
3513
.pdf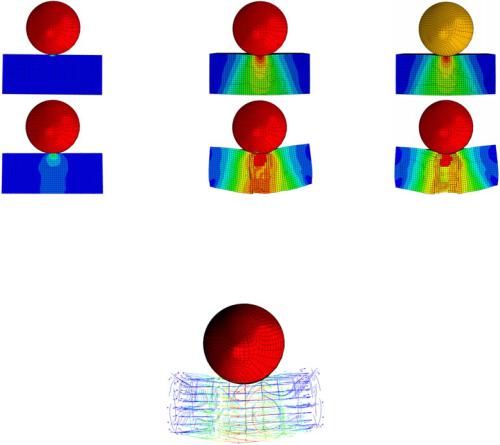
Issue № 3 (39), 2018 |
ISSN 2542-0526 |
a) |
b) |
c) |
d) |
e) |
f) |
Fig. 19. Simulation views representing three different steps: a) 0 % RSF/t = 0, b) 0 % RSF/t = 50, c) 0 % RSF/t=100, d) 2.5 % RSF/t = 0, e) 2.5 % RSF/t = 63 and f) 2.5 % RSF/t = 100
Fig. 20. The displacement and deflection views of RSF's
5. Conclusion. Wires of waste tires are extracted with different methods for use in cementitious materials. In this study, the selected combustion method to extract the wire of the tires in large volumes by proposed self-fabricated device seems to be a very fast and financially appropriate method. But the yield strength of the obtained wires is much decreased compared to the original case, i.e. from 1400 to 445 MPa. However, the mechanical and impact properties of the reinforced mortar specimens with the fibers obtained from these wires showed very good results. The results obtained are discussed below for different properties. According to compressive test results, the strengths of the specimens with 1, 1.5 and 2 % RSF contain increased by 9.8, 16.55 and 23.3 % respectively. But the strength of the specimen with 2.5 % RSF contain was increased compared to those of specimens with 1.5 and 2 % RSF contain. This may be related to the voids between RSFs with the increase in them. All flexural strength values were increased compared to the control specimen. The highest flexural strength values are reported with specimens with 2 and 2.5 % RSF contain, with no significant difference between them, which, compared to the unreinforced specimen was increased
81

Russian Journal of Building Construction and Architecture
by 55.28 and 57.20 %, respectively. The toughness (area under the load displacement curves) values obtained from the flexural test result were greatly increased for specimens with 1.5, 2 and 2.5 % RSF contain especially. The increase times were calculated as 10, 13 and 18, respectively. Using the self fabricated tool, the ultimate energy values, obtained by the drop weight impact test method as the experimental method, were increased at a highe ratio compared the control specimen for all specimens with RSF contain. The increase rates of the energy values of the specimens with 1, 1.5, 2 and 25 % RSF containing compared to the control specimens are 3.64, 7.10, 8.55 and 10.14, respectively. The Eu =118.24RSF + 22.751 equation was proposed between increasing ultimate energy values. The acceptability of this equation has been confirmed because the obtained coefficient of determination, R² = 0.9755, is above the minimum value (0.70). The impact test results were compared with the FE method results, confirming that the results were very similar. In FEMs, due to the good bond definition between the matrix and the reinforcement elements, it was appeared that the reinforcement elements in the matrix are good influenced by the impact load. According to the effect at the time of impact loading, both the failure time and the displacement values increased with the increase of the RSF ratio of the specimens. While the unreinforced specimen failed with the first cracks occurring without to a significant displacement, the specimens with 2% RSF contain showed displacement above 9 mm to fail.
References
1.Bagherzadeh R., Sadeghi A.-H. and Latifi M. Utilizing polypropylene fibers to improve physical and mechanical properties of concrete. Textile Research Journal, 2012. 82(1): p. 88––96.
2.Grassl P. and Jirásek M. Damage-plastic model for concrete failure. International journal of solids and structures, 2006. 43(22): p. 7166––7196.
3.Zhou C. et al. Enhanced mechanical properties of cement paste by hybrid graphene oxide/carbon nanotubes. Construction and Building Materials, 2017. 134: p. 336––345.
4.Hsie M., Tu C. and Song P. Mechanical properties of polypropylene hybrid fiber-reinforced concrete. Materials Science and Engineering: A, 2008. 494(1): p. 153––157.
5.Yoo D.-Y. et al., Response of ultra-high-performance fiber-reinforced concrete beams with continuous steel reinforcement subjected to low-velocity impact loading. Composite Structures, 2015. 126: p. 233––245.
6.Caratelli A., Meda A. and Rinaldi Z. Monotonic and cyclic behaviour of lightweight concrete beams with and without steel fiber reinforcement. Construction and Building Materials, 2016. 122: p. 23––35.
7.Cai G. et al. Shear capacity of steel fibre reinforced concrete coupling beams using conventional reinforcements. Engineering Structures, 2016. 128: p. 428––440.
8.Masmoudi A. and Bouaziz J. Durability of Steel Fibres Reinforcement Concrete Beams in Chloride Environment Combined with Inhibitor. Advances in Materials Science and Engineering, 2016.
82
Issue № 3 (39), 2018 |
ISSN 2542-0526 |
9.Abdallah S. et al. Anchorage effects of various steel fibre architectures for concrete reinforcement. International Journal of Concrete Structures and Materials, 2016. 10(3): p. 325––335.
10.Maghool F. et al. Environmental impacts of utilizing waste steel slag aggregates as recycled road construction materials. Clean Technologies and Environmental Policy, 2017. 19(4): p. 949––958.
11.Akça K. R., Çakır Ö. and Ipek M. Properties of polypropylene fiber reinforced concrete using recycled aggregates. Construction and Building Materials, 2015. 98: p. 620––630.
12.Yilmaz A. and Degirmenci N. Possibility of using waste tire rubber and fly ash with Portland cement as construction materials. Waste Management, 2009. 29(5): p. 1541––1546.
13.Lee T. Leaching characteristics of bottom ash from coal fired electric generating plants, and waste tire; individually and mixtures when used as construction site fill materials. Waste management, 2011. 31(2): p. 246––252.
14.Yang H.-S. et al. Possibility of using waste tire composites reinforced with rice straw as construction materials. Bioresource technology, 2004. 95(1): p. 61—65.
15.Wang Y., Wu H. and Li V. C. Concrete reinforcement with recycled fibers. Journal of materials in civil engineering, 2000. 12(4): p. 314––319.
16.Onuaguluchi O. et al. Performance of scrap tire steel fibers in OPC and alkali-activated mortars. Materials and Structures, 2017. 50(2): p. 157.
17.Jo B. W., Chakraborty S. and Kim H. Efficacy of alkali-treated jute as fibre reinforcement in enhancing the mechanical properties of cement mortar. Materials and Structures, 2016. 49(3): p. 1093––1104.
18.Yildirim K. and Sümer M. Effects of sodium chloride and magnesium sulfate concentration on the durability of cement mortar with and without fly ash. Composites Part B: Engineering, 2013. 52: p. 56––61.
19.Zhang W., Zakaria M. and Hama Y. Influence of aggregate materials characteristics on the drying shrinkage properties of mortar and concrete. Construction and building materials, 2013. 49: p. 500––510.
20.Khattab M. M. Effect of gamma irradiation on polymer modified white sand cement mortar composites. Journal of Industrial and Engineering Chemistry, 2014. 20(1): p. 1––8.
21.Ambily P. et al. Studies on ultra high performance concrete incorporating copper slag as fine aggregate. Construction and Building Materials, 2015. 77: p. 233––240.
22.Ahmadi M. et al. Mechanical properties of the concrete containing recycled fibers and aggregates. Construction and Building Materials, 2017. 144: p. 392––398.
23.Ekolu S. O. Potential South African standard sand for cement mortar testing and research. in Construction Materials and Structures: Proceedings of the First International Conference on Construction Materials and Structures, 2014. IOS Press.
24.EN, T., 196-1. Methods of testing cement–Part 1: Determination of strength. European Committee for standardization, 2005. 26.
25.Idir R., Cyr M. and Tagnit-Hamou A. Pozzolanic properties of fine and coarse color-mixed glass cullet. Cement and Concrete Composites, 2011. 33(1): p. 19––29.
26.Bilim C. and Atiş C. D. Alkali activation of mortars containing different replacement levels of ground granulated blast furnace slag. Construction and Building Materials, 2012. 28(1): p. 708––712.
27.Pereira-de-Oliveira L. A., Castro-Gomes J. P. and Santos P. M. The potential pozzolanic activity of glass and red-clay ceramic waste as cement mortars components. Construction and Building Materials, 2012. 31: p. 197––203.
83

Russian Journal of Building Construction and Architecture
28.Xu S., Gao L. and Jin W. Production and mechanical properties of aligned multi-walled carbon nanotubesM140 composites. Science in China Series E: Technological Sciences, 2009. 52(7): p. 2119––2127.
29.Sun W. et al. Damage and damage resistance of high strength concrete under the action of load and freezethaw cycles. Cement and Concrete Research, 1999. 29(9): p. 1519––1523.
30.Yahaghi J., Muda Z. C. and Beddu S. B. Impact resistance of oil palm shells concrete reinforced with polypropylene fibre. Construction and Building Materials, 2016. 123: p. 394––403.
31.Nili M. and Afroughsabet V. The effects of silica fume and polypropylene fibers on the impact resistance and mechanical properties of concrete. Construction and Building Materials, 2010. 24(6): p. 927––933.
32.Enfedaque A. et al. Numerical simulation of the fracture behaviour of glass fibre reinforced cement. Construction and Building Materials, 2017. 136: p. 108––117.
33.Mahmud G. H., Yang Z. and Hassan A. M. Experimental and numerical studies of size effects of Ultra High Performance Steel Fibre Reinforced Concrete (UHPFRC) beams. Construction and Building Materials, 2013. 48: p. 1027––1034.
34.Elsanadedy H. M. et al. Flexural strengthening of RC beams using textile reinforced mortar–Experimental and numerical study. Composite Structures, 2013. 97: p. 40––55.
35.Limazie T. and Chen S. FE modeling and numerical investigation of shallow cellular composite floor beams. Journal of Constructional Steel Research, 2016. 119: p. 190––201.
36.Chang C. et al. Temperature and mixing effects on electrical resistivity of carbon fiber enhanced concrete. Smart materials and structures, 2013. 22(3): p. 035021.
37.Hibbitt H., Karlsson B. and Sorensen P. Abaqus analysis user’s manual version 6.10. Dassault Systèmes Simulia Corp.: Providence, RI, USA, 2011.
38.Lubliner J. et al. A plastic-damage model for concrete. International Journal of solids and structures, 1989. 25(3): p. 299––326.
39.Huang Y. et al., Monte Carlo simulations of meso-scale dynamic compressive behavior of concrete based on X-ray computed tomography images. International Journal of Impact Engineering, 2016. 97: p. 102––115.
40.Zhao H. et al. Influence of pore structure on compressive strength of cement mortar. The Scientific World Journal, 2014.
41.Properties A. S. D. o. M. Standard test methods for flexural properties of unreinforced and reinforced plastics and electrical insulating materials. 1997. American Society for Testing Materials.
42.Alvarez G. L. et al. Microstructure, electrical and mechanical properties of steel fibres reinforced cement mortars with partial metakaolin and limestone addition. Construction and Building Materials, 2017. 135: p. 8––20.
43.Mousavi S. E. Flexural response and crack development properties of ferrocement panels reinforced with steel fibers. Journal of Building Engineering, 2017. 12: p. 325––331.
44.Foudazi A. et al. Evaluation of steel fiber distribution in cement-based mortars using active microwave thermography. Materials and Structures, 2016. 49(12): p. 5051––5065.
45.Choi Y. and Yuan R. L. Experimental relationship between splitting tensile strength and compressive strength of GFRC and PFRC. Cement and Concrete Research, 2005. 35(8): p. 1587––1591.
84

Issue № 3 (39), 2018 |
ISSN 2542-0526 |
TECHNOLOGY AND ORGANIZATION OF CONSTRUCTION
UDC 624.05
Z. R. Mukhametzyanov1, R. V. Razyapov2
MECHANISM OF DEVELOPMENT OF ORGANIZATIONAL SOLUTIONS
BASED ON A TECHNOLOGICAL INTERACTION
BETWEEN CONSTRUCTION WORKS AND PROCESSES
Ufa State Oil Technical University
Russia, Ufa, tel.: 79177803505, e-mail: zinur-1966@mail.ru
1PhD in Engineering, Assoc. Prof. of the Dept. of Technological Machines and Equipment
2Lecturer of the Dept. of Roads and Construction Technology
Statement of the problem. The aim is to address the question of improving the reliability of organizational and technological decisions in the construction of objects based on qualitative and quantitative assessments of the technological dependencies between jobs.
Results. Research of technologies of construction of buildings and structures allowed one to identify a model of technology parameters such as qualitative and quantitative estimation of technological relationships, time-domain performance of works for making organizational decisions. Based on a model technology of construction, a proposed method of making organizational decisions is proposed in order to form the parameters of the construction object for each planning period using the identified characteristics of the model of technology of construction.
Conclusions. Development of organizational solutions based on the characteristics of technological links between works improves the reliability of organizational and technological solutions in the construction of different objects.
Keywords: organizational and technological decisions, qualitative assessment, quantitative assessment, object construction technology, planning period, organizational decision model, power type resources.
Introduction. Complex, labour-intensive works, probabilistic nature of construction, variety of ways of organizing them leads to a great number of optimization tasks to handle to find and utilize differeny types of resources for enhancing the efficiency of various buildings and structures [2]. Nevertheless the methods associated with organizational and technological decisions (OTD) are still prioritized [1, 10].
Presently the base for forming OTD is still the approach relying on the principles of systemic technology of construction [3, 13]. As aprt of the research field Russian specialists have been
© Mukhametzyanov Z. R., Razyapov R. V., 2018
85

Russian Journal of Building Construction and Architecture
developing different methods of improving the efficiency of OTD. They are the methods of classifying organizational and technological decisions into different groups based on their reliability [4], development of methodological bases of designing organizational and technological processes to ensure systematic and technological connection between functional subsystems and informational and analytical tasks in informational and computational environment [6], implementation of the formalization of reverse connections between functional systems [14], methods of improving the reliability considering the risks in designing organization, technology and management of construction under conditions of uncertainty [5], “the project approach” that takes into account market foundations of construction design [7], development of projects of organizing construction by means of integration of a flow with the principles of complex mechanization and use of cyber systems of construction management [8, 9, 14, 15]. Researchers abroad point out the methods based on the strategy of the management of a buffer [17], design of an integrated metrics for quantitative evaluation of the stability of interdependent systems [19], safety of processes in construction [20—22].
But despite a high degree of development of the problem, the general issue of improving the reliability which is associated with considering the features and character of the interaction of organizational and technological factors emerging in construction of buildings and structures of different types still remains insufficiently investigated. This paper aims to address the problem and set forth new mechanisms of making a system of OTD.
1. Studies of the technology of construction of buildings and structures. Construction of objects of any degree of complexity involves attracting a large number of enterprises, use of a great amount of materials, structures, products with different construction and technological properties. Construction of any objects is associated with a great deal of technological processes and operations with specific characteristics and parameters 16].
Therefore it is necessary to first of all consider the character of the interaction of the interconnected technological processes within a technology of construction of buildings and structures that make up OTD, their internal interconnection and intertwining in space and time for their function in the construction of objects of different types and consideration of this interaction of different organization decisions.
According to the previous studies of OTD, the following results were obtained:
–– the processes or works are mutually connected at the start and end, i.e. in the interaction technological connections between the starts and technological connections between the ends of associated processes or works are involved;
86
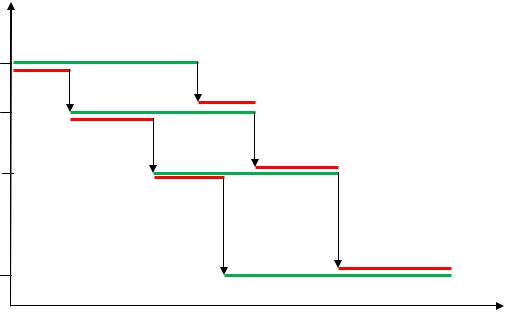
Issue № 3 (39), 2018 |
ISSN 2542-0526 |
–– the qualitative and quantitative evaluations of technological dependencies that determine the character of the interaction between associated processes and works are identified.
In Figure there is a graphic interpretation as a linear diagram of technological dependencies between associated works performed during the construction of an object.
In Figure the vertical arrows depict technological connections at the start and end of works and their technological sequence is identified. Dots identify quantitative aspects of the technological dependencies that represent minimal delays of the end of subsequent work from the previous one and a minimum acceleration of the start of the previous work in relation to the start of subsequent work. A minimal amount of work that are used to determine a qualitative minimal delay and acceleration are identified by means of a method developed by the author [11, 18].
The identified quantitative and qualitative evaluations of technological dependencies are restrictive for determining times of works with a quantitative evaluation giving no specification of exact times of works. These can take different values for each type of works within a time range and be specified in the development of organizational and other decisions for each specific work.
Work
1
2
3
…
n
Amount of
Fig. Linear diagram of technological dependencies of associated works
2. Order of the distribution of amount of construction processes in the planned periods of time. Distribution of the amount of construction processes in the planned periods is based on a construction task at hand while planning technical and economic aspects and involves a work plan as well as that of financial and technical provision.
87
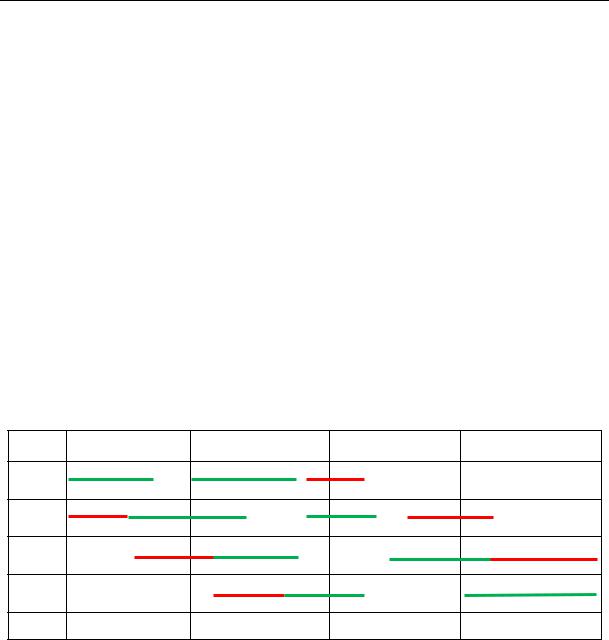
Russian Journal of Building Construction and Architecture
The result of the task is a set of the amount of processes and works at each planned period, which is the objective of industrial activities of an individual construction enterprise (Table). The development of a work plan in planned periods is based on the above method of modelling technological dependencies based on the developed quantitative and qualitative evaluations of technological dependencies between associated processes.
The distribution of the amount of construction and assembly works (CAW) in planned periods starts with determining a time range of the final work 4 that is restricted by a planned period of the end of the construction of an object and a minimal delay in the start of the work from the start of the work 3. Then similarly the remaining works are distributed with a necessary time restriction based on the connection between the start of the previous and end of the subsequent one based on a minimal amount. It is worth noting that amounts of CAW might vary in each planned period. They depend on a plan of technical and economic aspects in a planned period that eventually lead to further industrial activities of an enterprise.
Таble
Example of the distribution of the amount of construction processes in planned periods
Work
1
2
3
4
1 planned period |
2 planned period |
3 planned period |
4 planned period |
3. Formation of organizational decisions. Development of organizational decisions is associated with determining the intensity, time of works, duties, start and end of construction processes and works, optimal range of combining technologically associated processes and works, etc. But their development first of all depends on available labour resources in each planned period. The first condition (restriction) that is necessary in calculating the required labour resources for a planned amount of works in a certain planned period is considering a quantitative and qualitative characteristics of technologically associated works (processes) [12]. Planning of a necessary amount of works j is possible if
1 |
|
|
Vj k ,i, Vj k ,i VminН |
j, j k ,i ( 1,2, ), |
(1) |
1
88
Issue № 3 (39), 2018 |
ISSN 2542-0526 |
where Vj−k, i, is the planned amount of the previous work (j−k) on the object i in the planned
1
period that the work j is associated with technologically Vj k ,i is the amount of the previ-
1
ous work (j−k) on the object I performed (planned) in the previous planned periods; VminН j, j k,i
is a minimal amount that is necessary (or planned) on the previous work (j−k) for planning of a unit of the amount of work j.
The idea of the restriction is that while planning technologically associated works at a certain time period it is essential that it is possible while performing or planning the amount of work for subsequent work which is more technologically possible than the previous work. Therefore the end or start of the work j can be planned in a certain planned time period according to the character of the connections between the processes of the stage if the minimal amount of the previous work (j−k) is provided.
The second condition is the restriction of the planned amount of work j in the planned time period . According to this restriction, a planned amount of work should not be more than it is possible to carry out at a maximum workload with the following resources:
Vi, j, Vmaxi, j, , |
(2) |
where Vmax i,j,τ is the amount of work that can be performed at a maximum workload for the labour force.
The third condition is the restriction or required support of the planned amount of work with financial resources:
n |
m |
|
M , n , jVjпл,i, , |
(3) |
|
i 1 |
j 1 |
|
where M , is the amount of financial resources of the type µ that can be incurred by a construction enterprise at the time ; n , j is a norm of material consumption per a unit of the work j; Vjпл,i, is the planned amount of work on an object in a certain planned period in natural
measurements. Then the necessary resources that are required for the work planned in a certain time period are given by the following formula:
m |
n |
ед пл |
|
|
|
Rh |
Cj Vj,i, |
Rрасчh |
, |
(4) |
|
h |
|||||
j 1 |
i 1 |
g |
|
|
|
where Cедj is the cost of a unit of work; Vjпл,i, |
is the planned amount of work on an object in a |
||||
certain time period in natural measurements; i is the number of an object, i = 1, 2, … n; |
j is |
89

Russian Journal of Building Construction and Architecture
the number of work or process in a planned period, j = 1, 2, … m; is the number of a planned period, 1,2, ; gh is the daily cost of the labour resources h.
The resulting ratio indicates the amount of the required resources for implementing the entire amount of works in a certain time period as part of technical and economic aspects as specified by a construction enterprise. But note once more that construction production is a probabilistic system that is influenced by a variety of destabilizing factors. For the case at hand, i.e. for balanced performance of the planned amount of works within a planned period, it is necessary that there is a constant workload established for every professional involved within the entire time period. For that, the entire amount of works should be planned so that at any moment there is the following amount of work being handled:
Rh (t) Rh , |
(5) |
where Rh(t) is the amount of labour resources that can be involved at the moment t: |
|
m n |
CедV |
(t) |
|
|
Rh (t) |
j max j |
|
, |
(6) |
h h |
|
|||
j 1 i 1 |
g k |
|
|
|
where kh is a planned coefficient of improving the productivity of the professionals h. Conclusions. The model suggested for making organizational decisions in the construction of objects is advantageous in the way that within its parameter acceptable decisions can be specified, which contributes to the variety of possible decisions and thus balances them.
The method set forth for making organizational decisions in the construiction of objects allows an optimal calendar plan of OTD to be specified due to the following factors:
1)organizational decisions are identified in each planned period individually, which allows one to consider all the restrictions, reduce a range of decisions and pick one and thus improve their reliability;
2)organizational decisions are determined with no preliminary distribution of labour resources, no conditions of balance and continuity of OTD, which allows the start and end of works to vary with no disruptions to technological connections.
References
1. Abdullaev G. I. Osnovnye napravleniya povysheniya nadezhnosti stroitel'nykh protsessov [The main directions of improving the reliability of construction processes]. Inzhenerno-stroitel'nyi zhurnal, 2010, no. 4, pp. 59—60.
2. Votyakova O. N. Optimizatsiya organizatsionno-tekhnologicheskikh reshenii rekonstruktsii linii elektroperedach [Optimization of organizational and technological solutions of power lines reconstruction].
Promyshlennoe i grazhdanskoe stroitel'stvo, 2015, no. 2, pp. 43—45.
90