transfer ∆Emax from the projectile m1 to the stationary target m2 is given by
|
m1υ12 |
|
m1u12 |
m2u22 |
|
4m1m2 |
|
||
∆Emax = |
|
− |
|
= |
|
= |
|
EK1 |
, (4.27) |
2 |
2 |
2 |
(m1 + m2)2 |
where EK1 is the initial kinetic energy of the projectile m1. The same result can be obtained from the general relationship given in (4.23) after inserting φ = 0 for the target recoil angle.
Special Cases for the Classical Energy Transfer in a Head-On Collision
• m1 m2 → ∆Emax = |
|
|
4m1m2 |
|
||
|
|
|
|
EK1 |
||
(m1 + m2)2 |
||||||
|
|
|
m2 |
υ12 |
||
≈ |
4 |
|
EK1 = 2m2 |
|||
m1 |
Example: proton colliding with orbital electron: mp me
∆Emax = |
4memp |
≈ 4 |
me |
= 2meυ12 |
||
|
EK1 |
|
EK1 |
|||
(me + mp)2 |
mp |
(4.28)
(4.29)
Since 4me/mp = 4/1836 ≈ 0.002, we see that in a direct hit between a proton and an electron only about 0.2% of the proton kinetic energy is transferred to the target electron in a single collision.
• |
m1 m2 → ∆Emax = |
4m1m2 |
|
≈ 4 |
m1 |
(4.30) |
||||||
|
|
EK1 |
|
|
EK1 |
|||||||
(m1 + m2)2 |
m2 |
|||||||||||
|
Example 1: α particle colliding with gold nucleus (Au-207): mα mAu |
|||||||||||
|
(Rutherford scattering, see Sect. 2.2) |
|
|
|
|
|
|
|
||||
|
∆Emax = 4 |
mamAu |
≈ 4 |
mα |
(4.31) |
|||||||
|
|
EK1 |
|
EK1 . |
||||||||
|
(mα + mAu)2 |
mAu |
Since 4mα/mAu ≈ 0.08, we see that in a single direct hit head-on collision only about 8% of the incident α-particle kinetic energy is transferred to the gold target.
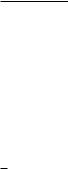
128 4 Two-Particle Collisions
Example 2: Neutron colliding with lead nucleus (Pb-207):
mneutron mlead nucleus
∆Emax = 4 |
mnmPb |
≈ 4 |
mn |
(4.32) |
||
|
EK1 |
|
EK1 . |
|||
(mn + mPb)2 |
mPb |
Since 4mn/mPb ≈ 1/50 = 0.02, we see that in a direct hit only about 2% of the incident neutron kinetic energy is transferred to the lead target. This shows that lead is a very ine cient material for slowing down neutrons; low atomic number materials are much more suitable for this purpose. Of practical importance here is the use of polyethylene as shielding material for doors in high-energy linac bunkers to shield against neutrons produced in the linac.
• |
m1 = m2 → ∆Emax = |
4m1m2 |
= EK1 |
(4.33) |
(m1 + m2)2 EK1 |
Example: Interaction between two distinguishable particles such as positron colliding with orbital electron or neutron colliding with hydrogen atom:
In a direct hit between two distinguishable particles of equal mass the whole kinetic energy of the incident particle is transferred to the target in a single hit.
• |
m1 = m2 → ∆Emax = |
1 |
(4.34) |
2 EK |
Example: Interaction between two indistinguishable particles such as electron colliding with orbital electron:
After the interaction, the particle with the larger kinetic energy is assumed to be the incident particle; therefore the maximum possible energy transfer is EK/2.
Relativistic Relationships for a Head-On Collision
The relationship for ∆Emax in (4.27) was calculated classically. The relativistic result given below is similar to the classical result, with m1o and m2o standing for the rest masses of the projectile m1 and target m2.
Before collision |
After collision |
||
◦ → |
◦ |
◦ → |
◦ → |
m1o, υ1 |
m2o, υ2 = 0 |
m1o, u1 |
m2o, u2 |
Conservation of Momentum: |
|
|
|
γβm1oc2 + 0 = γ1β1m1oc2 + γ2β2m2oc2 |
(4.35) |