
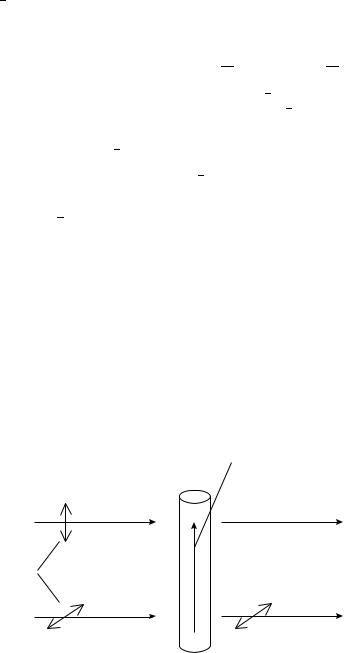
9. Liquid crystals with XDY groups |
463 |
(2) Guest Host Cell: A full coloured RGB (red, green, blue colours) display can be realized in two principle ways; in both cases one pixel consists of three subpixels containing the RGB colour. In the first situation a dye (guest) is dissolved in the nematic compound (host); the display appears coloured if the polarization of the incoming light is parallel to the dipole transition moment of the dye, achieved by mounting a polarizer in front of the display. The dye itself has to orient with the solvent the nematic phase and must not destroy the nematic ordering, therefore the guest should be of anisotropic shape like the host molecules too (Figure 45). Basic investigations on guest host systems were presented previously29,30,130,131. Figure 46 shows the fundamental guest host cell suggested by Heilmeier and Zannoni132. In the field-off state the polarization of the incoming light and the transition moment are parallel, absorption takes place and the display appears coloured. In the on-state the guest host mixture orients parallel to the electric field, the transition moment and the light polarization are perpendicular, the display occurs colourless. An outstanding review about the various guest host displays was written by Uchida and Wada133; Ivashenko and Rumyantsev134 summarized the accessible dye materials. The main disadvantages of the guest-host display are the photobleaching of the dye, the low contrast ratio (ca 5 10) and the large fabrication costs. Therefore, with a few exceptions (Table 18) this display has not been commercialized. The second approach follows the classical TV cathode-ray approach: RGB filters in front of the passive or active matrix display allow for the aspired colour mixture. Two different display types are commercially successful: first the simple (passive) matrix (double) super-twisted nematic display (STN-LCD), and the active matrix thin-film transistor display (TFT-LCD).
(3) STN-LCD: To overcome the multiplexing limit due to the poor threshold characteristics of the simple TN display, the super-twisted nematic display was established under consideration of the birefringence properties of the nematic phase135. The best twist angle of 270° in the cell in combination with a low driving voltage and an optimized multiplexing can be achieved by doping the nematic phase with a small amount of chiral agents, which introduce a rightor left-handed helical arrangement as in the cholesteric phase136. The combination of two single STN cells with opposite-handed helices improves the transmission over the whole visible spectral range (Figure 47).
(4) Passive Matrix LCD: The realization of high resolution displays, or even the more simple alphanumeric displays, requires the independent addressing of each pixel. Different matrix techniques have been established; common characteristics are the rectangular
Transition moment
Absorption
Polarization of incoming light
Transmission
Dye molecule
FIGURE 45. Absorption depends on the angle between transition moment and polarization
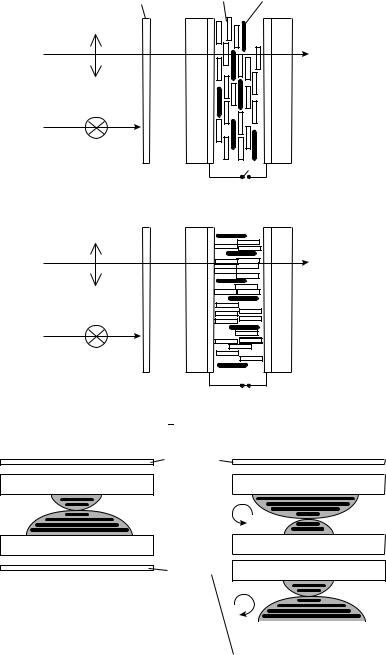
464 |
T. Hanemann and W. Haase |
|
|||
|
Polarizer |
nematic lc |
dye |
||
|
|
|
|
|
|
|
|
|
|
|
|
coloured
Incoming light
off-state
colourless
Incoming light
on-state
FIGURE 46. Schematic drawing of the guest host cell138
Polarizer
STN
STN
Polarizer
STN
|
|
|
|
STN |
D-STN |
FIGURE 47. Schematic drawing of STN-LCD and D-STN-LCD
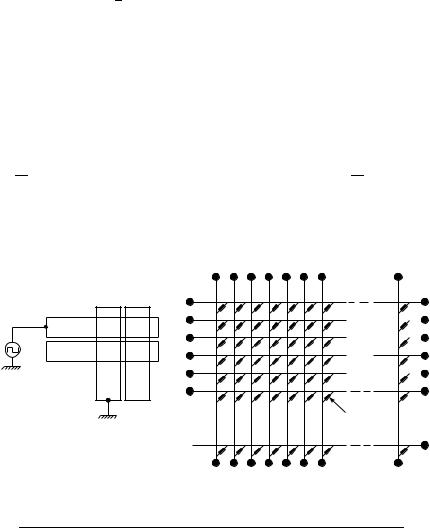
9. Liquid crystals with XDY groups |
465 |
arrangement of striped transparent vertical and horizontal electrodes on the inner surface of the top and bottom glass substrates. The unequivocal advantage of the matrix techniqe is the reduced number of necessary electric contacts (M ð N, Figure 48). This configuration is often called the X Y DOT matrix element and allows for the presentation of any characteristic pattern136. One can also depict the principle arrangement of the electrodes and the electric paths within a liquid crystal display matrix element. Without an electric field all pixels appear dark, but if voltage is applied to the electrode X1 (column) and electrode Y1 (row) is grounded, the cell P11 switches to the bright state. For all other pixels the circuit remains open and therefore they stay in the dark state. Besides the mentioned advantages of reduced bonding, this mechanism limits the number of rows and columns and, as a result, the accessible display size, the resolution and the image quality. With increasing number of columns and rows crosstalk occurs, i.e. unaddressed pixels become bright and the aspired contrast ratio decreases dramatically.
(5) Active Matrix LCD (TFT-Technique): The current technique to overcome the above mentioned problems of the passive matrix display is the direct addressing of each pixel independently of each other. A thin film transistor (TFT) is located at one corner of each pixel at the intersection of the scanning and signal electrode buses and acts as a switch (Figures 49 and 50). The source and drain electrodes of the TFT are connected to the signal electrode bus and pixel electrode; the gate electrode is attached to the scanning electrode bus, so any interaction between different pixel elements are excluded. Full coloured TFT displays can be realized using RGB filters. Table 20 lists the present
1 |
(x−1) x (x+1) |
M |
|
|
|
x2 |
1 |
|
|
Y1 |
P11 |
P12 |
(y−1) |
|
v |
|
|
|
||
Y2 |
P21 |
P22 |
y |
||
|
|||||
|
|
|
|
(y+1) |
Pixel
N
FIGURE 48. Matrix addressing and electric circuit diagram
TABLE 20. Characteristics of Sharp 1400 colour TFT module
Item |
Characteristics |
|
|
Display dimension |
36.0 cm diagonal |
DOT configuration, pitch |
640 ð 480 ð RGB (VGA or better), 0.150 ð 0.450 mm |
Displayable colours |
16 million |
Pixel arrangement |
RGB stripe configuration |
Contrast ratio |
>100:1 (vertical in panel) |
Viewing angle |
vertical and horizontal: 80 (š40°) |
Module dimensions |
384(H) ð 285(V) ð 25(D) mm |
Module weight |
1.8 kg |
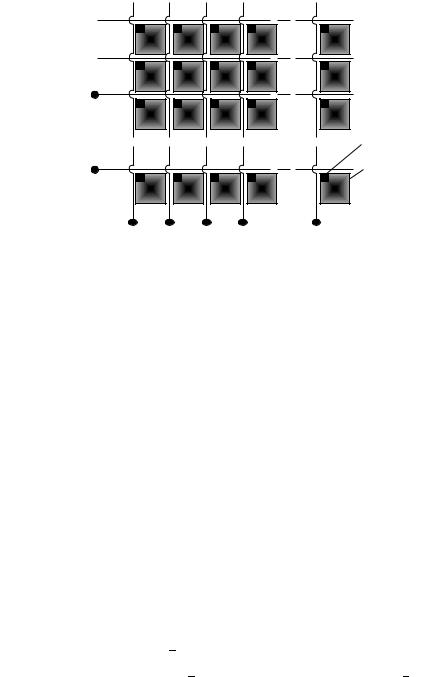
466 |
T. Hanemann and W. Haase |
Scanning electrode bus
Y1 |
Y2 |
YM |
X1
X2
|
TFT |
XN |
Pixel |
Signal electrode bus
FIGURE 49. Schematic drawing of the active matrix TFT display
FIGURE 50. Cross section through a single TFT pixel
state of the art for a modern lc display for multimedia applications (Sharp Electronics)125. For the beginning of the next millenium different display characteristics are planned, depending on the various kinds of displays and the aspired applications (Table 21)137.
B. Spatial Light Modulators
The realization of spatial light modulators for application in telecommunication has become of interest since the beginning of this decade. There is also much activity in developing flat panel devices based on ferroelectric or antiferroelectric LC’s. Both are about to be introduced into the market. In principle, using nematic and ferroelectric LC’s (FLC) enables many different devices to be constructed, e.g. as OASLM (optical addressed spatial light modulators), switches, shutters etc. It turned out that the nematic phase is not fast enough, therefore FLC devices are really good candidates for rapid effects. Because the linear electro-optical response of the electroclinic and DHF (deformed helicol ferroelectric) effects are compatible with the linear change in the resistivity of photoconductive layers, the sensitivity of OASLM based on these effects is much higher than in nematic liquid crystals. One great advantage is that the electroclinic and DHF effect allow the grey scale; this is not the case for SSFLC (surface stabilized ferroelectric liquid crystals) switching. OASLM consists of photosensitive layers of polycrystalline ZnSe with a thickness around 10 15 m on one side, and amorphous hydrogenized ˛- Si:H in the regime of intrinsic photoconductivity was used. The ZnSe films exhibited nice spatial resolution in a thickness of 1.3 2.0 m for the electroclinic mode and 5.5 6.0 m

TABLE 21. Display products in 2000 (AM: active matrix, PM: passive matrix)
Characteristic |
AM-LCD for office |
PM-LCD for office |
AM-LCD for HDTV |
AM-LCD for mobile |
PM-LCD for mobile |
|||||||||||||||||
|
|
|
|
machines |
|
|
|
machines |
|
|
|
|
|
|
equipment |
|
|
equipment |
||||
Screen size(00 ) |
17 |
|
|
|
10 |
|
|
|
30 |
|
|
|
6 |
|
|
6 |
|
|
|
|||
No. of pixels |
1280 ð 1024 |
640 ð 480 |
1920 ð 1035 |
320 ð 240 |
320 ð 240 |
|||||||||||||||||
Pixel pitch (mm) |
0.27 |
|
|
0.30 |
|
|
0.345 ð 0.36 |
0.375 |
|
0.375 |
|
|||||||||||
Display colour |
full |
full |
full |
full |
|
full |
|
|
||||||||||||||
Contrast |
100:1 |
|
30:1 |
|
|
100:1 |
|
100:1 |
|
40:1 |
|
|
|
|||||||||
Viewing angles: Up/down |
90° Cone |
90° Cone |
90° Cone |
120° |
|
|
90° Cone |
|||||||||||||||
Left/right |
(10:1) |
(10:1) |
(20:1) |
(10:1) |
|
|
|
|
|
|||||||||||||
Response time (ms) |
30 |
|
|
|
50 |
|
|
|
20 |
|
|
|
20 |
|
|
30 |
|
|
|
|||
Screen luminance (Cd/m2) |
150 |
|
|
100 |
|
|
200 |
|
|
300 |
|
|
300 |
|
|
|
||||||
Operating temp. range (°C) |
0 |
|
50 |
|
0 |
|
50 |
|
0 |
|
50 |
|
20 |
|
80 |
30 |
|
|
80 |
|||
|
|
|
|
|
|
|||||||||||||||||
Storage temp. range (°C) |
20 |
|
60 |
20 |
|
60 |
20 |
|
60 |
40 |
|
90 |
40 |
|
|
90 |
||||||
|
|
|
|
|
|
|||||||||||||||||
Vibration (m/s2) |
15 |
|
|
|
15 |
|
|
|
15 |
|
|
|
40 |
|
|
40 |
|
|
|
|||
Shock (m/s2) |
300 |
|
|
300 |
|
|
300 |
|
|
1000 |
|
1000 |
|
|
||||||||
Thickness (mm) |
10 |
|
|
|
6 |
|
|
|
|
25 |
|
|
|
6 |
|
|
6 |
|
|
|
||
Weight (g) |
1000 |
|
300 |
|
|
7000 |
|
|
|
|
|
|
|
|
||||||||
Power consumption (W) |
15 |
|
|
|
2 |
|
|
|
|
50 |
|
|
|
3 |
|
|
3 |
|
|
|
||
|
|
|
|
|
|
|
|
|
|
|
|
|
|
|
|
|
|
|
|
|
|
|
467
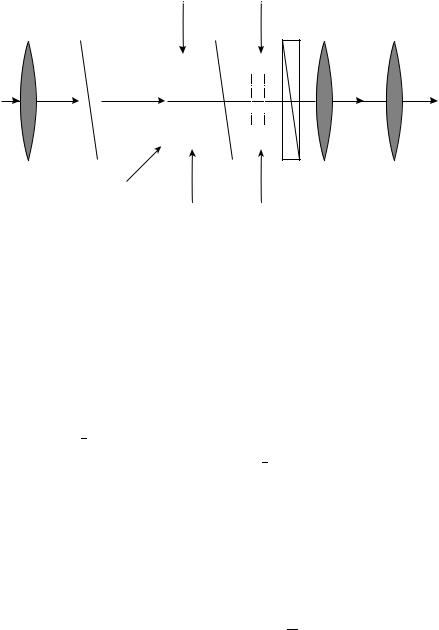
468 |
|
T. Hanemann and W. Haase |
|||||||||
|
|
OASLM |
Shutter |
||||||||
|
P1 |
|
|
|
|||||||
|
|
|
|
|
|
|
|
P2 |
|
|
P3 |
|
|
|
|
|
|
|
|
|
|
|
|
|
|
|
|
|
|
|
|
|
|
|
|
|
|
|
|
|
|
|
|
|
|
|
|
|
|
|
|
|
|
|
|
|
|
|
|
|
|
|
|
|
|
|
|
|
|
|
|
|
|
|
|
|
|
|
|
|
|
|
|
|
|
|
|
|
|
|
|
|
|
|
|
|
|
|
|
|
|
|
|
|
|
|
|
|
|
|
|
|
|
|
|
|
|
|
|
|
|
|
|
|
|
|
|
|
|
|
|
|
|
|
|
|
|
|
|
|
|
|
|
|
|
|
|
|
|
|
|
|
|
|
|
|
|
|
|
|
|
|
|
|
|
|
|
PC
FLC2 FLC1
FIGURE 51. The optical scheme of a goggle with fast shutter FLC2
for the DHF mode. Also, amorphous ˛-Si:H showed good effects but some problems still arise in the separation of the writing and reading light beams. The ferroelectric samples used for such experiments are mixtures with low rotation viscosities. To prepare good devices, a good surface preparation procedure is essential. OASLM can be used, e.g., as safety goggles or in general to modulate the light. One scheme is presented in Figure 51: PC is the photoconductive layer, P1, P2 and P3 are polarizers, FLC1 and FLC2 are active cells. The best version for FLC2 is a tilt angle of 22.5°. This goggle is now in a very advanced stage138. An excellent overview covering this new field of application was presented by Efron139.
VII. REFERENCES
1. J. P. van Meter, in Chemistry of Functional Groups, Supplement A (Ed. S. Patai), Wiley, London, 1977, pp. 93 129.
2. A. J. Leadbetter, in The Molecular Physics of Liquid Crystals (Eds. G. R. Luckhurst and
G. W. Gray), Academic Press, London, 1979, pp. 285 316.
3.G. W. Gray and J. Goodby, Smectic Liquid Crystals, Leonard Hill, Glasgow, 1984.
4.D. Demus, Fl¨ussige Kristallen in Tabellen, VEB Deutscher Verlag fur¨ Grundstoffindustrie, Leipzig, 1974.
5.D. Demus, Fl¨ussige Kristallen in Tabellen II, VEB Deutscher Verlag fur¨ Grundstoffindustrie, Leipzig, 1984.
6.M. J. S. Dewar and R. M. Riddle, J. Am. Chem. Soc., 97, 6658 (1975).
7.M. J. S. Dewar and A. C. Griffin, J. Am. Chem. Soc., 97, 6662 (1975).
8.I. Haller, H. A. Huggins, H. R. Lilienthal and T. R. McGuire, J. Phys. Chem., 77, 950 (1973).
9.S. Sinton and A. Pines, Chem. Phys. Lett., 76, 263 (1980).
10.Y. Iwakabe, M. Hara, K. Kondo, K. Togichi, A. Mukoh, A. Yamada, A. F. Garito and H. Sasabe,
Jpn. J. Appl. Phys., 10, 2542 (1991).
11.J. Frommer, Liq. Crystals Today, 3, 1 (1993).
12.D. Demus and L. Richter, Textures of Liquid Crystals, Verlag Chemie, Leipzig, 1978.
13. G. W. Gray and J. W. G. Goodby, Smectic Liquid Crystals Textures and Structures, Leonard Hill, Glasgow and London, 1984.
14.I. H. Ibrahim and W. Haase, J. Phys. Colloq. C, 40, 164 (1979).
15. I. Bikchantaev, Y. Galyametdinov, A. Prosvirin, K. Griesar, E. A. Soto Bustamante and W. Haase, Liq. Cryst., 16, 231 (1994).

9. Liquid crystals with XDY groups |
469 |
16.G. Sigaud, F. Hardouin, M. F. Achard and H. Gasparoux, J. Phys. Colloq. C, 40, 356 (1979).
17.B. I. Ostrovskii, A. I. Pavluchenko, V. F. Petrov and M. A. Saidachmetov, Liq. Cryst., 5, 513 (1989).
18.P. S. Pershan and J. Prost, J. Phys. Lett., 27, 40 (1979).
19.F. Dowell, Phys. Rev. A, 28, 3526 (1983).
20.H. Matsuzaki and Y. Matsunaga, Liq. Cryst., 14, 105 (1993).
21.H. Gasparoux, in Liquid Crystals of Oneand Two-Dimensional Order (Eds. W. Helfrich and
G.Heppke), Springer, Berlin, 1980, p. 373.
22.G. Khanarian (Ed.), Nonlinear Optical Properties of Organic Materials, Vol. III, SPIE Proc.,
1337, 144 (1990).
23.R. A. Hann and D. Bloor (Eds.), Organic Materials for Nonlinear Optics, OMNO Proc., 2, 1 (1990).
24.G. Roosen (Ed.), Nonlinear Optical Effects in Organic Molecules and Polymers, Theory Measurements and Devices, SPIE Proc., 1047, 2 (1988).
25.D. J. Williams, Angew. Chem., 96, 637 (1984).
26.C. Fouquey, J.-M. Lehn and J. Malthete, J. Chem. Soc., Chem. Commun., 1424 (1987).
27.T. Weyrauch, T. Hanemann, E. A. Soto Bustamante and W. Haase, SPIE Proc., 19 24.07.1992, San Diego, CA, USA.
28.T. Hanemann, E. A. Soto Bustamante, T. Weyrauch and W. Haase, Liq. Cryst., 14, 635 (1993).
29.T. Hanemann and W. Haase, Mol. Cryst. Liq. Cryst., 231, 119 (1993).
30.T. Hanemann and W. Haase, Ber. Bunsenges. Phys. Chem., 98, 596 (1994).
31.T. Hanemann, C. R. Moylan and R. J. Twieg, Liq. Cryst., in preparation.
32.F. Jede, A. W. Stratmann and B. Schrader, Mol. Cryst. Liq. Cryst., 140, 297 (1986).
33.I. H. Ibrahim, PhD Thesis, Darmstadt, FRG, 1980.
34.T. Hanemann, PhD Thesis, Darmstadt, FRG, 1993.
35.T. Hanemann and W. Haase, Polymer Preprints, 35(2), 254 (1994).
36.T. Hanemann, C. Noel¨ and W. Haase, Adv. Mater., 7, 465 (1995).
37.C. Mertesdorf, H. Ringsdorf and J. Stumpe, Liq. Cryst., 9, 337 (1991).
38.S. Yitzchaik, G. Berkovic, and V. Krongauz, Adv. Mater., 2, 33 (1990).
39.F. P. Shvartsman and V. A. Krongauz, Nature, 302, 608 (1984).
40.M. H. Wann and G. S. Harbison, J. Am. Chem. Soc., 111, 7273 (1989).
41.P. Keller, A. Zann, J. C. Dubois and J. Billard, in Liquid Crystals of Oneand Two-Dimensional Order (Eds. W. Helfrich and G. Heppke), Springer, Berlin, 1980, p. 57.
42.J. W. Goodby, G. W. Gray, A. J. Leadbetter and M. A. Mazid, in Liquid Crystals of Oneand Two-Dimensional Order (Eds. W. Helfrich and G. Heppke), Springer, Berlin, 1980, p. 3.
43.G. W. Gray, Phil. Trans. R. Soc. Lond. A, 309, 77 (1983).
44.G. Heppke, P. Kleineberg and D. Lotzsch,¨ Liq. Cryst., 14, 67 (1993).
45.S. Chandrasekhar, in Advances in Liquid Crystals, Vol. 5 (Ed. G. H. Brown), Academic Press, New York, 1982, pp. 47 78.
46.H. Stegemeyer and K. Bergmann, in Liquid Crystals of Oneand Two-Dimensional Order (Eds.
W.Helfrich and G. Heppke), Springer, Berlin, 1980, p. 161.
47.B. McArdle (Ed.), Side Chain Liquid Crystal Polymers, Blackie, Glasgow, 1989, p. 448.
48.A. Ciferri, (Ed.), Liquid Crystallinity in Polymers, VCH Publishers, N.Y., 1991, p. 438.
49.V. Percec and C. Pugh, in Side Chain Liquid Crystal Polymers (Ed. C. B. McArdle), Blackie, Glasgow, 1989, p. 30.
50.A. Sirigu, Liq. Cryst., 14, 15 (1993).
51.R. Zentel and H. Ringsdorf, Makromol. Chem. Rapid Commun., 5, 393 (1984).
52.B. Gallot, F. Monnet and S. He, Liq. Cryst., 19, 901 (1995).
53.S. Shilov, B. Volchek, V. Zuev and S. Skorokhodov, Macromol. Chem. Phys., 195, 865 (1994).
54.A. Sirigu, in Liquid Crystallinity in Polymers (Ed. A. Ciferri), VCH Publishers, New York, 1991, p. 261.
55.L. Noirez, P. Keller and P. Kotton, Liq. Cryst., 18, 129 (1995).
56.M. Niemann and H. Ritter, Makromol. Chem., 195, 1169 (1993).
57.S. Ishibashi, K. Tokahashi, S. Ishizawa and F. Yamamoto, Ferroelectrics, 148, 277 (1993).
58.M. V. Kozlovsky, E. A. Soto Bustamante and W. Haase, Ferroelectrics, in press.
59.M. V. Kozlovsky and L. A. Beresnev, Phase Transitions, 40, 129 (1992).
60.S. Esselin, C. Noel, G. Decobert and J. C. Dubois, Mol. Cryst. Liq. Cryst., 155, 371 (1988).
61.V. Percec and B. Hahn, J. Polym. Sci., Polym. Chem. Ed., 27, 2367 (1989).

470 |
T. Hanemann and W. Haase |
62.G. Kostromin, V. V. Sinitzyn, R. V. Talroze and V. P. Shibaev, Makromol. Chem., Rapid. Commun., 3, 809 (1982).
63.H. Poths, R. Zentel, S. U. Vallerien and F. Kremer, Mol. Cryst. Liq. Cryst., 203, 101 (1991).
64.R. Zentel and M. Brehmer, Adv. Mater., 6, 598 (1994).
65.P. Keller, R. Shao, D. M. Walba and M. Brunet, reported at the Vth Int. Conf. on Ferroelectric Liquid Crystals, July 23 27, 1995, Cambridge, UK.
66.S. Uchida, K. Morita, K. Miyoshi and K. Kawasaki, Mol. Cryst. Liq. Cryst., 155, 93 (1988).
67.K. Kawasaki, H. Kidera, T. Sekiya and S. Hachiya, Ferroelectrics, 148, 233 (1993).
68.P. Espinet, M. A. Esteruelas, L. A. Oro, J. L. Serrano and E. Sola, Coord. Chem. Rev., 117, 215 (1992).
69.A. Hudson and P. M. Maitlis, Chem. Rev. 93, 861 (1993).
70.M. Giroud-Godquin and P. M. Maitlis, Angew. Chem., 103, 370 (1991).
71.J. Malthete` and J. Billard, Mol. Cryst. Liq. Cryst., 34, 117 (1976).
72.D. Vorlander,¨ Ber. Dtsch. Chem. Ges., 43, 3120 (1910).
73.D. Vorlander,¨ Z. Phys. Chem. Stoechiom. Verwandschaftsl., 105, 211 (1923).
74.W. Bruce, D. A. Dunmur, E. Lalinde, P. M. Maitlis and P. Styring, Nature, 323, 791 (1986).
75.J. Bulkin, R. K. Rose and A. Santoro, Mol. Cryst. Liq. Cryst., 43, 53 (1977).
76.M. Giroud-Godquin and J. Billard, Mol. Cryst. Liq. Cryst., 66, 287 (1983).
77.M. Giroud-Godquin and J. Billard, Mol. Cryst. Liq. Cryst., 97, 287 (1985).
78.V. Salas Reyes, G. Soto-Garrido, C. Aguilera, K. Griesar, M. Athanassopoulou, H. Finkelmann and W. Haase, Mol. Materials, 3, 321 (1994).
79.M. Giroud-Godquin, Ann. Phys., 3, 147 (1978).
80.M. Giroud-Godquin, A. Nazzal and U. T. Muller¨-Westerhoff, Mol. Cryst. Liq. Cryst., 56, 225 (1980).
81.M. Cortrait, J. Gaultier, C. Polycarpe, A. M. Giroud and U. T. Muller¨-Westerhoff, Acta Crystallogr., C39, 833 (1983).
82. H. Adams, N. A. Bailey, D. W. Bruce, R. Dhillon, D. A. Dunmur, S. E. Hunt, E. Lalinde,
A.A. Maggs, R. Orr, P. Styring, M. S. Wragg and P. M. Mailis, Polyhedron 7, 1861, (1988).
83.J. Barbera, P. Espinet, E. Lalinde, M. Marcos and J. L. Serrano, Liq. Cryst., 2, 833 (1987).
84.K. Griesar, Y. Galyametdinov, M. A. Athanassopoulou, I. Ovchinnikov and W. Haase, Adv. Mater., 6, 381 (1994).
85.P. Eastman, M. L. Horng, B. Freiha and K. W. Sheu, Liq. Cryst., 2, 223 (1987).
86.Y. Galyametdinov, G. Ivanova, K. Griesar, A. Prosvirin, I. Ovchinnikov and W. Haase, Adv. Mater., 4, 739 (1992).
87.Y. Galyametdinov, M. A. Athanassopoulou, K. Griesar, O. Kharitonova, E. A. Soto Bustamante,
L.Tinchurina, I. Ovchinnikov and W. Haase, Chem. Mater., 8, 922 (1996).
88.G. A. Jeffrey, Acc. Chem. Res., 19, 168 (1986).
89.H. A. Van Doren, R. Van der Geest, C. F. de Ruijter, R. M. Kellogg and H. Wynberg, Liq. Cryst., 8, 109 (1990).
90.G. A. Jeffrey and L. M. Wingert, Liq. Cryst., 12, 179 (1992).
91.E. Fischer and B. Helferich, Ann. Chem., 383, 68 (1911).
92.R. Noller and W. C. Rockwell, J. Am Chem. Soc., 60, 2076 (1938).
93.J. Koscielak and R. W. Jeanloz, Carbohydr. Res., 5, 220 (1967).
94.D. C. Carter, J. R. Ruble and G. A. Jeffrey, Carbohydr. Res., 102, 59 (1982).
95.R. G. Zimmermann, G. B. Jameson, R. G. Weiss and G. Demailly, Mol. Cryst. Liq. Cryst. (Lett.), 1, 183 (1985).
96.M. A. Marcus and P. L. Finn, Mol. Cryst. Liq. Cryst. (Lett.), 2, 159 (1985).
97.J. W. Goodby, Mol. Cryst. Liq. Cryst., 110, 205 (1984).
98.D. Baeyens-Volant, P. Cuvelier, R. Fornasier, E. Szalai and C. David, Mol. Cryst. Liq. Cryst., 128, 277 (1985).
99.D. Baeyens-Volant, R. Fornasier, E. Szalai and C. David, Mol. Cryst. Liq. Cryst., 135, 93 (1986).
100.B. Pfannemueller, W. Welte, E. Chin and J. W. Goodby, Liq. Cryst., 1, 357 (1986).
101.J. W. Goodby, M. A. Marcus, E. Chin, P. L. Finn and B. Pfannemueller, Liq. Cryst., 3, 1569 (1988).
102.A. Eckert, B. Kohne and K. Praefcke, Z. Naturforsch., 43b, 878 (1988).
103.K. Praefcke, A.-M. Levelut, B. Kohne and A. Eckert, Liq. Cryst., 6, 363 (1989).
104.T. Itoh, A. Takada, T. Fukuda, T. Miyamoto, Y. Yakoh and J. Watanabe, Liq. Cryst., 9, 221 (1991).

9. Liquid crystals with XDY groups |
471 |
105.V. Vill and J. Thiem, Liq. Cryst., 9, 451 (1991).
106.V. Vill, T. Boecker, J. Thiem and F. Fischer, Liq. Cryst., 6, 349 (1989).
107.H. A. van Doren, R. van der Geest, R. M. Kellogg and H. Wynberg, Carbohydr. Res., 194, 71 (1989).
108.H. A. van Doren and L. M. Wingert, Mol. Cryst. Liq. Cryst., 198, 381 (1989).
109.G. A. Jeffrey, Mol. Cryst. Liq. Cryst., 185, 209 (1990).
110.F. W. Lichtenthaler, R. Klimesch, V. Mueller and M. Kunz, Ann. Chem., 975 (1993).
111.F. W. Lichtenthaler, D. Martin, T. Weber and H. Schiweck, Ann. Chem., 967 (1993).
112.T. Hanemann, E. Schumacher, F. W. Lichtenthaler and W. Haase, Liq. Cryst., in press.
113.D. Blunk, K. Praefcke and G. Legler, Liq. Cryst., 18, 149 (1995).
114.M. M. Murza, Z. H. Kuvatov, O. A. Scaldin, Y. A. Lebedev and M. G. Safarov, Mol. Cryst. Liq. Cryst., 191, 381 (1990).
115.R. B. Meyer, L. Liebert, L. Strzelecki and P. Kelle, J. Phys. Lett., 36, L69 (1975).
116.N. A. Clark and S. T. Lagerwall, Appl. Phys. Lett., 36, 899 (1980).
117. L. A. Beresnev, V. G. Chigrinov, D. I. Dergachev, E. P. Pozhidaev, J. Funfschilling¨ and
M. Schadt, Liq. Cryst., 5, 1171 (1989).
118.S. Garoff and R. B. Meyer, Phys. Rev. Lett., 38, 848 (1977).
119.G. Andersson, I. Dahl, P. Keller, W. Kuczynski, S. T. Lagerwall, K. Skarp and B. Stebler, Appl. Phys. Lett., 51, 640 (1987).
120.L. A. Beresnev, L. M. Blinov, M. A. Osipov and S. A. Pikin, Mol. Cryst. Liq. Cryst., 158A, 3 (1988).
121.A. Fukuda, Y. Takanishi, T. Isozaki, K. Ishihawa and H. Takezoe, J. Mater. Chem., 4, 997 (1994).
122.P. Keller, L. Liebert and L. Strzelecki, J. Phys. (Paris) Col., C3, 27 (1976).
123.L. A. Beresnev, G. Scherowsky, M. Pfeiffer, W. Haase, C. Escher and R. Wingen, Mol. Mat., 2, 73 (1992).
124.M. Schadt and W. Helfrich, Appl. Phys. Lett., 18, 127 (1971).
125. S. Stegemann and J. Mayer, Proc. 10th Electronic Displays, 29 30.03.1995, Chemnitz, Germany, 1995.
126.G. Warmer, Proc. 10th Electronic Displays, 29 30.03.1995, Chemnitz, Germany, 1995.
127.K. Yoshikawa, Y. Kanazawa, M. Wakitani, T. Shinoda and A. Ohtsuka, F & M, 103, 647 (1995).
128.B. Bahadur, Mol. Cryst. Liq. Cryst., 109, 3 (1984).
129.E. P. Raynes, Phil. Trans. R. Soc. Lond. A, 309, 167 (1983).
130.U. Quotschalla, T. Hanemann, M. C. Bohm¨ and W. Haase, Mol. Cryst. Liq. Cryst., 207, 103 (1989).
131.J. K. Foitzik and W. Haase, Mol. Cryst. Liq. Cryst., 149, 401 (1987).
132.G. H. Heilmeier and L. A. Zannoni, Appl. Phys. Lett., 13, 91 (1968).
133.T. Uchida and M. Wada, Mol. Cryst. Liq. Cryst., 63, 19 (1981).
134.A. V. Ivashenko and V. G. Rumyantsev, Mol. Cryst. Liq. Cryst., 150A, 3 (1987).
135.T. J. Scheffer and J. Nehring, Appl. Phys. Lett., 45, 1021 (1984).
136.E. Kaneko, Liquid Crystal TV Displays, KTK Scientific Publishers, Tokyo, Japan (1987).
137.Display Devices Fall ’93, Supplement to Journal of Electronics Engineering, 8, 8 (1993).
138.L. A. Beresnev, T. H. Darmstadt, unpublished results.
139.U. Efron (Ed.), Spatial Light Modulators: Materials, Devices, and Applications, M. Dekker, New York, 1995.