
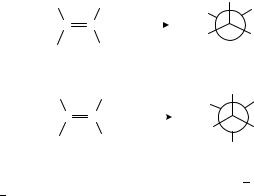
16. Heterogeneous catalytic hydrogenation |
853 |
recently proposed120,122,127 that H adds to the surface ethylidyne to form ethylidene (8). Two ethylidene species then react with gas-phase ethylene to yield ethane and regenerate ethylidyne. Ethyl radicals have recently been detected by EPR in conjunction with spin trapping on supported Pd catalysts130.
The above interpretation is also consistent with ethylene self-hydrogenation. This occurs when transition metal surfaces are exposed to ethylene in the absence of hydrogen and requires the dissociative adsorption of ethylene to form ethane and hydrogen-deficient surface species67,112. In recent years, single crystal surfaces with different crystallographic orientation have been identified to promote this process. Although self-hydrogenation of ethylene is not always observed98,100,128, it does occur on Pt(111)99,127 and Ni(111)131 at higher pressure. Self-hydrogenation of higher alkenes was also detected67,101,132,133. It is always noted, however, that alkane formation is greatly promoted by the presence of preadsorbed hydrogen99,101,132,134.
A partially dehydrogenated hydrocarbon overlayer on metal surfaces under hydrogenation conditions is considered to be responsible for the structure insensitivity of alkene hydrogenation135,136. This means that turnover rates, i.e. the number of molecules reacting (or forming) per unit time per surface metal atom, are the same irrespective of support, metal loading or dispersion. In recent years, this phenomenon was observed in the hydrogenation on different metals of ethylene120,137, propylene138, cyclohexene139 and styrene140,141. Another possible explanation of structure insensitivity is surface restructuring142. Structure insensitivity may also indicate that neither the dissociation of dihydrogen nor the adsorption of alkene requires multiple atoms but they take place on a single metal atom. The active sites, therefore, are not multinuclear in character and H addition occurs via the interconversions of various - and -bonded intermediates as transient ligands of the same metal atom143.
3. Stereochemistry
The basic principle of the stereochemistry of the hydrogenation of the CDC bond is syn (cis) addition. Established already in the early 1930s, it means that both hydrogen atoms add from the same face of the double bond. One of the highest selectivities was observed in the hydrogenation of isomeric 2,3-diphenyl-2-butenes144: the meso compound is formed from the cis isomer in 98% yield (equation 8), whereas the trans isomer gives the racemic compound in 99% yield (equation 9).
Ph |
|
Ph |
|
|
H |
|||
|
|
Me |
|
Ph |
||||
|
|
|
|
|
|
|
||
|
|
|
Pd, H2 |
|
|
|
||
C |
C |
|
|
|
(8) |
|||
|
A cOH |
|
Ph |
|||||
|
|
|
|
|
Me |
|||
Me |
|
Me |
|
|||||
|
|
|
H |
|||||
|
|
|
|
|
|
|
||
|
|
|
|
|
meso-2,3-diphenylbutane |
|||
Ph |
|
Me |
|
|
H |
|||
|
|
Ph |
|
H |
||||
|
|
|
|
Pd, H2 |
|
|
||
C |
C |
|
|
|
|
(9) |
||
|
|
A cOH |
|
Me |
||||
|
|
|
|
|
||||
|
|
|
|
|
|
Ph |
Me Ph
Me
racemic-2,3-diphenylbutane
The cis stereochemistry is consistent with the Horiuti Polanyi mechanism with the Langmuir Hinshelwood pathway. The stepwise addition of the two hydrogen atoms from
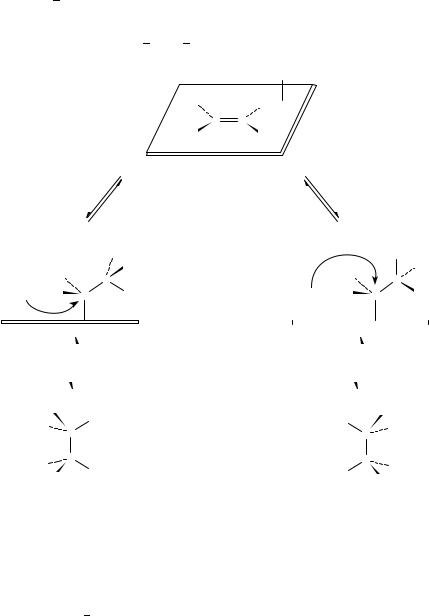
854 |
´ |
Mihaly´ Bartok´ and Arpad´ Molnar´ |
the metal surface to the bottom face of adsorbed alkene via the half-hydrogenated intermediate ensures syn stereochemistry. It is important to emphasize that rotation about the C C single bond does not affect the stereochemical outcome of addition. A new study demonstrated, however, that addition from the top side with identical stereochemistry is also possible90. The addition of hydrogen to cyclohexene on Cu(100) was shown to occur by the Eley Rideal mechanism88,89 (hydrogen from the gas phase adds to the adsorbed alkene). When a prochiral alkene is hydrogenated, the alkanes formed by bottom-face and top-face additions are mirror images of each other (Scheme 1. Group R1 is more highly ranked according to the Cahn Ingold Prelog system than R2).
|
|
|
|
|
|
|
H |
|
|
|
||
|
|
|
|
|
|
R1 |
R2 |
|
|
|
||
|
|
|
|
H |
C |
C |
|
|
|
|||
|
|
|
|
|
|
R2 |
R1 |
|
|
|
||
|
|
bottom-face |
|
|
|
|
|
|
top-face |
|
|
|
|
|
|
|
|
|
|
|
addition |
|
|
||
|
|
addition |
|
|
|
|
|
|
|
|
||
|
|
|
|
|
|
|
|
|
|
|
||
|
|
|
|
R2 |
|
|
|
|
|
|
H |
|
|
|
R1 |
|
R1 |
|
|
|
|
R1 |
|
R2 |
|
|
|
C |
|
|
|
|
|
|
|
C |
||
|
|
R2 |
|
|
|
|
|
|
R2 |
|
||
H |
C |
H |
|
|
H |
C |
R1 |
|||||
|
|
|
|
|
|
|
|
|
|
|
|
|
|
|
|
|
|
|
|
|
|
|
|
|
|
reductive |
|
|
|
reductive |
elimination |
|
|
|
elimination |
|
|
|
|
|
|
|
|
|
|
R1 |
H |
H |
R1 |
|
R2 |
R2 |
|||
C |
||||
|
C |
|
||
R1 |
C |
C |
R1 |
|
H |
||||
R2 |
H |
R2 |
||
|
||||
(S, S)-alkane |
(R, R)-alkane |
SCHEME 1
A further possibility to interpret cis addition is the so-called cis-concerted mechanism74,145. It assumes that the addition of the two hydrogen atoms takes place in a single step in a concerted fashion on a single 3M site possessing three coordinative unsaturations. The transfer of the two hydrogens to the double bond through a concerted process, where the interaction with the catalyst removes the symmetry restrictions imposed by the Woodward Hoffman rules, leads directly to alkane formation.
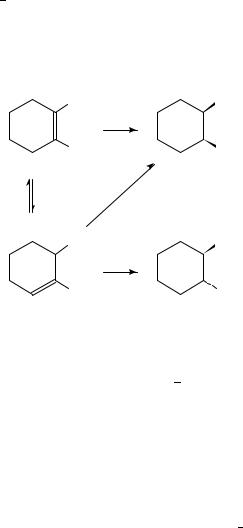
16. Heterogeneous catalytic hydrogenation |
855 |
The transformation of substituted cycloalkenes provided further useful data on the stereochemistry. Most studies were performed in the 1960s. Further systematic studies were carried out and comprehensive interpretations were given by Augustine in the 1980s.
When 1,2-dimethylcyclohexene undergoes hydrogenation over Pt and Pd it always forms cis-1,2-dimethylcyclohexane as the dominant product expected from the syn addition of hydrogen146 148. Varying amounts of the trans isomer, however, are always formed. Detailed studies revealed that instead of anti (trans) addition, the trans isomer is formed through 1,6-dimethylcyclohexene, the product of double bond migration (Scheme 2). The latter can yield both cis and trans saturated products via syn addition. Iridium and osmium were shown to be the most selective metals forming the cis compound with almost 99% selectivity148.
Me |
Me |
|
H2 |
Me |
Me |
|
H2 |
Me |
Me |
|
H2 |
Me |
Me |
SCHEME 2
The effect of hydrogen pressure on the stereochemistry of addition is in accordance with this observation. It was established62,67,149 that under conditions of high hydrogen availability (small catalyst quantity, high pressure and rapid agitation) the product-determining step is the adsorption of the alkene (step 2 in the Horiuti Polanyi mechanism, equation 4). Desorption is negligible under these conditions and product stereochemistry is determined by the difference in steric constraints exerted by the two faces of the double bond with the catalyst surface. Under conditions of low hydrogen availability the product-determining step is the hydrogenation of the half-hydrogenated state (equation 6), since both the alkene adsorption and the formation of the half-hydrogenated state (equations 4 and 5, respectively) are reversible. Additional factors determining product distributions are the mode of adsorption affected by steric effects and the type of adsorbed intermediates formed on different metals.
In the transformation of 1,2-dimethylcyclohexene the fraction of the cis isomer was observed to increase with increasing hydrogen pressure. This is a clear indication that the hydrogen partial pressure affects step 3 (equation 5) in the Horiuti Polanyi mechanism by shifting the equilibrium to the formation of the half-hydrogenated state, therefore suppressing isomerization and increasing selectivity.
The effect of different metals on the stereochemistry was studied149,150,151 in the hydrogenation of 4-tert-butylmethylenecyclohexane (9). Whereas Pt, Rh, Ir and Ru yield predominantly the cis isomer, trans-1-tert-butyl-4-methylcyclohexane is the main product on Pd. The stereochemical behavior of 9 was very similar to that found for the hydrogenation of the isomeric 4-tert-butyl-1-methylcyclohexene (10) (increasing cis selectivity
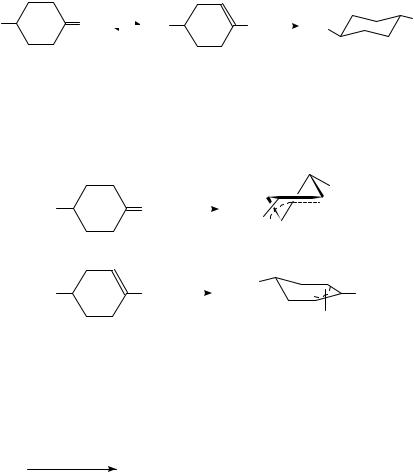
856 |
´ |
Mihaly´ Bartok´ and Arpad´ Molnar´ |
with increasing hydrogen pressure). Since Pd exhibits the highest tendency to catalyze isomerization among platinum metals, it was always suggested that 9 first undergoes isomerization to 10, which is then hydrogenated (equation 10).
t-Bu |
CH2 |
Pd |
t-Bu |
Me |
H2 |
t-Bu |
Me |
|
|
major product (10) |
|||||
|
(9) |
|
(10) |
|
|
|
|
The amount of the cis |
isomer formed in the hydrogenation of 10 at various hydrogen |
pressures, however, is always considerably lower150 than that formed in the hydrogenation of 9. All these observations can be interpreted by invoking -allyl-adsorbed species (11 and 12) instead of 1,2- -diadsorbed species as surface intermediates in the hydrogenation over Pd (equations 11 and 12)150. Of the two species, 12 formed from 10 (equation 12) is expected to result in a higher trans ratio.
|
|
|
|
|
t-Bu |
t-Bu |
CH2 |
Pd |
|
(11) |
|
|
|||||
|
|
|
|||
|
|
|
|
||
|
|
|
CH2 |
|
|
|
|
|
|
Pd |
|
(9) |
|
t-Bu |
Me |
Pd |
|
||
|
(10) |
|
(11)
t-Bu
Me (12)
Pd
(12)
Interesting changes take place in the stereochemistry of the hydrogenation of 9 over Raney Ni. The trans compound is always the main product over a fresh catalyst, whereas high cis ratios are observed over aged samples (equation 13)152. This was interpreted to prove that the adsorption of the substrate becomes the product-controlling step over the aged catalysts.
Me
Raney Ni
9
EtOH, RT, 1 atm H2
t-BuMe + t-Bu
(13)
fresh catalyst |
78 |
22 |
aged for 30 days |
15 |
85 |
reflux in EtOH for 2 h |
6 |
94 |
poisoned with CO |
2 |
98 |
1,3-Dimethylcyclohexene and 1,5-dimethylcyclohexene yield an identical mixture of cis- and trans-1,3-dimethylcyclohexane on Pd153 (Table 7). On Pt and Rh, in contrast, the two isomeric dimethylcyclohexenes lead to products of different composition (Table 7). The adsorption modes of 1,3-dimethylcyclohexene is illustrated by the -adsorbed species 13 and 14 leading to cis- and trans-1,3-dimethylcyclohexane, respectively (equations 14 and 15)155. A comparison of 13 and 14 clearly indicates that the high cis/trans ratio
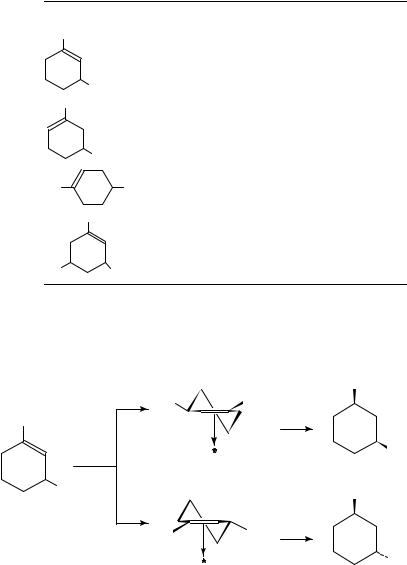
16. Heterogeneous catalytic hydrogenation |
857 |
TABLE 7. Product distribution (cis/trans ratio) in the hydrogenation of substituted cyclohexenes (ambient temperature and pressure, ethanol)
Compound |
|
Pt |
Rh C |
Pd C |
Reference |
Me |
|
|
|
|
|
|
|
72/28 |
74/26 |
82/18 |
153 |
Me |
|
|
|
|
|
Me |
|
|
|
|
|
|
|
59/41 |
49/51 |
81/19 |
153 |
Me |
|
|
|
|
|
Me |
Me |
57/43 |
57/43 |
27/73 |
153 |
61/39 |
57/43 |
28/72a |
154 |
||
Me |
|
|
|
|
|
|
|
60/40b |
54/46 |
73/27 |
155 |
Me Me
a Pd.
b Pt C.
observed on Pt and Rh is a result of the steric interaction in 14 of the allylic methyl group with the catalyst surface.
|
|
|
Me |
Me |
Me |
|
|
Me |
|
H2 |
(14) |
|
|
|
|
Pt or Rh |
(13) |
|
Me |
|
|
||
|
|
|
|
Me |
|
|
Me |
|
|
|
|
Me |
Me |
H2 |
(15) |
|
|
|
|
|
|
|
Me |
|
(14) |
|
|
The -adsorbed species of 1,5-dimethylcyclohexene are those depicted as 15 and 16. The nearly equal amount of the isomeric products formed on Pt and Rh (Table 7) testifies to the almost equal degree of hindrance of the homoallylic methyl group with the catalyst surface in the alternate adsorption modes.
The identical product composition observed in the transformation of the two alkenes over Pd, however, is strong evidence of a common intermediate. This species is a -allyl
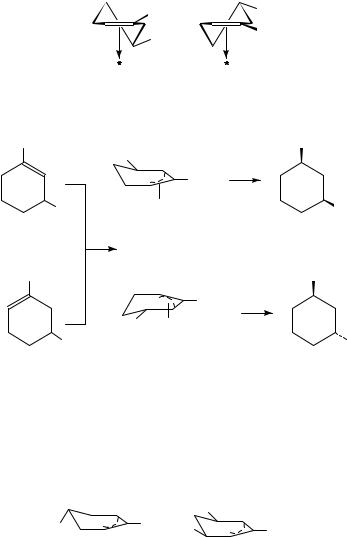
858 |
|
´ |
Mihaly´ Bartok´ and Arpad´ Molnar´ |
||
|
Me |
Me |
|
Me |
Me |
|
|
|
|
(15) |
(16) |
intermediate adsorbed on the catalyst surface primarily in the less hindered cis mode (17) yielding cis-1,3-dimethylcyclohexane (equation 16).
Me |
|
|
Me |
Me |
|
|
|
|
|
Me |
3 H |
|
|
|
|
Me |
Pd |
|
Me |
(17) |
|
||
|
|
|
|
Pd |
|
|
(16) |
−H |
|
|
|
Me |
|
|
Me |
|
|
Me |
3 H |
|
|
|
|
Me |
Pd |
|
|
Me |
|
|
Me |
When 1,4-dimethylcyclohexene is hydrogenated on Pt and Rh, cis-1,4-dimethylcyclo- hexane is formed in slight excess (Table 7). These data, again, are consistent with the involvement of -adsorbed species with an almost equal degree of hindrance in the alternate adsorption modes. The homoallylic substituent, consequently, does not exert any strong influence on stereochemistry. The trans compound, however, is the main product on Pd (Table 7) indicating a favored trans adsorption mode (18).
|
|
|
|
Me |
|
Me |
|
Me |
Me |
|
Me |
|
|
|
|
|
|
|
Pd |
|
|
|
|
|
|
|
Pd |
||
|
|
|
|
||
|
(18) |
|
(19) |
||
Hydrogenation of 1,3,5-trimethylcyclohexene over Pt and Rh gives essentially the same |
|||||
results (nearly the same amount of cis |
product) as obtained in the hydrogenation of 1,4- |
and 1,5-dimethylcyclohexene155 (Table 7). This indicates that in this case the homoallylic methyl group is more influential in determining stereochemistry than the allylic methyl. The favored 19 -allyl species is responsible for the high cis/trans ratio on Pd. The observations summarized above are supported by the results of the transformations of selectively deuterated substrates156.
Further details on the stereochemistry of alkene hydrogenation can be found in earlier books and review papers62,63,67. They treat the effect of polar substituents
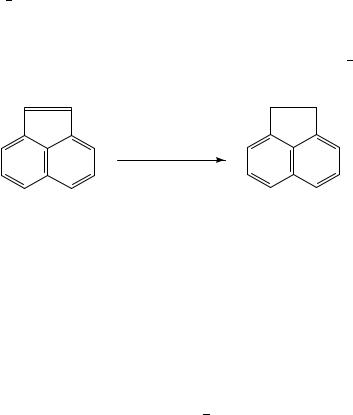
16. Heterogeneous catalytic hydrogenation |
859 |
on stereochemistry, i.e. substrate-directed transformations are also discussed157. Such reactions are, however, outside the scope of the present review.
4. New catalysts for alkene hydrogenation
During the last 15 years many new catalyst preparations were introduced and their catalytic properties were very often tested in the hydrogenation of alkenes. Since heterogeneous catalytic hydrogenation is a surface reaction, the effectiveness of the process can be greatly increased by increasing the number of active sites available. This can be done by applying high surface area support materials and specific catalyst preparation techniques. These highly dispersed catalysts, i.e. catalysts with high active sites density, are expected to exhibit high specific activity. In this subchapter the most important examples of these new catalysts are discussed and their main characteristics in the hydrogenation of alkenes are demonstrated.
a. Metal atoms, clusters and colloidal particles. Free metal atoms, metal clusters and extremely small metal particles of diameters from one to some tens of nanometers exhibit profoundly different characteristics compared to metals in the bulk state. Various methods have been, therefore, developed to produce such metal agglomerates and test their catalytic behavior158 160.
Solvated metal atoms can be dispersed in excess organic solvent at low temperature and used as a source of metal particles for the preparation of both unsupported metal powders and supported metal catalysts158,161. Alternatively, metal vapor is condensed into a cold solution of a stabilizing polymer to form crystallites of the order 2 5 nm in diameters159. Equation 17 illustrates the unique activity of a colloidal Pd catalyst in the partial hydrogenation of acenaphthene.
methylcyclohexane, |
(17) |
298 K, 1 atm H2 |
|
uptake of 1 equiv. H2 |
|
Colloidal Pd |
0.25 h |
Pd black |
5 h |
Reduction methods can also be used to produce polymer-protected colloidal metal powder catalysts160. Ions of noble metals are readily reduced in solution in the presence of soluble protective polymers to form ultrafine metal particles. The protective polymers are physically adsorbed on the surface of the metal particles thereby preventing their aggregation by steric stabilization and dispersing them homogeneously in the liquid phase. Pt162,163, Pd164 and Rh particles165 were covalently immobilized in this way. Such catalysts may exhibit much higher activity in the hydrogenation of alkenes than the corresponding supported catalysts (Table 8). Their activity, however, may be smaller than that of the nonprotected colloidal metal (Table 8).
Polymer-protected colloidal Pt could be deposited onto high-surface-area titania prepared by the sol-gel-aerogel method166. This catalyst exhibited much higher activity in the hydrogenation of trans-stilbene (306 K, isopropyl acetate, 1 atm H2) than catalysts prepared by using commercial supports or Pt titania made by the one-step aerogel technique167.
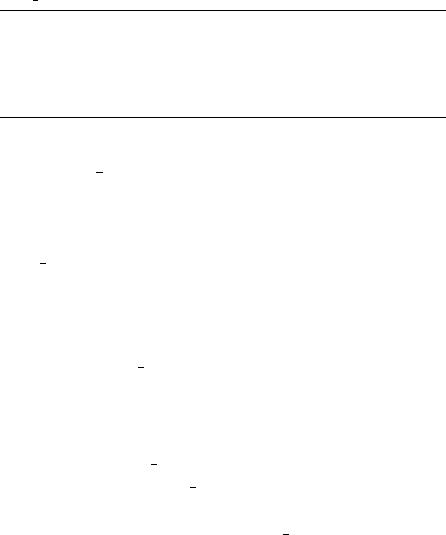
860 |
´ |
Mihaly´ Bartok´ and Arpad´ Molnar´ |
TABLE 8. Catalytic activity of Rh and Pt catalysts in the hydrogenation of alkenes (303 K, 1 atm H2, ethanol water)162,165
|
|
|
|
Initial hydrogenation rate |
|
|
|
||
|
|
(10 3 |
mol H2 molmetal1 |
s 1) |
|
|
|
||
|
ultrafine Rh |
5% Rh |
|
C |
ultrafine Pt |
colloidal Pt |
50% Pt |
|
C |
|
|
|
|||||||
|
particles |
|
|
|
particles |
particles |
|
|
|
|
|
|
|
|
|
|
|
||
Cyclopentene |
230 |
44 |
|
|
n.a.a |
n.a.a |
n.a.a |
||
Cyclohexene |
150 |
7 |
|
|
36 |
240 |
2.1 |
|
|
1-Hexene |
190 |
38 |
|
|
110 |
330 |
3.2 |
|
|
a n.a. denotes not available.
Various forms of nickel metal powders are prepared by the reduction of different nickel compounds with K B alloy168 or low-oxidation-potential metals (Na, Mg or Zn)169. The activity of the latter catalyst in the hydrogenation of alkenes (1-hexene and cyclohexene) is comparable with that of Raney nickel.
Graphimets, a special class of graphite intercalation compounds, contain metals between the layers of graphite either in atomic dispersion or as small clusters since the graphite layers prevent metal aggregation170. In addition, a considerable amount of the metal may be on the graphite surface. Alkene hydrogenation was used to study the characteristics of Pt171 175, Pd176, Ni171,172,177 and Cu172 graphimets. Interlayer metal particles do not participate in alkene hydrogenation since active sites located between the graphite layers are inaccessible for 1-butene174 and cyclohexene173,175 test molecules. As a result, hydrogenation occurs only on surface particles. Metal-graphimets, therefore, can be regarded as traditional supported metal catalysts in alkene hydrogenation175.
b. Amorphous metal alloy powders. When certain metal salts are reduced by appropriate reagents, amorphous metal metalloid alloys in the form of a finely divided black precipitate are formed, which exhibit excellent properties in hydrogenating many functional groups. Catalysts prepared from Ni and Co have been studied most41.
The classical P-2 nickel boride catalyst is prepared by mixing a 95% ethanolic solution of nickel acetate with the ethanolic solution of sodium borohydride14. The resulting alloy powder is very sensitive to the alkene structure (cyclohexene is hydrogenated at a very low rate70) and shows an unusually low tendency for isomerization. With slight modifications of the original procedure Ni B catalysts of different hydrogenation activities can be obtained. These catalyst preparations are usually more active and productive in alkene hydrogenations than Raney nickel178 180. Cobalt borides, in turn, are less active than nickel borides due to the lower activity of cobalt in alkene hydrogenation181.
Catalytic activities were shown to strongly depend on the Ni concentration and the molar ratio of borohydride to nickel179,180,182,183. Ni B powders showed maximum activity when the NaBH4:NiCl2 ratio was 1.5 (the rate of the hydrogenation of cycloheptene was 4.3 ð 10 3 mol g-atom Ni 1 s 1; 303 K, 1 atm H2)184. When the synthesis, in contrast, was performed in the presence of PVP serving as a protective polymer, a threefold excess of NaBH4 was required to achieve maximum activity (191 ð 10 3 mol g-atom Ni 1 s 1)184. The polymer-protected sample proved to be especially effective in the hydrogenation of compounds with isopropenyl group (2-methyl-1-hexene and ˛- methylstyrene).
The activity of three Ni67B33 catalyst samples was compared in the hydrogenation of ethylene185. The parent amorphous powder made by reducing Ni2C ions with KaBH4 in an aqueous solution was heat-treated to produce partially amorphous and crystalline catalysts.
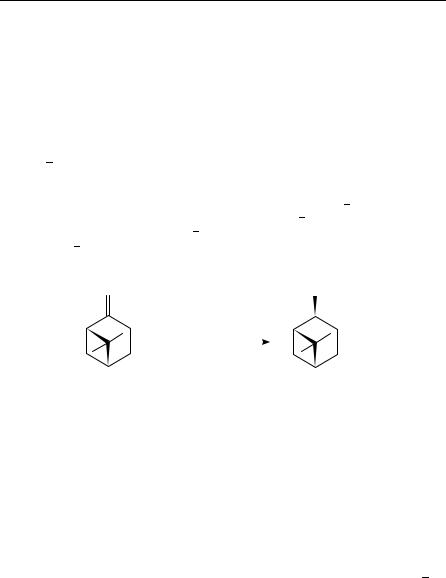
16. Heterogeneous catalytic hydrogenation |
861 |
TABLE 9. Surface and catalytic properties of Ni67B33 alloy catalysts in the hydrogenation of ethylene (423 K, 1 atm, H2/ethylene D 1)
Catalysts |
|
Surface area |
Conversion |
H2 uptake |
Relative turnover |
||
|
|
(m2 g 1) |
(%) |
(10 |
6 |
mol g 1) |
frequency |
|
|
cat |
|
|
|
cat |
|
Amorphous |
|
19 |
50 |
|
|
23 |
1.00 |
Partially amorphous |
|
|
|
|
|
|
|
(heat treatment at 573 |
K) |
15 |
88 |
|
|
41 |
0.99 |
Crystalline |
|
|
|
|
|
|
|
(heat treatment at 773 |
K) |
8 |
24 |
|
|
11 |
1.00 |
|
|
|
|
|
|
|
|
Though the relative rates were very similar, the partially amorphous catalyst exhibited the most rapid hydrogen uptake when expressed in gram of catalyst used (Table 9).
Nickel phosphorous amorphous alloy powders are produced by the reaction of an aqueous solution of NiCl2 and NaH2PO2. A Ni85P15 sample prepared in this way showed stable activity in the hydrogenation of styrene when compared to an amorphous Ni87P13 ribbon prepared by the rapid quenching method186. Amorphous Ni P deposited on silica by electroless plating is superior to polycrystalline Ni silica and a Ni90P10 ribbon in the hydrogenation of styrene (453 573 K, atmospheric pressure, cyclohexane, flow reactor)187. Ni P deposited on various alumina supports showed high activity and selectivity in the hydrogenation of ˛-pinene to cis-pinane188 (equation 18), comparable to those of polycrystalline nickel catalysts189.
0.5 g Ni-P-on-A l2 O3 |
(18) |
|
383−423 K, 27.5 atm H2 , 2 h |
||
|
31−69% conversion
87−95% selectivity
c. Rapidly quenched amorphous metal alloys. In the early 1960s a new technique was developed to produce bior multicomponent amorphous metal alloys, also called metallic glasses. This method, the rapid quenching from the melt190,191, made amorphous alloys easily available in large quantities in the form of thin ribbons or flakes. During the last 15 years numerous studies have been performed to explore the catalytic properties of these unique materials15,16. Due to their low surface area they usually require activation and in many instances they exhibit better activities and selectivities than their crystalline counterparts.
In the liquid-phase hydrogenation of cis-cyclododecene the ratio of isomerization to hydrogen addition was higher on amorphous Pd80Si20 and Pd77Ge23 than on the crystalline alloys, i.e. the amorphous catalysts produced more trans isomer192,193. In the hydrogenation of (C)-apopinene (6,6-dimethyl-1R,5R-bicyclo[3.1.1]hept-2-ene) the two amorphous alloys resulted in exceptionally low isomerization relative to addition194 196. On the basis of these observations a method to characterize palladium catalysts using (C)-apopinene as probe molecule was developed (see Section IV.A.7).
Many amorphous alloys were tested in the hydrogenation of ethylene. It was shown that the thermal pretreatment of a Cu70Zr30 amorphous precursor in hydrogen leads to structural modification and the resulting catalysts exhibit extremely high activities in ethylene hydrogenation197 (Table 10).
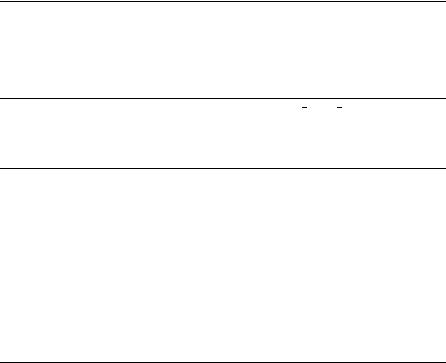
862 |
´ |
Mihaly´ Bartok´ and Arpad´ Molnar´ |
TABLE 10. Catalytic activity of hydrogen-treated (473 K, 16 h) Cu70Zr30 catalysts in the hydrogenation of ethylene (1.6 atm total pressure, ethylene/hydrogen D 1)
Catalysts |
BET surface areaa |
Initial rate (106 mol mcat2 s 1) |
||
|
(m2 g 1) |
358 K |
423 K |
453 K |
Completely amorphous precursor |
0.56 |
3.7 |
29.9 |
34.9 |
Partially amorphous precursor |
0.03 |
117 |
455 |
579 |
Crystalline precursor |
n.a.b |
n.a.b |
18.1 |
21.0 |
Cu powder |
n.a.b |
n.a.b |
11.5 |
15.3 |
a Surface area determined by krypton physisorption calculated by the Brunauer Emmett Teller equation. b n.a. denotes not available.
TABLE 11. Effect of pretreatment of amorphous and crystalline Cu62Zr38 alloys on the surface characteristics and catalytic activity in the hydrogenation of ethylene (473 K)a
Catalysts and |
Cu concentration |
BET surface area |
Initial rate |
||
pretreatments |
(ICu/ICu |
C |
IZr) |
(m2 g 1) |
(105 mol min 1 g 1) |
|
|
|
cat |
cat |
|
A (hydrogen, 473 K, 2 h) |
0.30 |
|
2.12 |
13 |
|
A (1 M HF, 5 min C |
0.95 |
|
7.52 |
410 |
|
hydrogen at 473 K, 2 h) |
|
|
|
|
|
A (1 M HNO3, 5 min C |
0.10 |
|
1.13 |
1 |
|
hydrogen at 473 K, 2 h) |
|
|
|
|
|
A (1 M HNO3, 5 min C |
0.38 |
|
2.85 |
10 |
|
oxygen at 473 K, 1 h C |
|
|
|
|
|
hydrogen at 473 K, 2 h) |
|
|
|
|
|
C (hydrogen, 473 K, 2 h) |
0.41 |
|
2.32 |
2 |
|
C (1 M HF, 5 minChydrogen |
0.89 |
|
4.96 |
8 |
|
at 473 K, 2 h) |
|
|
|
|
|
a A denotes amorphous, C denotes crystalline; ICu and IZr are peak intensities of Cu and Zr, respectively, in the ESCA spectra.
Dissolution of Zr from bimetallic amorphous alloys proved to be a very effective way of activation198,199. A pulverized amorphous Cu62Zr38 alloy treated with hydrogen fluoride (1 M solution, 5 min) gave a Raney-type copper catalyst exhibiting much higher activity in the hydrogenation of ethylene than the catalyst made from the crystalline alloy198 (Table 11). The high activity was attributed to the increase in both the surface area and the surface concentration of copper. Similar conclusions were arrived at when the same HF dissolution was applied for amorphous and crystalline Ni36Zr64 ribbons199.
Ni81P19 and Ni62B38 glassy alloys were studied in the hydrogenation of simple alkenes (ethylene, propylene and butenes) and dienes (1,3-butadiene and isoprene, see Section IV.B.1). Successive treatments of the virgin alloy ribbons with HNO3, oxygen and hydrogen brought about high catalytic activity for both hydrogenation and isomerization of CDC bonds200. Activation of Ni62B38 was also achieved by heat treatment below 623 K resulting in specimens in the precrystallization state201,202 and by the pulverization in a vibratory rod mill203. In all these cases, electronic interactions between nickel, oxygen and the metalloid were invoked to account for favorable changes in hydrogenation activity.
Numerous alloy |
ribbons in |
both amorphous and |
crystalline forms were tested in |
|||||||||||||
the hydrogenation |
of 1-hexene204 |
|
206. Selectivities |
were found to be independent |
||||||||||||
|
||||||||||||||||
on crystallinity. Ni-containing |
alloys (Ni |
|
Zr, Fe |
|
Ni |
|
B, Fe |
|
Ni |
|
P) and Cu |
|
Zr were |
|||
|
|
|
|
|
|