
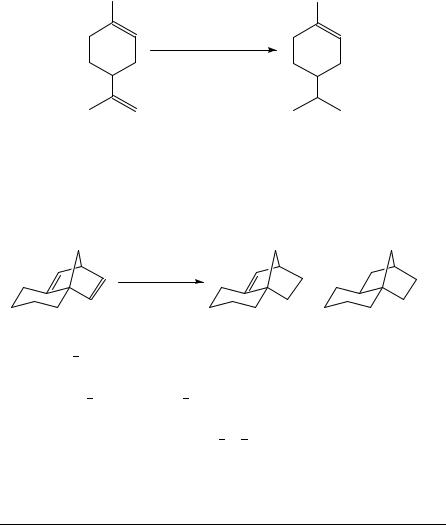
16. Heterogeneous catalytic hydrogenation |
873 |
(21)
NaBH4 , CoCl2 .6H2 O, EtOH, 273 K, 12.5 h |
79% yield |
W-4 Raney Ni, RT, 1 atm H2 |
96% yield |
The hydrogenation of norbornadiene possessing two double bonds of identical reactivity, in contrast, yields the saturated product300. Regioselective semihydrogenation of a similar molecule with two different double bonds, however, can be achieved with a supported Pd catalyst302 (equation 22).
5% Pd-BaSO4 |
+ |
(22) |
|
EtOH, pyridine, |
|||
|
|
||
273 K, 3 h, 1 atm H2 |
|
|
|
|
40 |
1 |
Since differences in the hydrogenation rates of variously substituted monoalkenes were found over Pt zeolite catalysts modified by CVD (see Section IV.A.1) these catalysts were anticipated to exhibit regioselectivities in the hydrogenation of dienes. Selective saturation of the less substituted double bonds of linear and cyclic dienes was indeed achieved over Pt zeolite A and Rh zeolite A catalysts treated with tetraethoxysilane78 or diethoxydiphenylsilane79,80 (Table 13).
Nonconjugated dienes, as mentioned, react less readily than conjugated dienes. The reactivity of 1,5-cyclooctadiene over an Fe Cu SiO2 catalyst, for example, was almost two orders of magnitude lower than that of 1,3-cyclooctadiene286. Isomerization (doublebond migration) of nonconjugated molecules to the more reactive conjugated dienes,
TABLE 13. Regioselective semihydrogenation of dienes over CVD-modified Pt and Rh catalystsa (298 K, hexane, 1 atm H2)78,80
|
|
|
|
|
|
|
|
|
|
|
1,5-Undeca- |
1,5-Trideca- |
1,11-Octadeca- |
Limonene |
8-Cyclohexyl- |
||||||||
|
|
|
|
|
|
|
|
|
|
|
diene |
diene |
|
|
|
|
diene |
|
1,5-octadiene |
||||
|
|
|
|
|
|
|
|
|
|
|
|
|
|
|
|
|
|
|
|
|
|
|
|
Pt |
|
zeolite Ab |
5.0 |
20.8 |
|
|
20.0 |
|
|
59.3 |
2.4 |
|
|
||||||||||
|
|
|
|
|
|||||||||||||||||||
CVD |
|
|
|
Pt(Et)c |
34.8 |
65.2 |
|
|
|
|
n.a. |
87.8 |
58.9 |
|
|
||||||||
|
|
|
|
|
|
|
|||||||||||||||||
CVD |
|
|
|
Pt(Ph)d |
74.8 |
78.7 |
|
|
91.5 |
|
|
n.a. |
47.1 |
|
|
||||||||
|
|
|
|
|
|
|
|||||||||||||||||
CVD |
|
|
|
Rh(Ph)d |
|
|
n.a. |
n.a. |
|
|
64.9 |
|
|
91.5 |
n.a. |
|
|
||||||
|
|
|
|
|
|
|
|||||||||||||||||
a CVD |
|
|
Pt(Et): Pt |
|
|
zeolite A modified |
with (EtO) |
4 |
Si, |
CVD |
|
Pt(Ph): Pt |
|
zeolite |
A modified |
with Ph Si(OEt) |
2 |
, |
|||||
|
|
|
|
|
|
|
|
|
|
|
|
|
|
|
|
|
|
|
|
2 |
|
||
CVD |
|
|
Rh(Ph): Rh |
|
|
zeolite A modified with Ph2Si(OEt)2. |
|
|
|
|
|
|
|
|
|
||||||||
|
|
|
|
|
|
|
|
|
|
|
|
||||||||||||
n.a. denotes not available. |
|
|
|
|
|
|
|
|
|
|
|
|
Values indicate maximum yield of the corresponding monoalkene with internal double bond. b Catalyst quantity D 60 mg.
c Catalyst quantity D 30 mg except for limonene (600 mg). d Catalyst quantity D 250 mg.
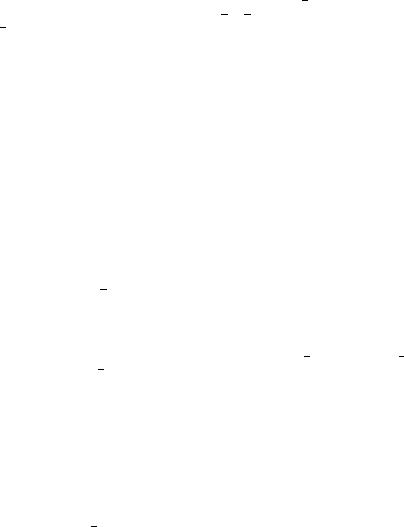
874 |
´ |
Mihaly´ Bartok´ and Arpad´ Molnar´ |
therefore, may be beneficial. Isomeric dienes were indeed detected in the hydrogenation of 1,5-cyclooctadiene over Pd catalysts283,289,303. The hydrogenation of 1,5-cyclooctadiene is usually less selective256,283 than that of 1,3-cyclooctadiene, except on colloidal Pd289. Phenylacetaldehyde, however, was shown to increase the selective formation of cyclooctene (97.4% and 97.6% selectivity over Pd black and Pd CaCO3, respectively)303. The decrease in selectivity observed on a Pd Ag C catalyst relative to monometallic Pd C (87.6% vs 92.4%) is interpreted in terms of a decrease in the rate of the 1,5- to 1,3-cyclooctadiene isomerization caused by silver256.
3. Polyenes
There are very few new data on the hydrogenation of polyenes97,304. The process is practiced in the partial hydrogenation of glycerides.
C. Industrial Applications
Catalytic hydrogenation of the CDC bond plays an important role in large-scale industrial processes. These are called catalytic hydrorefining and used in the purification of cracker streams305,306. Cracking processes produce gasoline and basic raw materials in large quantities for the petrochemical industry. These are ethylene, propylene, butenes and butadiene. Before their further use, however, selective hydrogenation is needed to remove impurities (alkynes and dienes) present. Palladium on alumina is the most selective and widely used catalyst to carry out such purifications. This catalyst transforms diene (and alkyne) contaminants to the corresponding alkenes. The additional advantage of palladium is that it is less sensitive to sulfur poisoning than nickel-based catalysts. Bimetallic Pd catalysts307,308 and Cu silica309 were recently shown to be highly active and selective in such applications.
In C3 hydrorefining the selective conversion of propadiene (and methylacetylene) present in propylene streams is necessary. Liquid-phase or gas-phase processes are practiced.
C4 raw cuts of stream crackers typically contain butanes (4 6%), butenes (40 65%) and 1,3-butadiene (30 50%), as well as some vinylacetylene, 1-butyne, propadiene and methylacetylene. First, acetylenes are selectively hydrogenated and the 1,3-butadiene is extracted resulting in butene cut (or raffinate I). Isobutylene is next removed to produce raffinate II which contains linear butenes and some residual 1,3-butadiene. The latter needs to be removed to achieve maximum butene yields. The methods and catalysts for this process are chosen according to the final use of butenes. The demand for polymer-grade 1-butene, for example, has increased recently. Hydrogenation of residual 1,3-butadiene, therefore, must be carried out under conditions to avoid isomerization of 1-butene to 2-butenes305. Butenes are also of considerable importance in alkylation. The removal of 1,3-butadiene decreases the acid consumption of the alkylation step and thus improves operating conditions and product quality. In this case, however, hydroisomerization of 1-butene to 2-butene is a desirable reaction, since it upgrades the alkylation feed resulting in better alkylates310 312. Oxygenates (alcohols and ethers) have recently gained significance as additives to increase the octane number of reformulated gasoline. Purification of isoamylenes313 and higher alkenes314 by removing 1,3-butadiene under conditions of hydroisomerization has the advantage of forming branched (trisubstituted) alkenes which are transformed to tertiary alkyl methyl ethers.
Gasoline hydrorefining is applied to remove polymerizable by-products (isoprene, cyclopentadiene, styrene and indene) from stream-cracked gasoline to prevent gum formation. Partial hydrogenation of dienes to monoalkenes and the hydrogenation of
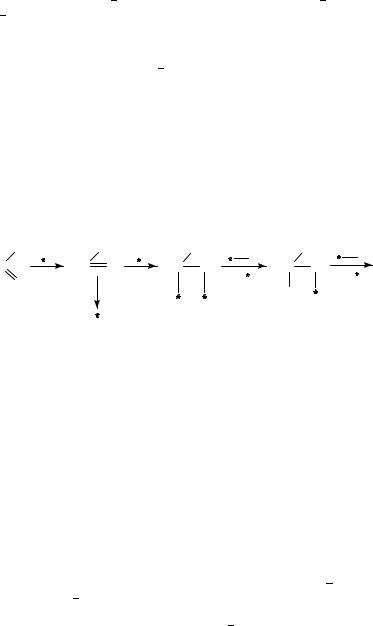
16. Heterogeneous catalytic hydrogenation |
875 |
the alkene double bond in arylalkenes without the saturation of the aromatic ring are achieved by Pd, Ni or Ni W sulfides at elevated temperature (313 373 K) and pressure (25 30 atm)305.
V. HYDROGENATION OF C=O BONDS
Catalytic hydrogenation of carbon oxygen double bonds to the corresponding alcohols proceeds readily in the presence of various metal catalysts, usually under ambient conditions. Two reviews were published1,315 in 1987 and 1991, respectively, since our monograph appeared in 19852. In the latter book this field is summarized in detail with reference to earlier monographs. The present review shortly summarizes the results published recently and discusses the basic conclusions arrived at on the hydrogenation of oxo compounds.
A. Hydrogenation of Aldehydes
Aldehydes are readily hydrogenated to primary alcohols under low pressure conditions (equation 23).
H |
H |
H |
|
H |
|
RC |
RC O |
+ |
H |
+ |
H |
RC O |
RC |
O |
RCH2 OH |
||
(Ar) O |
|
|
−2 |
|
−2 |
|
|
H |
|
(23) |
|
|
|
|
|
Pt, Rh, Ru and Ni catalysts are often used, and Cu has also found application, but Pd is much less active in the hydrogenation of aliphatic aldehydes.
The main conclusions of earlier observations in the field of hydrogenation of aldehydes are as follows:
the hydrogenolysis of the product alcohols formed in the hydrogenation of aliphatic aldehydes leading to alkanes is usually not a major side-reaction,
in the hydrogenation of aromatic aldehydes, however, the hydrogenolysis may become significant, mainly in the presence of Pd under acidic conditions and also on other catalysts,
Raney Ni and copper chromite are selective in forming benzylic alcohols from these compounds,
hydrogenolysis can be avoided by carrying out the hydrogenation under mild conditions and in the presence of small amounts of organic or inorganic bases,
CO formed through the decarbonylation of aldehydes may poison noble metal catalysts, the deactivated catalysts can be activated by the effect of air followed by hydrogen
treatment,
hydrogenation of glucose to sorbitol over Ni is an important example of industrial aldehyde hydrogenation.
Recent research activities resulted in the following additional information.
Most data deal with the hydrogenation of aliphatic aldehydes316 321, mainly that of butyraldehydes318 321, to the corresponding primary alcohols, and the industrial application of this process. In the majority of cases Ni-based catalysts are used, but new results were disclosed using Cu-containing catalysts321 324. The results concerning the active sites of Cu catalysts322 are especially important. Both Cu0 and copper ions were shown to participate in the hydrogenation reaction. Catalyst supports play an important role in forming and stabilizing these active sites.
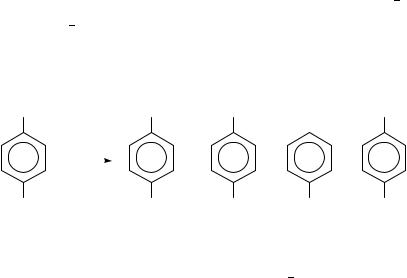
876 |
´ |
Mihaly´ Bartok´ and Arpad´ Molnar´ |
The kinetics of the hydrogenation of propionaldehyde was investigated on different transition metals325. The catalytic activity of the metals studied changes in the sequence Pt > Ir > Rh > Ni > Cu > Co.
Kinetic measurements and adsorption studies325 allowed one to determine the mechanism of the hydrogenation.
A detailed investigation was carried out to study the deactivation of a Ru alumina industrial catalyst326 used in the hydrogenation of glucose. This and numerous other patent applications327 332 testify to the importance of industrial applications of the hydrogenation of aliphatic aldehydes.
Of the aromatic aldehydes the hydrogenation of para-substituted benzaldehydes was studied on supported Pd, Pt and Rh catalysts prepared with different types of TiO2 (equation 24)333,334.
X |
|
X |
X |
|
X |
|
Pd − TiO2 |
+ |
|
+ |
+ |
|
|
|
|||
|
solvent, |
|
|||
|
|
|
|
|
|
|
423 K, |
|
|
|
(24) |
|
10 bar H2 |
|
|
|
|
|
|
|
|
|
|
CHO |
|
CH2 OH |
Me |
Me |
CH2 OEt |
X = COOH |
|
>90% |
max.55% |
min. |
min. |
Me |
|
max.64% |
max.77% |
max.6% |
99% |
Cl |
|
0% |
max.30% |
max.62% |
100% |
Most experimental data are reported on the use of Pd TiO2 catalysts in the hydrogenation. As equation 24 shows, product distribution is considerably affected by the para substituent. The formation of benzyl alcohols is favorable on nonacidic supports while acidic supports promote hydrogenolysis. Hydrogenolysis can also be avoided under strongly acidic conditions in the presence of ethanol. In this case, the product benzyl alcohol readily undergoes dehydration to form benzyl ethyl ether.
Transfer hydrogenation of aldehydes with 2-propanol without the formation of sideproducts is described on a silica-supported Zr catalyst335.
B. Hydrogenation of Ketones
1. Reactivity and mechanistic studies
Selective synthesis of secondary alcohols through the hydrogenation of ketones has long been one of the important reactions of preparative organic chemistry. Numerous reviews were published. An earlier review2 summarizes the following important conclusions:
The majority of group VIII metals and Cu-containing catalysts can be used. Pt, Ni and Cu catalysts are applied most frequently. Pd exhibits negligible activity in the hydrogenation of aliphatic ketones. It can be used, however, in the hydrogenation of phenyl ketones, since the reactant is able to adsorb on the catalyst surface through the aromatic ring. The rate of hydrogenation of ketones is generally lower than that of the corresponding aldehydes.
The hydrogenation of the carbonyl group can, in principle, proceed in two ways (Scheme 9): by the addition of adsorbed hydrogen to the CDO bond (ketonic mechanism), or by the addition of adsorbed hydrogen to the CDC bond of the enol form (enolic mechanism). Deuteration appears to be a good method to distinguish the two mechanisms: the ketonic mechanism would give rise to a C(1) D bond, whereas the enolic mechanism
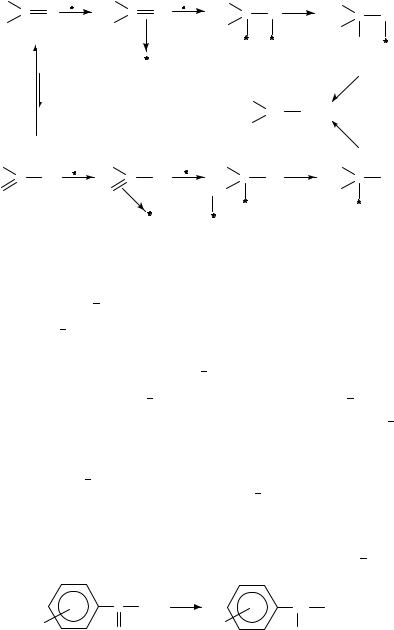
|
|
16. Heterogeneous catalytic hydrogenation |
|
877 |
||||
R |
|
R |
|
R |
H |
R |
|
|
C |
O |
C |
O |
C |
C |
O |
||
O |
||||||||
RCH2 |
|
RCH2 |
|
RCH2 |
|
RCH |
|
|
|
|
|
|
|
|
2 |
|
|
|
|
|
|
|
|
H |
|
|
|
|
|
|
|
(38) |
|
(39) |
|
|
|
|
|
R |
H |
|
||
|
|
|
|
|
|
|||
|
|
|
|
|
CH |
OH |
|
|
|
|
|
|
RCH2 |
|
|
|
|
|
|
|
|
|
|
H |
|
|
R |
|
R |
|
R |
H |
R |
|
|
C |
OH |
C |
OH |
C |
C |
OH |
||
OH |
||||||||
RCH |
|
RCH |
|
RCH |
|
RCH2 |
|
|
|
|
|
|
|
|
(40) |
|
SCHEME 9
leads to a product with C(1) D and C(2) D bonds. It has been found that the ketonic mechanism predominates at lower temperatures, while the enolic mechanism does so at higher temperatures (423 523 K)2.
When cyclic ketones undergo deuterium addition on a fused iron catalyst in the temperature range of 323 483 K, the deuteriogenation proceeds via both the ketonic and enolic mechanisms336.
Numerous mechanistic investigations have been carried out (see an earlier summary2 and new publications, e.g. References 325, 337 339). The reaction of 38 or 39 is regarded as the RDS (Scheme 9). Of the two half-hydrogenated states (39 and 40), 39, in principle, is the more stable, since the oxygen metal bond is stronger than the carbon metal bond.
In recent years special attention has been paid to the study of support effects. The role of the SMSI was mainly examined concerning its effect on the hydrogenation rate337,340 347. The main conclusions of these investigations can be summarized as follows:
TiO2-supported noble metals exhibit high activity in the hydrogenation of molecules containing carbonyl bonds,
the sites at the metal support interface are responsible for this high activity, new sites of high activity appear to reside at the oxide metal adlineation,
the effects of titania on these reactions are attributed to an interaction between CDO bonds and Ti3C ions located at the perimeter of titania islands.
Table 14 gives characteristic data demonstrating the role of SMSI342.
Numerous publications have appeared recently disclosing observations on the hydrogenation of aryl or diaryl ketones to yield aromatic carbinols (equation 25)39,338,343 345,348.
|
M |
|
|
|
(25) |
|
C R |
|
CH |
R |
|
X |
(Ar) H2 |
X |
|
(Ar) |
|
O |
OH |
|
|
||
|
|
|
|
The hydrogenolysis of the carbinol C O bond and the perhydrogenation of the aryl groups may be side-reactions. In most cases, hydrogenolysis is undesirable, but selective hydrogenolysis349 may be the aim of certain synthetic processes. Practical
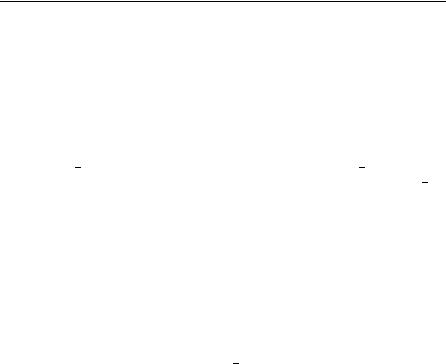
878 |
´ |
Mihaly´ Bartok´ and Arpad´ Molnar´ |
TABLE 14. Hydrogenation of acetone to isopropyl alcohol (i-PrA) over Pt (303 K, Ptotal D 0.1 MPa, H2/acetone D 3.06)342
Catalyst |
Activity |
Turnover frequency |
Eact |
||
|
(10 6 |
mol i-PrA s 1 gPt 1) |
(102 s 1) |
(kcal mol 1) |
|
Pt powder |
0.041 |
0.74 |
16.6 |
||
5.0% Pt |
|
SiO2 |
19.7 |
1.24 |
16.3 |
|
|||||
0.7% Pt |
|
SiO2 |
15.7 |
0.52 |
15.3 |
|
|||||
2.1% Pt |
|
-Al2O3 |
35.7 |
2.39 |
18.6 |
|
|||||
1.9% Pt |
|
TiO2 (LTR) |
106 |
2.75 |
14.1 |
|
|||||
1.9% Pt |
|
TiO2 (HTR) |
238 |
565 |
16.3 |
|
|||||
66% Pt powder C TiO2 |
0.36 |
0.66 |
17.8 |
||
(physical mixture) |
|
|
|
applications350 354 were disclosed in new patents. Pt345, Pd39,350 352, Co348 and Ni338,343,344 catalysts were applied and disclosed in new patents. Pt345, Pd39,350 352,
Co348 and Ni338 catalysts were applied in different ways (various supports, additives, mainly bases, bimetallic catalysts, solvents). In most cases, carbinol selectivities over 90% can be obtained in the liquid phase, at elevated hydrogen pressure, with 100% or nearly 100% conversions.
2. Stereochemistry
Alicyclic ketones, in general, are the appropriate compounds to study the stereochemistry of addition reactions and, in particular, the stereochemistry of hydrogenation. The stereochemical characteristics of the hydrogenation of the carbonyl group have been studied for 70 years. Numerous observations have been made allowing to formulate certain rules, which, however, are suitable for predicting the stereochemistry of hydrogenation only in certain cases. According to the Auwers Skita355,356 rule, reduction with a Pt catalyst in acidic medium leads to a product mixture rich in the cis isomer, whereas reduction with a Raney Ni catalyst under neutral or alkaline conditions yields predominantly the trans isomer. Later, Skita and then others2 established that the isomer ratio is affected not only by the pH of the medium, but also by the rate of hydrogenation (fast and slow hydrogenations favoring the formation of the cis and the trans isomer, respectively), the temperature, the pressure and the catalyst activity.
Barton357 studied steroid ketones and modified the empirical rule. Catalytic hydrogenation of both hindered and nonhindered ketones in strongly acidic medium (fast hydrogenation) results in an axial OH group, while in neutral medium (slow hydrogenation) an equatorial OH group is formed from nonhindered ketones, and an axial OH group from hindered ketones.
One of the numerous schemes published in the literature with respect to the stereochemistry of hydrogenation reaction is given in Scheme 10.
In spite of the large number of investigations, the characteristic conclusion with respect to the stereochemistry of catalytic hydrogenation of cyclic ketones is that it depends on many factors, namely on the structure of the substrate, the nature of the catalyst and the reaction conditions (solvent, reaction temperature, hydrogen pressure, additives).
An earlier review2 gives detailed information about the stereochemistry of the hydrogenation of the CDO group. Some recent investigations358,359 allowed one to demonstrate the decisive role of the structure of the substrate in determining the stereochemistry. Characteristic results shown in equation 26 were achieved by studying alkylcyclohexanones and their corresponding O-containing358 and N-containing359 heterocyclic analogues.
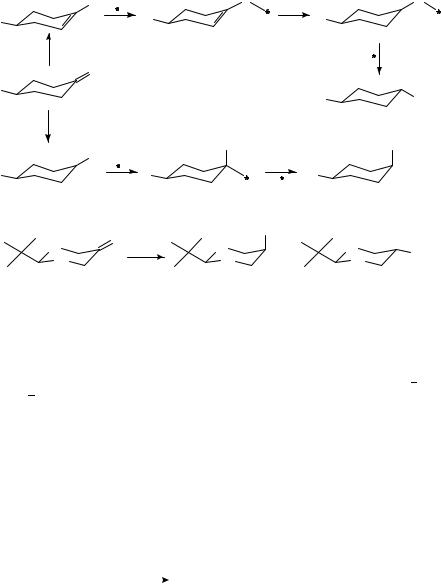
|
|
|
16. Heterogeneous catalytic hydrogenation |
|
879 |
|||
|
|
|
− |
O |
|
|
|
O |
|
|
|
O |
|
|
2H |
|
|
R |
|
|
R |
|
|
R |
|
|
|
|
|
|
|
|
|
|
|
|
|
basic |
|
|
|
|
− |
H |
|
|
medium |
|
|
|
|
||
|
|
|
O |
|
|
|
|
|
R |
|
|
|
|
|
R |
|
OH |
|
|
|
|
|
|
|
||
|
|
acidic |
|
|
|
|
|
|
|
|
medium |
|
|
|
|
|
|
|
|
|
OH |
OH |
|
|
|
OH |
|
|
|
|
|
|
|
|
|
R |
|
+ |
R |
|
H |
R |
|
|
|
|
− |
|
|
||||
|
|
|
|
|
||||
|
|
|
|
|
|
|
|
|
|
|
|
|
SCHEME 10 |
|
|
|
|
|
|
|
O |
|
OH |
|
|
|
|
|
X1 |
X1 |
|
|
X1 |
|
|
|
|
M, H2 |
|
+ |
OH |
|||
|
|
X2 |
|
X2 |
|
X2 |
||
1 |
2 |
= CH2 |
|
32−77% |
|
|
23−68% |
(26) |
|
|
|
|
|||||
X |
= X |
|
70−96% |
|
|
4−30% |
|
|
X1 = O, X2 = CH2 |
|
|
|
|
||||
X1 = X2 = O |
|
90−100% |
|
|
0−10% |
|
M = Co, Ni, Ru, Rh, Pd, Pt, Ir
A high cis selectivity is observed in the hydrogenation of oxaand dioxacyclohexanones. A similar tendency also predominates when the corresponding N-containing analogues are reduced. These results were explained in terms of intramolecular nO CO and nN CO interactions.
During the hydrogenation of methylcyclohexane-1,4-dione on Pt and Pd catalysts the less hindered 4-carbonyl group is selectively hydrogenolyzed360.
3. Industrial applications
The study of the hydrogenation of D-fructose is of both practical importance and theoretical significance since it allows one to investigate stereochemical regularities (equation 27).
|
|
CH2 OH |
|
|
|
CH2 OH |
|
|
|
CH2 OH |
|
|||||||||||||||
|
|
|
|
|
|
|
|
|
HO |
|
|
|
|
|
|
|
|
|
|
|
|
|
|
|
OH |
|
|
|
C |
|
|
O |
|
|
CH |
|
|
|
HC |
|
|
|
|||||||||||
|
|
|
|
|
|
|||||||||||||||||||||
|
|
|
|
|
|
|
|
|
||||||||||||||||||
|
|
|
|
|
|
|
||||||||||||||||||||
HO |
|
|
|
|
|
|
|
|
HO |
|
|
|
|
|
|
|
HO |
|
|
|
|
|
|
|
|
|
CH |
|
CH |
|
|
CH |
|
|
|||||||||||||||||||
|
|
|
|
|
|
|
|
|||||||||||||||||||
|
|
|
|
|
|
|
|
H2 |
|
|
|
|
|
|
+ |
|
|
|
|
|
|
|
|
|
|
|
|
HC |
|
|
|
OH |
|
|
HC |
|
OH |
|
HC |
|
|
OH |
(27) |
||||||||||
|
|
|
|
|
|
|
|
|
|
|
||||||||||||||||
|
|
|
|
|
|
OH |
|
|
|
|
|
|
|
OH |
|
|
|
|
|
|
|
|
|
OH |
|
|
|
HC |
|
|
|
|
|
HC |
|
|
|
HC |
|
|
|||||||||||||
|
|
|
|
|
|
|
|
|
|
|||||||||||||||||
|
|
|
|
|
|
|
|
|
|
|
|
|
|
|
|
|
|
|
|
|
||||||
CH2 OH |
|
|
CH2 OH |
|
CH2 OH |
|
||||||||||||||||||||
D-fructose |
|
D-mannitol |
|
D-sorbitol |
|
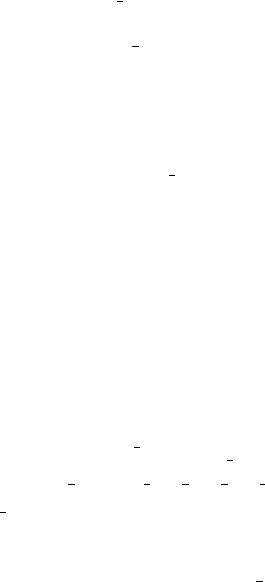
880 |
´ |
Mihaly´ Bartok´ and Arpad´ Molnar´ |
The aim of the investigations is to perform the stereoselective hydrogenation of D-fructose to D-mannitol which is widely used as a sweetening agent and finds also different applications in the food industry. D-Sorbitol, the other isomer, is an important starting material in the preparation of Vitamin C and can easily be prepared from D- glucose. Detailed studies were carried out362 364 for the stereoselective preparation of D-mannitol. Based on the information of an earlier patent361 many different Cu catalysts were prepared and tested. With the best catalysts after suitable modification and under appropriate reaction conditions, selectivities of 85 95% at high conversions can nowadays be obtained.
C. Hydrogenation of Unsaturated Carbonyl Compounds
The hydrogenation of unsaturated carbonyl compounds has recently become a research field studied in great detail. The reason is the practical importance of the selective hydrogenation of two different functional groups of these easily accessible compounds. Of the possible products, saturated oxo compounds and unsaturated alcohols are useful synthons in preparative organic chemistry. The increased interest in this field is verified by the appearance of comprehensive works in recent years1,365 367.
From a thermodynamic point of view, the hydrogenation of the CDC bond is more favorable than that of the CDO group. Since there are numerous other important processes to synthesize saturated aldehydes and ketones, the main objective of recent research efforts is to increase the selectivity of hydrogenation of unsaturated oxo compounds into unsaturated alcohols. The results summarized below clearly indicate the significant success achieved in the selective hydrogenation of unsaturated aldehydes. The hydrogenation of unsaturated ketones, in turn, cannot be accomplished yet with similar selectivities.
1.Hydrogenation of unsaturated aldehydes
a. Reactivity and selectivity. The hydrogenation of ˛,ˇ-unsaturated aldehydes was mostly studied. The reaction can be carried out according to Scheme 11. The products of hydrogenation are the saturated aldehyde and the ˛,ˇ-unsaturated alcohol being formed through competitive reactions as well as the saturated alcohol from consecutive hydrogenation of the intermediate compounds.
The hydrogenation of ˛,ˇ-unsaturated aldehydes to saturated aldehydes is readily achieved over most platinum metal catalysts under mild conditions. The selective synthesis of unsaturated alcohols is, however, much more difficult to perform and several attempts have been made to develop a suitable catalytic system.
The characteristic results are given in Tables 15 19 arranged according to the substrates investigated. The corresponding reactions are given in equations 28 31 and Scheme 12.
According to the data listed in the tables, most of the investigations were carried out
on Ru370,375,377,379,382,383,386,390,392 403 and Pt372 375,378 381,387 389,404 416 catalysts.
Numerous studies were also carried out with Co375,384,404,417, Ni368,375,385,418,419, Rh371,376,377,379,391,420 422, and Cu369,371. Investigations were also performed on Pd377,416,423, Os376, Ir375,376, Ag424,425 and Re426. The fundamental aim is to explore the effect of the catalysts, their modification and the different experimental conditions on the rate of hydrogenation, i.e. to establish the activity and selectivity of the catalysts.
In order to achieve this purpose, investigations were carried out to study the following: the effect of the preparation and pretreatment of catalysts372,373,376,384 389,391,395,399,
407,409,412,414,417,419,421 ,
the effect of modification of catalysts by bases379,382,383,397,398,400,426 ,
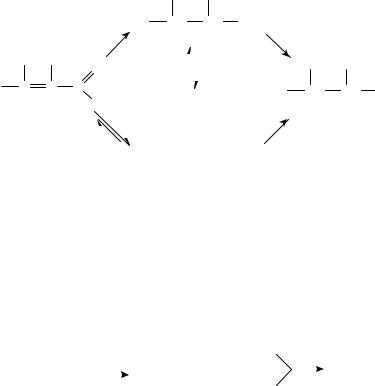
16. Heterogeneous catalytic hydrogenation |
881 |
R2 R3
R1 CH CH CHO
|
|
|
|
H2 |
|
|
|
|
|
|
|
|
|
|
|
R2 |
R3 |
O |
|
|
|
|
|
|
|
|
|
|
|
|
|
|
|
|
|
|
|
||||
|
|
|
|
|
|
|
|
|
|
|
|
||
R1 |
C |
C |
C |
|
|
|
|
|
|
|
|
||
|
|
|
|
|
|
|
|
||||||
|
|
|
|
H |
|
|
|
|
|
|
|
|
|
|
|
|
|
H2 |
R2 |
|
R3 |
H2 |
|||||
|
|
|
|
R1 |
|
|
|
|
|
|
|
|
CH2 OH |
R1 = R2 = R3 = H |
C |
|
|
C |
|
||||||||
|
|
|
|||||||||||
|
|
||||||||||||
|
|
|
|||||||||||
acrolein |
|
|
|
|
|
|
|
|
|||||
R1 = Me, R2 = R3 = H |
crotonaldehyde |
|
|
|
|
|
|
|
|
||||
R1 |
= R2 |
= Me, R3 = H |
3-Me-crotonaldehyde |
|
|
|
|
|
|||||
R1 |
= R3 |
= Me, R2 = H |
2-Me-crotonaldehyde |
|
|
|
|
|
|||||
R1 |
= R2 |
= H, R3 = Me |
methacrolein |
|
|
|
|
|
|
|
|
||
R1 |
= Ph, R2 = R3 = H |
cinnamaldehyde |
|
|
|
|
|
|
|
|
|||
|
|
|
|
|
|
|
SCHEME 11 |
H2
R2 R3
R1 CH CH CH2 OH
TABLE 15. Characteristic data on the hydrogenation of acrolein
|
|
|
|
|
|
|
|
|
|
|
|
|
|
|
|
|
|
|
|
CH3 CH2 CHO |
|
|
(A) |
|
|
|||
|
|
|
|
|
|
|
|
|
|
|
|
|
|
|
|
|
|
|
H2 |
+ |
|
|
|
H2 |
CH3 CH2 CH2 OH (28) |
|||
|
|
|
|
|
|
CH2 |
|
CHCHO |
|
|
(C) |
|||||||||||||||||
|
|
|
|
|
|
|
|
CH2 |
|
CHCH2 OH |
|
|
|
(B) |
||||||||||||||
|
|
|
|
|
|
|
|
|
||||||||||||||||||||
|
|
|
|
|
|
|
|
|
|
|
|
|
|
|
|
|
|
|
|
|
|
|
|
|||||
|
|
|
|
|
|
|
|
|
|
|
|
|
|
|
|
|
|
|
|
|
|
|
|
|||||
|
|
|
|
|
|
|
|
|
|
|
|
|
|
|
|
|
|
|
|
|
|
|
||||||
|
|
|
|
|
|
|
|
|
|
|
|
Catalyst |
Temp. |
Conversion |
|
|
Selectivity (%) |
Reference |
||||||||||
|
|
|
|
|
|
|
|
|
|
|
|
|
|
|
|
|
|
|
(K) |
(%) |
|
|
|
|
|
|
||
|
|
|
|
|
|
|
|
|
|
|
|
|
|
|
|
|
|
|
|
A |
B |
C |
|
|||||
|
|
|
|
|
|
|
|
|
|
|
|
|
|
|
|
|
|
|
|
|
|
|
|
|
||||
|
|
|
|
|
|
|
|
|
|
|
|
|
|
|
|
|
|
|
|
|
|
|
|
|
|
|||
Ni |
|
|
|
glass |
373 |
|
|
|
|
|
|
major |
368 |
|||||||||||||||
|
|
|
|
|
|
|
|
|
||||||||||||||||||||
Cu |
|
|
Al2O3 |
423 |
67 |
75 |
4 |
7 |
369 |
|||||||||||||||||||
|
|
|||||||||||||||||||||||||||
Cu |
|
|
|
Al2O3 C thiophene |
423 |
17 |
68 |
|
|
29 |
369 |
|||||||||||||||||
|
|
|
|
|
||||||||||||||||||||||||
Ru |
|
|
Al2O3 |
363 |
|
low |
24 |
|
|
45 |
370 |
|||||||||||||||||
|
|
|
|
|
||||||||||||||||||||||||
Ru |
|
|
Sn |
|
|
|
|
|
|
Al2O3 |
363 |
|
low |
35 |
6 |
53 |
370 |
|||||||||||
|
|
|
|
|
|
|
||||||||||||||||||||||
Ru |
|
|
Fe |
|
|
|
|
|
Al2O3 |
363 |
|
low |
43 |
|
|
57 |
370 |
|||||||||||
|
|
|
|
|
|
|
|
|
||||||||||||||||||||
Ru |
|
|
Ge |
|
|
Al2O3 |
363 |
|
low |
71 |
9 |
17 |
370 |
|||||||||||||||
|
|
|
|
|
||||||||||||||||||||||||
Ru |
|
|
Zn |
|
Al2O3 |
363 |
|
low |
76 |
4 |
7 |
370 |
||||||||||||||||
|
|
|
|
|||||||||||||||||||||||||
Rh |
|
|
|
Sn |
|
|
|
|
|
|
SiO2 |
353 |
|
|
80 |
|
|
18 |
371 |
|||||||||
|
|
|
|
|
|
|
|
|
|
|
|
|
||||||||||||||||
Pt |
|
|
Nb2O5 (LTR) |
373 |
|
|
58 |
24 |
18 |
372 |
||||||||||||||||||
|
|
|
|
|||||||||||||||||||||||||
Pt |
|
|
Nb2O5 (HTR) |
373 |
|
|
30 |
0 |
70 |
372 |
||||||||||||||||||
|
|
|
|
|||||||||||||||||||||||||
Pt |
|
|
SiO2 |
353 |
|
low |
93 |
2 |
2 |
373 |
||||||||||||||||||
|
|
|
||||||||||||||||||||||||||
Pt |
|
|
Sn |
|
|
|
SiO2 |
348 |
15 |
70 |
10 |
20 |
374 |
|||||||||||||||
|
|
|
|
|
||||||||||||||||||||||||
Pt |
|
|
Fe |
|
|
|
SiO2 |
353 |
|
low |
85 |
7 |
7 |
373 |
||||||||||||||
|
|
|
|
|
|
|||||||||||||||||||||||
Co, Ni, Ru, Ir or Pt on |
|
|
|
|
|
|
|
|
major |
375 |
||||||||||||||||||
Al2O3, SiO2 or ZrO2 |
|
|
|
|
|
|
|
|
|
|
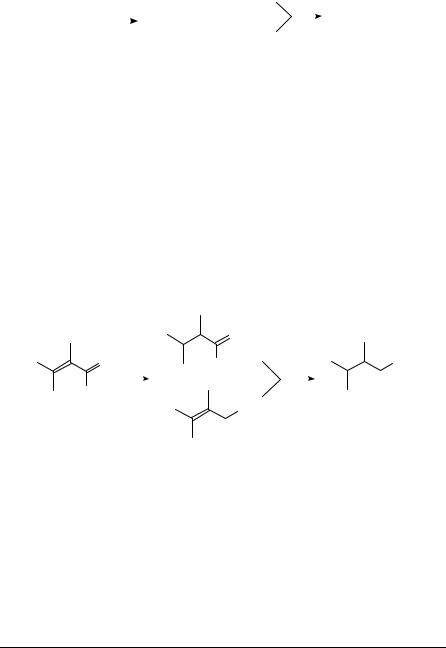
882 |
|
|
|
|
|
|
|
|
|
|
|
|
|
|
|
|
|
´ |
|
|
|
|
|
|
|
|
|
|
|
||||||
|
|
|
|
|
|
|
|
|
|
|
|
|
Mihaly´ Bartok´ and Arpad´ Molnar´ |
|
|
|
|
|
|
|
|||||||||||||||
TABLE 16. |
Characteristic data on the hydrogenation of crotonaldehyde |
|
|
|
|
||||||||||||||||||||||||||||||
|
|
|
|
|
|
|
|
|
|
|
|
|
|
|
|
|
|
|
|
CH3 CH2 CH2 CHO |
(A) |
|
|
|
|
|
|
|
|||||||
|
CH3 CH |
|
|
|
H2 |
+ |
|
|
|
|
|
H2 |
|
|
CH3 CH2 CH2 CH2 OH (29) |
||||||||||||||||||||
|
|
|
CHCHO |
|
|
(C) |
|
|
|||||||||||||||||||||||||||
|
|
|
|
|
|
|
|
|
|
|
|||||||||||||||||||||||||
|
|
|
|
|
|
|
|||||||||||||||||||||||||||||
|
|
|
|
|
|
|
|
|
|
|
|
|
|
|
|
|
|
|
|
CH3 CH |
|
CHCH2 OH |
|
|
|
(B) |
|
|
|||||||
|
|
|
|
|
|
|
|
|
|
|
|
|
|
|
|
|
|
|
|
|
|
|
|
|
|
||||||||||
|
|
|
|
|
|
|
|
|
|
|
|
|
|
|
|
|
|
|
|
|
|
|
|
|
|
|
|
|
|
|
|
||||
|
|
|
|
|
|
|
|
|
|
|
|
Catalyst |
|
Temp. |
Conversion |
|
|
Selectivity (%) |
|
|
|
Reference |
|||||||||||||
|
|
|
|
|
|
|
|
|
|
|
|
|
|
|
|
|
|
|
|
(K) |
(%) |
|
|
|
|
|
|
|
|
|
|
|
|||
|
|
|
|
|
|
|
|
|
|
|
|
|
|
|
|
|
|
|
|
|
A |
B |
C |
|
|
||||||||||
|
|
|
|
|
|
|
|
|
|
|
|
|
|
|
|
|
|
|
|
|
|
|
|
|
|
|
|
||||||||
|
|
|
|
|
|
|
|
|
|
|
|
|
|
|
|
|
|
|
|
|
|
|
|
|
|
|
|
|
|||||||
Cu |
|
|
Al2O3 |
|
423 |
100 |
1 |
|
98 |
1 |
|
|
369 |
||||||||||||||||||||||
|
|
|
|
|
|
||||||||||||||||||||||||||||||
Cu |
|
|
Al2O3 C thiophene |
423 |
|
|
26 |
59 |
|
3 |
29 |
|
|
369 |
|||||||||||||||||||||
|
|
|
|
|
|
|
|||||||||||||||||||||||||||||
Ru |
|
|
TiO2 (LTR) |
373 |
|
|
79 |
51 |
|
30 |
min. |
|
376 |
||||||||||||||||||||||
|
|
|
|
|
|
||||||||||||||||||||||||||||||
Ru |
|
|
TiO2 (HTR) |
373 |
|
|
40 |
43 |
|
38 |
13 |
|
|
376 |
|||||||||||||||||||||
|
|
|
|
|
|
|
|||||||||||||||||||||||||||||
Rh |
|
|
TiO2 (LTR) |
373 |
100 |
71 |
|
17 |
|
|
|
376 |
|||||||||||||||||||||||
|
|
|
|
|
|
||||||||||||||||||||||||||||||
Rh |
|
|
TiO2 (HTR) |
373 |
|
|
41 |
76 |
|
15 |
min. |
|
376 |
||||||||||||||||||||||
|
|
|
|
|
|
||||||||||||||||||||||||||||||
Rh |
|
|
SiO2 |
|
|
|
|
|
|
|
99 |
100 |
|
|
|
|
|
|
|
377 |
|||||||||||||||
|
|
|
|
|
|
|
|
|
|
|
|
|
|
|
|
||||||||||||||||||||
Rh |
|
|
Sn |
|
|
|
SiO2 |
|
|
|
|
|
|
|
51 |
65 |
|
7 |
28 |
|
|
377 |
|||||||||||||
|
|
|
|
|
|
|
|
|
|
|
|
|
|
|
|||||||||||||||||||||
Ir |
|
|
|
TiO2 (LTR) |
373 |
|
|
73 |
18 |
|
44 |
15 |
|
|
376 |
||||||||||||||||||||
|
|
|
|
|
|
|
|||||||||||||||||||||||||||||
Ir |
|
|
|
TiO2 (HTR) |
373 |
|
|
29 |
17 |
|
28 |
48 |
|
|
376 |
||||||||||||||||||||
|
|
|
|
|
|
|
|||||||||||||||||||||||||||||
Pt |
|
|
SiO2 |
|
353 |
<10 |
98 |
|
2 |
|
|
|
378 |
||||||||||||||||||||||
|
|
|
|
|
|
|
|||||||||||||||||||||||||||||
Pt |
|
|
Ni |
|
|
|
|
SiO2 |
|
353 |
<10 |
92 |
|
6 |
2 |
|
|
378 |
|||||||||||||||||
|
|
|
|
|
|
|
|
|
|||||||||||||||||||||||||||
Pt |
|
|
Ga |
|
SiO2 |
|
353 |
<10 |
37 |
|
6 |
56 |
|
|
378 |
||||||||||||||||||||
|
|
|
|
|
|
|
|||||||||||||||||||||||||||||
Pt |
|
|
Sn |
|
SiO2 |
|
353 |
<10 |
63 |
|
6 |
31 |
|
|
378 |
||||||||||||||||||||
|
|
|
|
|
|
|
|||||||||||||||||||||||||||||
Pt |
|
|
SiO2 |
|
353 |
|
low |
50 |
|
34 |
13 |
|
|
373 |
|||||||||||||||||||||
|
|
|
|
|
|
|
|||||||||||||||||||||||||||||
Pt |
|
|
Fe |
|
|
SiO2 |
|
353 |
|
low |
50 |
|
26 |
28 |
|
|
373 |
||||||||||||||||||
|
|
|
|
|
|
|
|
||||||||||||||||||||||||||||
Pt |
|
|
Sn |
|
SiO2 |
|
353 |
|
low |
50 |
|
19 |
30 |
|
|
373 |
|||||||||||||||||||
|
|
|
|
|
|
|
|
||||||||||||||||||||||||||||
TABLE 17. |
Characteristic data on the hydrogenation of other unsaturated aldehydesa |
|
|
||||||||||||||||||||||||||||||||
|
|
|
|
|
|
|
|
|
|
|
|
|
|
|
|
|
|
|
|
|
|
|
|
O (A) |
|
|
|
|
|
|
|
|
|
|
|
|
|
|
|
|
|
|
|
|
|
|
|
|
|
|
|
|
O |
|
H2 |
|
|
H |
|
|
H2 |
|
|
|
|
|
|
OH |
|||
|
|
|
|
|
|
|
|
|
|
|
|
|
|
|
|
|
|
|
|
|
|
|
|
|
|
|
|
|
|
||||||
|
|
|
|
|
|
|
|
|
|
|
|
|
|
|
|
|
|
|
+ |
|
|
|
|
|
|
|
|
|
(30) |
||||||
|
|
|
|
|
|
|
|
|
|
|
|
|
|
|
|
|
H |
|
|
|
|
|
|
|
|
|
|
|
|
|
|
|
|
(B) |
|
|
|
|
|
|
|
|
|
|
|
|
|
|
|
|
|
|
|
|
|
|
|
|
|
|
|
|
|
|
|
|
|
|
|
|
|
|
|
|
|
|
|
|
|
|
|
|
|
|
|
|
|
|
|
|
|
|
|
|
|
OH |
|
|
|
|
|
|
|
|
|
|
|
|
|
|
|
|
|
|
|
|
|
|
|
|
|
|
|
|
|
|
|
|
|
|
|
(C) |
|
|
|
|
|
|
|
||||
|
|
|
|
|
|
|
|
|
|
|
|
|
|
|
|
|
|
|
|
|
|
|
|
|
|||||||||||
|
|
|
|
|
|
|
|
|
|
|
|
Catalyst |
|
|
Substrate |
|
|
Temp. |
Conversion |
|
|
Selectivity (%) |
Reference |
||||||||||||
|
|
|
|
|
|
|
|
|
|
|
|
|
|
|
|
|
|
|
|
|
|
|
|
(K) |
(%) |
|
|
|
|
|
|
|
|||
|
|
|
|
|
|
|
|
|
|
|
|
|
|
|
|
|
|
|
|
|
|
|
|
|
|
A |
B |
C |
|
||||||
|
|
|
|
|
|
|
|
|
|
|
|
|
|
|
|
|
|
|
|
|
|
|
|
|
|
|
|
|
|
|
|
||||
|
|
|
|
|
|
|
|
|
|
|
|
|
|
|
|||||||||||||||||||||
Cu |
|
Al2O3 |
|
2-Me-crotonaldehyde |
423 |
100 |
|
|
|
min. |
96 |
|
|
369 |
|||||||||||||||||||||
|
|
|
|
|
|
|
|||||||||||||||||||||||||||||
Cu |
|
|
Al2O3 C thiophene |
2-Me-crotonaldehyde |
423 |
45 |
|
43 |
38 |
|
15 |
369 |
|||||||||||||||||||||||
|
|
|
|
||||||||||||||||||||||||||||||||
Rh |
|
|
NaY |
|
3-Me-crotonaldehyde |
373 |
25 |
|
84 |
10 |
|
6 |
379 |
||||||||||||||||||||||
|
|
|
|
|
|||||||||||||||||||||||||||||||
Rh |
|
|
KY |
|
3-Me-crotonaldehyde |
373 |
25 |
|
80 |
10 |
|
10 |
379 |
||||||||||||||||||||||
|
|
|
|
|
|||||||||||||||||||||||||||||||
Pt |
|
|
NaY |
|
3-Me-crotonaldehyde |
343 |
25 |
|
48 |
10 |
|
42 |
379 |
||||||||||||||||||||||
|
|
|
|
|
|||||||||||||||||||||||||||||||
Pt |
|
|
KY |
|
|
|
|
|
|
3-Me-crotonaldehyde |
343 |
25 |
|
42 |
2 |
|
56 |
379 |
|||||||||||||||||
|
|
|
|
|
|
|
|
|
|
||||||||||||||||||||||||||
Pt(110) |
|
|
|
|
|
|
3-Me-crotonaldehyde |
353 |
10 |
|
35 |
30 |
|
25 |
380 |
||||||||||||||||||||
Pt(111) |
|
|
|
|
|
|
3-Me-crotonaldehyde |
358 |
10 |
|
8 |
20 |
|
70 |
381 |
||||||||||||||||||||
Pt |
|
|
SiO2 |
|
3-Me-crotonaldehyde |
353 |
|
low |
17 |
55 |
|
21 |
373 |
||||||||||||||||||||||
|
|
|
|
|
|||||||||||||||||||||||||||||||
Pt |
|
|
Fe |
|
|
SiO2 |
|
3-Me-crotonaldehyde |
353 |
|
low |
5 |
14 |
|
80 |
373 |
|||||||||||||||||||
|
|
|
|
|
|
||||||||||||||||||||||||||||||
Pt |
|
|
Sn |
|
SiO2 |
|
3-Me-crotonaldehyde |
353 |
|
low |
8 |
15 |
|
78 |
373 |
||||||||||||||||||||
|
|
|
|
|
|
||||||||||||||||||||||||||||||
Pt |
|
|
Sn |
|
SiO2 |
|
Methacrolein |
|
|
353 |
|
low |
61 |
11 |
|
26 |
373 |
||||||||||||||||||
|
|
|
|
|
|
|
|
||||||||||||||||||||||||||||
Ru |
|
|
SiO2 |
|
3-Me-crotonaldehyde |
313 |
2.1 |
100 |
|
|
|
382 |
|||||||||||||||||||||||
|
|
|
|
|
|
||||||||||||||||||||||||||||||
Ru |
|
|
KC |
|
|
SiO2 |
3-Me-crotonaldehyde |
313 |
2.6 |
27 |
3 |
|
70 |
383 |
|||||||||||||||||||||
|
|
|
|
|
a A: saturated aldehyde, B: saturated alcohol, C: unsaturated alcohol.